Introduction
Land-use and land-cover changes are major threats to biodiversity conservation that result in forest loss, fragmentation and degradation (Pereira et al., Reference Pereira, Leadley, Proença, Alkemade, Scharlemann and Fernandez2010; Lindenmayer, Reference Lindenmayer, Cunningham, Young and Lindenmayer2012). Regions of high biodiversity that experience continual threats (Myers et al., Reference Myers, Mittermeier, Mittermeier, da Fonseca and Kent2000) need attention as the shifts in land-use and land-cover patterns, and climate change, exacerbate disturbance processes such as wildfires, insect irruptions, invasions of exotic species, droughts and heat-induced tree mortality (Allen et al., Reference Allen, Macalady, Chenchouni, Bachelet, McDowell and Vennetier2010; Veblen et al., Reference Veblen, Holz, Paritsis, Raffaele, Kitzberger and Blackhall2011; Gaertner et al., Reference Gaertner, Biggs, Beest, Hui, Molofsky and Richardson2014; Seidl et al., Reference Seidl, Thom, Kautz, Martín-Benito, Peltoniemi and Vacchiano2017; Miranda et al., Reference Miranda, Lara, Altamirano, Di Bella, González and Julio Camarero2020).
The temperate forests of south-central Chile are a biodiversity hotspot (Myers et al., Reference Myers, Mittermeier, Mittermeier, da Fonseca and Kent2000) because of their high species richness and endemism, and habitat loss and wildlife population declines. The native forests and biodiversity in the coastal region (35°–37° S) of south-central Chile are particularly threatened because of the high human population density and conflicting land-use demands (Armesto et al., Reference Armesto, Rozzi, Smith-Ramirez and Arroyo1998; Echeverria et al., Reference Echeverria, Coomes, Salas, Rey-Benayas, Lara and Newton2006; Alaniz et al., Reference Alaniz, Galleguillos and Perez-Quezada2016; Miranda et al., Reference Miranda, Altamirano, Cayuela, Lara and González2016). Here several narrowly distributed and endemic tree species of the Maulino forests (sensu San Martín & Donoso, Reference San Martín, Donoso, Armesto, Kalin and Villagrán1996) are categorized as Endangered on the IUCN Red List because of intense deforestation and fragmentation (e.g. Nothofagus alessandrii, Gomortega keule, Pitavia punctata; Lara et al., Reference Lara, Donoso, Aravena, Armesto, Kalin and Villagrán1996; Barstow et al., Reference Barstow, Baldwin, Rivers and Echeverría2017; IUCN, 2018). There are few public or private areas protecting these species' rich and endemic ecosystems (Armesto et al., Reference Armesto, Rozzi, Smith-Ramirez and Arroyo1998; Pliscoff & Fuentes-Castillo, Reference Pliscoff and Fuentes-Castillo2011; Bannister et al., Reference Bannister, Vidal, Teneb and Sandoval2012). Only 15% of the total area of N. alessandrii forests lie within protected areas (Santelices et al., Reference Santelices, Drake, Mena, Ordenes and Navarro-Cerrillo2012). During 1981–1991, the N. alessandrii forests declined at an annual rate of 8.15% of their area, resulting in the loss of > 50% of these forests in that period, mainly because of the expansion of industrial Pinus radiata plantations (Bustamante & Castor, Reference Bustamante and Castor1998; Grez et al., Reference Grez, Bustamante, Simonetti, Fahrig, Salinas-Chávez and Middleton1998; Heilmayr et al., Reference Heilmayr, Echeverría, Fuentes and Lambin2016). As of 2008, the remnant 314 ha of N. alessandrii forests were confined to a mosaic of many small (< 2 ha) and scattered fragments surrounded by a matrix of exotic pine plantations (Grez et al., Reference Grez, Bustamante, Simonetti, Fahrig, Salinas-Chávez and Middleton1998; Santelices et al., Reference Santelices, Drake, Mena, Ordenes and Navarro-Cerrillo2012), making them prone to disturbances such as forest fires and invasive species.
