Book contents
- The Cambridge Handbook of the Neuroscience of Creativity
- The Cambridge Handbook of the Neuroscience of Creativity
- Copyright page
- Contents
- Figures
- Tables
- Contributors
- Acknowledgments
- Introduction
- Part I Fundamental Concepts
- Part II Pharmacology and Psychopathology
- 5 Stress, Pharmacology, and Creativity
- 6 Functional Neuroimaging of Psychedelic Experience: An Overview of Psychological and Neural Effects and their Relevance to Research on Creativity, Daydreaming, and Dreaming
- 7 A Heated Debate: Time to Address the Underpinnings of the Association between Creativity and Psychopathology?
- 8 Creativity and Psychopathology: A Relationship of Shared Neurocognitive Vulnerabilities
- Part III Attention and Imagination
- Part IV Memory and Language
- Part V Cognitive Control and Executive Functions
- Part VI Reasoning and Intelligence
- Part VII Individual Differences
- Part VIII Artistic and Aesthetic Processes
- Index
- References
5 - Stress, Pharmacology, and Creativity
from Part II - Pharmacology and Psychopathology
Published online by Cambridge University Press: 19 January 2018
- The Cambridge Handbook of the Neuroscience of Creativity
- The Cambridge Handbook of the Neuroscience of Creativity
- Copyright page
- Contents
- Figures
- Tables
- Contributors
- Acknowledgments
- Introduction
- Part I Fundamental Concepts
- Part II Pharmacology and Psychopathology
- 5 Stress, Pharmacology, and Creativity
- 6 Functional Neuroimaging of Psychedelic Experience: An Overview of Psychological and Neural Effects and their Relevance to Research on Creativity, Daydreaming, and Dreaming
- 7 A Heated Debate: Time to Address the Underpinnings of the Association between Creativity and Psychopathology?
- 8 Creativity and Psychopathology: A Relationship of Shared Neurocognitive Vulnerabilities
- Part III Attention and Imagination
- Part IV Memory and Language
- Part V Cognitive Control and Executive Functions
- Part VI Reasoning and Intelligence
- Part VII Individual Differences
- Part VIII Artistic and Aesthetic Processes
- Index
- References
Summary