Anthropogenic fires and the invasion of exotic species are significant threats to the survival of the Maulino forests. Since 2010 forest fire activity has increased in south-central Chile, particularly in the coastal range of the Maule region (Carvajal & Alaniz, Reference Carvajal, Alaniz, Smith-Ramirez and Squeo2019). Droughts and heatwaves are the main factors associated with increasing numbers of large wildfires and the burning of larger areas (Garreaud et al., Reference Garreaud, Alvarez-Garreton, Barichivich, Boisier, Christie and Galleguillos2017; González et al., Reference González, Gómez-González, Lara, Garreaud and Díaz-Hormazábal2018; Bowman et al., Reference Bowman, Moreira-Muñoz, Kolden, Chávez, Muñoz and Salinas2018). During the 2016–2017 fire season, an extreme and destructive mega-fire (named Las Máquinas) initiated by human action burnt an area of 160,000 ha, including 55% (172 ha) of the last remnants of N. alessandrii forests (Valencia et al., Reference Valencia, Saavedra, Brull and Santelices2018). Pine plantations may favour the spread of large fires (McWethy et al., Reference McWethy, Pauchard, García, Holz, González and Veblen2018; Bowman et al., Reference Bowman, Moreira-Muñoz, Kolden, Chávez, Muñoz and Salinas2018) and promote the invasion of N. alessandrii fragments by massive seedling recruitment of P. radiata, a pyrophytic species (i.e. adapted to fire).
Key drivers of ecosystem change can interact with and reinforce each other, and can shift entire landscapes into undesirable and potentially irreversible states (i.e. landscape traps) in which major functional and ecological attributes are compromised (Lindenmayer et al., Reference Lindenmayer, Hobbs, Likens, Krebs and Banks2011). For instance, post-fire salvage logging in tropical rainforests and the subsequent increase of fire-prone grassland species can lead to an altered fire regime that limits recovery (van Nieuwstadt et al., Reference van Nieuwstadt, Shiel and Kartawinata2001; Cochrane & Laurance, Reference Cochrane and Laurance2008). Similarly, alteration of the stand structure in temperate forests by logging can increase the occurrence and severity of wildfires through modification of fuel types and loads (Odion et al., Reference Odion, Frost, Strittholt, Jiang, Dellasala and Moritz2004; Lindenmayer et al., Reference Lindenmayer, Hunter, Burton and Gibbons2009, Reference Lindenmayer, Hobbs, Likens, Krebs and Banks2011). In north Patagonia, Araucaria araucana forests affected by extreme wildfire events and re-burns after short intervals, attributed to changing climate trends and land-use practices, have favoured a transition to a fire-prone landscape, creating a potential landscape trap, especially when invaded by Pinus species (Cóbar-Carranza et al., Reference Cóbar-Carranza, García, Pauchard and Peña2014; González et al., Reference González, Muñoz, González-Reyes, Christie and Sibold2020).
Forest fires and invasion by exotic species are critical emerging pressures, especially for the coastal range Maulino forests (San Martín & Sepúlveda, Reference San Martín and Sepúlveda2002; Bustamante et al., Reference Bustamante, Serey, Pickett, Bradshaw and Marquet2003; Santelices et al., Reference Santelices, Espinoza, Cabrera, Magni and Cabrera Ariza2018; Carvajal & Alaniz, Reference Carvajal, Alaniz, Smith-Ramirez and Squeo2019; González et al., Reference González, Becerra-Rodas, Lara and Galleguillos2019; Leal, Reference Leal2020). Here we examine the effects of the 2017 Las Máquinas mega-fire on the last remnants of forests harbouring N. alessandrii, a rare, endemic deciduous tree species of the coastal range of central Chile. Our main goals were to: (1) assess the severity of the fire in the N. alessandrii forest fragments affected by the mega-fire, (2) determine the land-cover composition and configuration of the main land uses surrounding the N. alessandrii forest fragments, (3) assess the invasion of P. radiata into N. alessandrii forests, and (4) evaluate the survival and post-fire responses of these threatened forests 2 years after the fire. Finally, considering the critical state of these forests, we recommend restoration and management strategies at the landscape and forest scales to avoid the loss of the last remnants of these threatened N. alessandrii forest ecosystems.
Study area
The study area lies in the coastal range of the Maule region of central Chile. The predominant climate is Mediterranean, characterized by winter rainfall, with dry summers in September–April (Beck et al., Reference Beck, Zimmermann, McVicar, Vergopolan, Berg and Wood2018). Nothofagus alessandrii (family Nothofagaceae) grows in relatively deep soils (> 50 cm) of metamorphic and granitic origin, which are relatively poor, with low phosphorus content, and are highly susceptible to erosion (San Martín et al., Reference San Martín, Santelices, Henríquez and Donoso2013). We studied nine N. alessandrii populations burnt by the 2017 mega-fire. Six of these populations are in the southern burnt area and three in the northern burnt area (Fig. 1).

Fig. 1 (a) Land-use (Table 1) and land-cover classes within a 10-km radius of the centroid of Nothofagus alessandrii forest fragments (three in the north and six in the south) in the study area in the Maule region of central Chile, and the extent of the 2017 anthropogenic Las Máquinas mega-fire. (b) Per cent of the various land-use and land-cover classes surrounding the northern and southern N. alessandrii fragments in the burnt areas. (c) The land-use and land-cover classes surrounding the N. alessandrii fragments in the southern area.
Table 1 Land-use classes in the study area of the Maule region in central Chile estimated using remote-sensing data (Fig. 1).
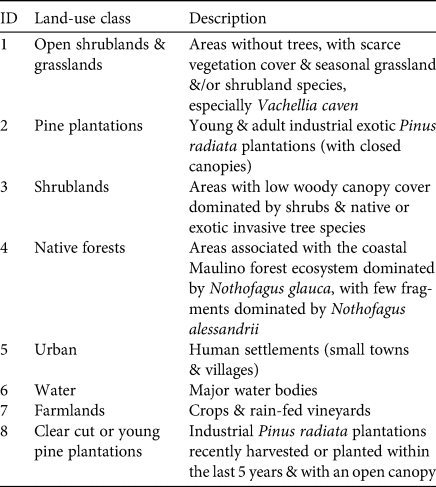
Nothofagus alessandrii is an endemic, broad-leaved deciduous tree species that occurs in small patches on south, south-west or south-east facing aspects at elevations of 160–440 m (Donoso & Lara, Reference Donoso, Lara, Armesto, Kalin and Villagrán1996). This species forms nearly pure or mixed second-growth post-fire forests originated mostly from vegetative reproduction (i.e. suckers arising from the base of a tree). In mixed stands it is associated with Nothofagus glauca and other hygrophyllous (Aextoxicon punctatum, Nothofagus obliqua, Gevuina avellana) and mesophytic (Cryptocarya alba, Peumus boldus) species of the sclerophyllous forests (San Martín et al., Reference San Martín, Figueroa and Ramírez1984; Donoso, Reference Donoso, Veblen, Hill and Hill1996). The present distribution of N. alessandrii forests is restricted and fragmented along 100 km of the coastal range of central Chile within a landscape dominated by extensive industrial forest plantations of P. radiata, and shrublands (San Martín et al., Reference San Martín, Figueroa and Ramírez1984). These forests are thought to be remnants of a much larger population (Baldwin et al., Reference Baldwin, Barstow and Rivers2018). The small forest patches include 15 populations of 1–67 ha, with a total forest cover of 314 ha (Donoso, Reference Donoso, Veblen, Hill and Hill1996; San Martín & Donoso, Reference San Martín, Donoso, Armesto, Kalin and Villagrán1996; San Martín & Sepúlveda, Reference San Martín and Sepúlveda2002; Santelices et al., Reference Santelices, Drake, Mena, Ordenes and Navarro-Cerrillo2012, Reference Santelices, Espinoza, Cabrera, Magni and Cabrera Ariza2018). Currently, N. alessandrii is one of the most threatened tree species in continental Chile and is recognized as a Natural Monument (Hechenleitner et al., Reference Hechenleitner, Gardner, Thomas, Echeverria, Escobar, Brownless and Martínez2005; MMA, 2021) and categorized as Endangered on the IUCN Red List (Barstow et al., Reference Barstow, Baldwin, Rivers and Echeverría2017; Baldwin et al., Reference Baldwin, Barstow and Rivers2018).
Methods
Remote-sensing data and fire severity assessment
We used radar-based and optical information from the Sentinel-1 and Sentinel-2 satellites, respectively, to assess the fire severity of the nine burnt N. alessandrii populations (172 ha). We selected pre-fire images from both satellites (January 2017) and a post-fire image from Sentinel-2 (March 2017).
The radar-based Sentinel-1 image was obtained from Google Earth (Google, Mountain View, USA) and included vertical–horizontal and vertical–vertical polarities and a speckle filter correction (Lee et al., Reference Lee, Jurkevich, Dewaele, Wambacq and Oosterlinck1994). We used the sen2r package (Ranghetti et al., Reference Ranghetti, Boschetti, Nutini and Busetto2020) in R 4.0.1 (R Core Team, 2020) to obtain and process the optical Sentinel-2 images. We applied radiometric and atmospheric corrections to convert the data from the top of the atmospheric radiance to the bottom of the atmospheric reflectance. We selected bands with 10 or 20 m spatial resolution (B2, B3, B4 and B8) corresponding to the visible, near-infrared (NIR) and shortwave-infrared (SWIR) spectra for further post-processing. Using these bands, we estimated the normalized difference vegetation index, green normalized difference vegetation index and soil-adjusted vegetation index as indicators of vegetation vigour and greenness.
We used the difference normalized burn ratio (dNBR) as a fire severity proxy as it integrates pre- and post-fire vegetation status (Fernandez-Manso et al., Reference Fernandez-Manso, Fernández-Manso and Quintano2016; Mallinis et al., Reference Mallinis, Mitsopoulos and Chrysafi2018). Firstly, we used the NIR and SWIR Sentinel-2 bands to assess the pre- and post-fire normalized burn ratio of the vegetation as:

Secondly, we assessed the difference between pre- and post-fire greenness as:

Because of the low variability amongst the intermediate severity classes, we reclassified the five dNBR classes (Key & Benson, Reference Key and Benson2006) into three fire severity classes, supported by ground truthing: (1) high severity (carbonized tree trunks, stems and leaves; dNBR > 0.66), (2) moderate severity (trees did not retain leaves or needles and most of the crown canopy was burnt; 0.27 < dNBR < 0.66), and (3) low severity (trees possess green foliage and most of their structure was not severely affected by the fire; dNBR < 0.27).
Finally, we used a lidar-derived digital terrain model resampled to a resolution of 10 m from which we depicted the topographical position index using SAGA GIS (Conrad et al., Reference Conrad, Bechtel, Bock, Dietrich, Fischer and Gerlitz2015). This index indicates whether, in relation to its neighbours, a pixel corresponds to a flat, concave or convex area. We uploaded all images and derived variables to Google Earth for further cloud-based post-processing.
Land use and land cover surrounding N. alessandrii forests
We selected the nine fire-affected N. alessandrii populations mapped by Corporación Nacional Forestal (Valencia et al., Reference Valencia, Saavedra, Brull and Santelices2018). We then identified the main land use and land cover (Table 1) within a 10-km radius area of influence around the centroid of N. alessandrii forest patches. Because the nine N. alessandrii populations are geographically separate, we analysed patches in the northern and southern areas of the mega-fire. We used all of the derived remote-sensing variables described in the previous section for the analysis, including optical (Sentinel-2 bands and indices), structural (Sentinel-1) and topographical (digital terrain model and topographical position index) information.
We collected 90 training and 30 validation polygons per class (first seven classes of Table 1) using visual interpretation of high-resolution Google Earth images and a 2016 land-cover map (Zhao et al., Reference Zhao, Feng, Yu, Wang, Chen and Bai2016). We then used Google Earth to train and validate a random forest classifier (Breiman, Reference Breiman2001) using the collected samples. We tuned and assessed model performance using the overall accuracy metric obtained from the confusion matrix.
Classifying clear cuts or young pine plantations (identified as class 8 in Table 1) was spectrally complex as these classes are often confused with crops, shrublands and naturally non-vegetated areas. To overcome this, we used the Google Earth integration of the LandTrendr algorithm (Kennedy et al., Reference Kennedy, Yang, Gorelick, Braaten, Cavalcante, Cohen and Healey2018) to segment and assess time since last disturbance using normalized difference vegetation index time series analysis. We analysed annual data for 1990–2016. We assumed that disturbances during 2011–2016 occurring inside the shrubland and grassland classes corresponded to the ‘clear cuts or young pine plantations’ class and reclassified them accordingly. We did not consider fire occurrences during 2011–2016, so there could be small assignment errors to class 8. Finally, we applied a majority filter to improve the classification and to obtain per-class coverages (in ha and per cent, respectively).
Pinus radiata invasions inside N. alessandrii forest fragments before the mega-fire
To develop a pre-fire land-cover map, we selected pre-fire images from Sentinel-1 and Sentinel-2 from 19 January 2017, 1 week before the Las Máquinas mega-fire. Within this map we assessed P. radiata invasion inside the N. alessandrii forest fragments by counting the number of pixels classified as pine (corresponding mainly to dominant pine trees) inside the native forest. Then we depicted the coverage of the invasion in ha. We reclassified shrubland predictions into native forest classes as we know from field experience that these forest stands do not have dominant shrubland species.
Forest composition and structure, and post-fire responses of burnt N. alessandrii forests
We assessed the survival and post-fire responses of a second-growth N. alessandrii forest affected by the Las Máquinas mega-fire. This remnant, corresponding to one of the nine N. alessandrii forest populations, is located in El Desprecio (The Neglected) property owned and protected by a private company dedicated to forest plantations. The mega-fire occurred in summer 2017 and burnt almost 100% of this N. alessandrii population.
In April 2017 (i.e. 2 months after the fire), we set nine 250-m2 circular plots (8.9-m radius) in stands with low, moderate and high dNBR-based severity levels. In these plots we recorded all tree species (native and exotic) and measured the diameter at breast height of all individuals with a diameter at breast height ≥ 5 cm. We classified the survival status of the trees as either dead (stems and canopy burnt without green foliage) or alive (green foliage in the stems and/or crowns, and/or basal vegetative sproutings). In each plot we set 12 circular subplots of 3.14 m2 (1-m radius) to count the number of tree seedlings (0.05–2.00 m in height) of the various species and to assess their regeneration origin (i.e. seeds or basal sprouts of trees). We assessed the survival status of trees and seedling recruitment again 2 years after the fire, in April 2019.
Results
Fire severity, and land use and land cover surrounding N. alessandrii forests
Of the 172 ha of N. alessandrii stands burnt by the 2017 mega-fire (corresponding to 55% of the total area), 15% (25 ha) was burnt by a low-severity fire and 47% (81 ha) and 38% (66 ha) were burnt by moderate- and high-severity fires, respectively. The land-use and land-cover map of the first seven classes (Table 1) had an overall accuracy of 87%. The land-use and land-cover map indicated that 60% of the area surrounding N. alessandrii forests corresponded to P. radiata plantations and clear cuts of this tree species (Fig. 1). Shrublands were the primary native vegetation type surrounding N. alessandrii forests, with 16% cover, and only 8% of the area was classified as native forests (Fig. 1b), with a fragmented spatial pattern.
Pinus radiata invasion inside N. alessandrii forests before the mega-fire
We estimated that P. radiata had invaded and become a dominant species in the forest canopy in 16% (28 ha) of the total area of the nine N. alessandrii populations (172 ha) before the mega-fire occurred in 2017 (Fig. 2). These invasions were mainly along the edges of the fragments close to plantations and clear cuts (Fig. 2b). The range of P. radiata cover in the nine populations was 11–40%. Smaller fragments had a greater cover of pine compared to larger fragments.

Fig. 2 (a) Invasions of pine Pinus radiata into N. alessandrii forest fragments in the southern study area in the Maule region of central Chile, and (b) magnification illustrating the invasion of pine at the edges of N. alessandrii fragments.
Forest composition and structure, and post-fire responses of burnt N. alessandrii forests
The structure of N. alessandrii stands indicates that at the time these fragments were affected by the 2017 mega-fire, they were in an intermediate state of development, with a density of 1,200–3,240 trees/ha and a basal area of 17–74 m2/ha. The diameter distribution of these second-growth patches shows a greater number of trees in the smallest size class (5–15 cm diameter at breast height) representing an evenly aged cohort established after a high-severity fire (Fig. 3a). In addition, N. alessandrii stands feature adult P. radiata (> 50 cm diameter at breast height) trees established in forest clearings in recent decades.

Fig. 3 Tree survival and tree species seedlings or shoots within N. alessandrii forest stands in the study area in the Maule region of central Chile affected by low-, moderate- and high-severity fires. (a) Diameter at breast height (DBH) distribution of tree species (10 cm refers to the class 5–15 cm, etc.) in the N. alessandrii stands showing surviving, dead and total trees in April 2017 (i.e. 2 months after the anthropogenic Las Máquinas mega-fire). (b) Post-fire recruitment of N. alessandrii and associated tree species from seeds and vegetative shoots monitored in April 2017 and 2019. Note the high invasion of Pinus radiata seedlings. Nale, Nothofagus alessandrii; Ngla, Nothofagus glauca; Prad, Pinus radiata; Other: Cryptocarya alba, Aextoxicon punctatum, Persea lingue and Gevuina avellana.
The survival status measured 2 months after the 2017 mega-fire demonstrated high mortality of N. alessandrii trees independent of fire severity (Fig. 3a). Nevertheless, by April 2019 > 80% of the N. alessandrii trees had re-sprouted, demonstrating their ability to survive high-severity fires (Fig. 3b, Plate 1b). Evidence for recurrent and severe fires having shaped these fragments comes from the protuberances formed at the bases of many trees as a result of continuous re-sprouting. Post-fire recruitment of N. alessandrii from seeds was low by 2019 (< 17%) and restricted to the fragments affected by low- and moderate-severity fires. All adult P. radiata affected by moderate severity fires were killed.

Plate 1 (a) Nothofagus alessandrii second-growth forest. (b) N. alessandrii forest burnt with high severity in the private protected area El Desprecio and sprouting from burnt N. alessandrii trees (note in the background, left, an adult pine Pinus radiata). (c) Dense post-fire recruitment of P. radiata from seeds. Photos: (a) M.E. González; (b,c) C. Becerra-Rodas.
During the 2 years after the fire, seedlings of P. radiata colonized the burnt sites at high density from seeds stored in serotinous cones, particularly in fragments burnt with high severity (Plate 1c). By 2019 the number of seedlings of P. radiata was 1,600–15,000 per ha in the burnt sites (Fig. 3b).
Discussion
Since 1750, N. alessandrii forest stands have been degraded and destroyed as a result of fires set by people, and by logging (Donoso & Landaeta, Reference Donoso and Landaeta1983; Donoso & Lara, Reference Donoso, Lara, Armesto, Kalin and Villagrán1996), and since the 1970s this has occurred because of the conversion of the Maulino forests to fast-growing industrial plantations of P. radiata associated with a large-scale subsidy programme from the Chilean state through Decree Law 701 (Donoso & Lara, Reference Donoso, Lara, Armesto, Kalin and Villagrán1996; Lara et al., Reference Lara, Donoso, Aravena, Armesto, Kalin and Villagrán1996; San Martín & Donoso, Reference San Martín, Donoso, Armesto, Kalin and Villagrán1996; Bustamante & Castor, Reference Bustamante and Castor1998; Echeverria et al., Reference Echeverria, Coomes, Salas, Rey-Benayas, Lara and Newton2006; Heilmayr et al., Reference Heilmayr, Echeverría, Fuentes and Lambin2016). Currently, the landscape is dominated by an extensive and homogeneous matrix comprising forest plantations and scattered, small fragments of N. alessandrii. Pinus radiata is categorized as Endangered in its native habitat in California, with a population that is severely fragmented. One of the main threats P. radiata faces is competition from other trees in the absence of periodic fires (Farjon, Reference Farjon2013).
The Las Máquinas mega-fire burnt 160,000 ha, including 55% (172 ha) of the last forests of N. alessandrii, a threatened species protected by law. Extreme weather conditions characterized by heatwaves and strong winds (Bowman et al., Reference Bowman, Moreira-Muñoz, Kolden, Chávez, Muñoz and Salinas2018) resulted in > 85% of these forests being burnt with moderate or high severity (Valencia et al., Reference Valencia, Saavedra, Brull and Santelices2018). However, the ease of propagation and the severity of the fire were probably amplified by the large proportion of monoculture plantations of highly flammable trees surrounding N. alessandrii fragments (> 60% of the land use covered by pine plantations). The presence of adult P. radiata within the fragments of N. alessandrii, especially in the smaller fragments and at their edges, could also have increased the spread and severity of the fire (Veblen et al., Reference Veblen, Holz, Paritsis, Raffaele, Kitzberger and Blackhall2011; Cóbar-Carranza et al., Reference Cóbar-Carranza, García, Pauchard and Peña2014; García et al., Reference García, Engler, Peña, Pollnac and Pauchard2015; Raffaele et al., Reference Raffaele, Nuñez, Eneström and Blackhall2016; Taylor et al., Reference Taylor, Maxwell, McWethy, Pauchard, Nuñez and Whitlock2016).
Stand-replacing fires have shaped N. alessandrii forests over the past centuries (Donoso, Reference Donoso, Veblen, Hill and Hill1996; González et al., Reference González, Becerra-Rodas, Lara and Galleguillos2019, Reference González, Becerra-Rodas, Lara, Little and Martin2022). The 2017 mega-fire that affected these second-growth stands demonstrated the ability of N. alessandrii to cope with and recover after fires. Most trees survived mainly through vegetative reproduction, by re-sprouting from the epicormic buds at the bases of the trunks of the dead trees, which frequently displayed protuberances as evidence of re-sprouting following past fire events. Pinus radiata trees died in this mega-fire event because of their greater flammability (González et al., Reference González, Becerra-Rodas, Lara and Galleguillos2019).
Species of the genus Pinus are highly invasive in the Southern Hemisphere, where they are used extensively in plantations for industrial timber production (Richardson et al., Reference Richardson, Williams and Hobbs1994; Simberloff et al., Reference Simberloff, Nuñez, Ledgard, Pauchard, Richardson and Sarasola2009; Langdon et al., Reference Langdon, Pauchard and Aguayo2010; Cóbar-Carranza et al., Reference Cóbar-Carranza, García, Pauchard and Peña2014; Pauchard et al., Reference Pauchard, Escudero, García, Cruz, Langdon, Cavieres and Esquivel2016; Raffaele et al., Reference Raffaele, Nuñez, Eneström and Blackhall2016). These introduced pines are pioneer species adapted to regenerate after high-severity fires (e.g. from the serotinous cones of P. radiata) or to survive fires and provide seed sources for post-fire regeneration (Williams & Wardle, Reference Williams and Wardle2007; Veblen et al., Reference Veblen, Holz, Paritsis, Raffaele, Kitzberger and Blackhall2011). In the coastal Maule region, fire has been a key disturbance process promoting the invasion of P. radiata into native forest remnants. Fires that affect the matrix dominated by this exotic conifer stimulate the release of large amounts of viable wind-dispersed seeds from the serotinous cones of surrounding plantations, which facilitates their invasion of N. alessandrii and other native forest stands (Williams & Wardle, Reference Williams and Wardle2007). After the 2017 mega-fire the fire-prone pines demonstrated rapid post-fire recruitment within the N. alessandrii forests because of the abundant seeds stored in the serotinous cones of killed pines within these forest fragments (Veblen et al., Reference Veblen, Holz, Paritsis, Raffaele, Kitzberger and Blackhall2011; González et al., Reference González, Becerra-Rodas, Lara and Galleguillos2019, Reference González, Becerra-Rodas, Lara, Little and Martin2022). Pine density was particularly high in forests burnt with high severity. Considering that 85% (147 ha) of the N. alessandrii forests were burnt with moderate or high severity, a potentially dense invasion of exotic pines would be expected, which would reduce the resilience of these forests to fire disturbances.
Since 2010, forest fire activity has increased in south-central Chile. The increase in large wildfires and area burnt, and the extension of the fire seasons, have been associated with increased drought conditions and heatwaves (González et al., Reference González, Gómez-González, Lara, Garreaud and Díaz-Hormazábal2018; Bowman et al., Reference Bowman, Moreira-Muñoz, Kolden, Chávez, Muñoz and Salinas2018). Furthermore, pine plantations have been heavily affected (in terms of area and severity) under the extreme fire conditions facilitated by drying and warming climate trends (Garreaud et al., Reference Garreaud, Alvarez-Garreton, Barichivich, Boisier, Christie and Galleguillos2017; González et al., Reference González, Gómez-González, Lara, Garreaud and Díaz-Hormazábal2018; McWethy et al., Reference McWethy, Pauchard, García, Holz, González and Veblen2018), leading to positive feedback between wildfires and invasions (Taylor et al., Reference Taylor, Maxwell, McWethy, Pauchard, Nuñez and Whitlock2016). Thus, P. radiata invasion after wildfires converts N. alessandrii forests into areas of fire-prone pyrophytic vegetation (a condition also favoured by invasions of exotic shrubs such as Teline monspessulana and Cytisus scoparius; Pauchard et al., Reference Pauchard, García, Langdon, Nuñez, Donoso, González and Lara2014; García et al., Reference García, Engler, Peña, Pollnac and Pauchard2015; Kitzberger et al., Reference Kitzberger, Perry, Paritsis, Gowda, Tepley, Holz and Veblen2016; Raffaele et al., Reference Raffaele, Nuñez, Eneström and Blackhall2016). Major changes in landscape spatial patterns (i.e. massive industrial plantations of P. radiata) and feedback processes (i.e. increased fire activity and invasions of exotic species) are leading N. alessandrii forests into a landscape trap (sensu Lindenmayer et al., Reference Lindenmayer, Hobbs, Likens, Krebs and Banks2011). Most examples of landscape traps involve a modified disturbance regime and forest structure and composition, invasion processes and climate change as key drivers of ecosystem change, which can shift the forested landscape into an altered and irreversible state that is ecologically impaired (Cochrane & Laurance, Reference Cochrane and Laurance2008; Lindenmayer et al., Reference Lindenmayer, Hobbs, Likens, Krebs and Banks2011; Bowman et al., Reference Bowman, Murphy, Neyland, Williamson and Prior2014; Cóbar-Carranza et al., Reference Cóbar-Carranza, García, Pauchard and Peña2014; Fairman et al., Reference Fairman, Nitschke and Bennett2015; Kitzberger et al., Reference Kitzberger, Perry, Paritsis, Gowda, Tepley, Holz and Veblen2016; Tepley et al., Reference Tepley, Thompson, Epstein and Anderson-Teixeira2017; González et al., Reference González, Muñoz, Sibold, Riquelme and Rodriguez2021).
Recognition of the processes that could lead to a landscape trap requires the use of diverse approaches and tools (Lindenmayer et al., Reference Lindenmayer, Hobbs, Likens, Krebs and Banks2011). Remote-sensing technologies are essential to assess invasion processes and the spatial and temporal attributes of fires (i.e. severity and size) at the landscape level (Kattenborn et al., Reference Kattenborn, Lopatin, Förster, Braun and Fassnacht2019; Fassnacht et al., Reference Fassnacht, Schmidt-Riese, Kattenborn and Hernández2021). In addition, the IUCN Red List of Ecosystems (Alaniz et al., Reference Alaniz, Pérez-Quezada, Galleguillos, Vásquez and Keith2019) can inform policy process and identify policy instruments that could improve ecosystem-level outcomes for biodiversity conservation (Keith et al., Reference Keith, Rodríguez, Brooks, Burgman, Barrow, Bland and Spalding2015). The use of complementary and integrated approaches at a national scale would facilitate the study of temporal ecosystem changes and generate landscape-integrated conservation and restoration strategies, including those that are pertinent to N. alessandrii stands. Thus, land-use planning and large-scale restoration are key to changing those landscape spatial characteristics that render areas vulnerable to human and natural disturbances.
Programmes focused on the conservation of N. alessandrii forests at the landscape scale should receive high national priority to prevent the disappearance of these ecosystems. Such programmes will need to design a more diverse, heterogeneous and less flammable landscape through the conversion of 40–50% of industrial forest plantations in each 20,000–50,000 ha watershed to native forests, including N. alessandrii. This should also include the creation of 40–50 m wide buffer zones along streams and headwaters surrounded by exotic forest plantations and/or other productive uses. Converting these areas to native forests would introduce fire breaks as native forests are significantly less flammable than plantations and provide ecosystem services such as water provision, carbon sequestration, maintenance of soil fertility, recreation and habitat conservation. Such conversion would require large-scale restoration programmes designed and implemented through collaborations between the government, timber companies, NGOs and other landowners. This could be part of the restoration at the landscape scale of 1 million ha, committed by Chile as a National Determined Contribution to the Conference of the Parties (COP25, Madrid 2019) of the United Nations Framework Convention on Climate Change. This landscape-scale restoration appears to be the only realistic way to maintain sustainable timber supply from productive forest plantations that are compatible with the conservation of N. alessandrii stands and other forest types, as well as the provision of ecosystem services under current and future fire regimes and the drying and heating trends associated with climate change. This landscape restoration plan is ambitious but probably the only way to ensure the survival of the remaining N. alessandrii stands and the re-establishment of stands in the historical distribution of the species through restoration. The restoration programmes should also create buffer zones (or fire breaks) around remnant N. alessandrii stands when surrounded by exotic pine plantations, to protect them from fire and invasion by exotic species. These buffer zones should be 40–50 m wide and covered by short native shrubs surrounded by 40–100 m of thinned and pruned plantations, to reduce fuel load. These programmes should also create a financial foundation for the conservation, restoration and study of N. alessandrii stands, comprising public and private funding and led by stakeholders living in the Maule region, along with a strong outreach programme. A model for this kind of organization could be Corporación Alerce, which is dedicated to the conservation of Fitzroya cupressoides (alerce), a large, long-lived, threatened conifer in southern Chile and an iconic species that is more abundant than N. alessandrii. At the stand scale, the most urgent actions are to control the post-fire regeneration of pines established in N. alessandrii stands and to remove large adult pines from these stands. Effective land-use planning and the implementation of restoration and forest management strategies are priorities if we are to protect the Maulino forests and avoid the extinction of N. alessandrii forest ecosystems.
Acknowledgements
Research support was provided by Fondo Nacional de Desarrollo Científico y Tecnológico, Fondo de Financiamiento de Centros de Investigación en Áreas Prioritarias, Centre for Fire and Socioecosystem Resilience, and Forestal Mininco S.A. through the Ecological Restoration and Recovery of Ecosystem Services project. We thank Mauricio Barría for field assistance, Alamiro Navarrete from Forestal Mininco S.A. for assistance in the restoration project, Forestal Arauco S.A. for providing 5-m resolution digital elevation model products, and two anonymous reviewers for their helpful critiques.
Author contributions
Conception, design: MEG, MG, JL, CL, CB-R; data collection: MEG, MG, CB-R; data analysis, writing: MEG, MG, JL, CL, CB-R, AL, JSM; revision: all authors.
Conflicts of interest
None.
Ethical standards
This study abided by the Oryx guidelines on ethical standards.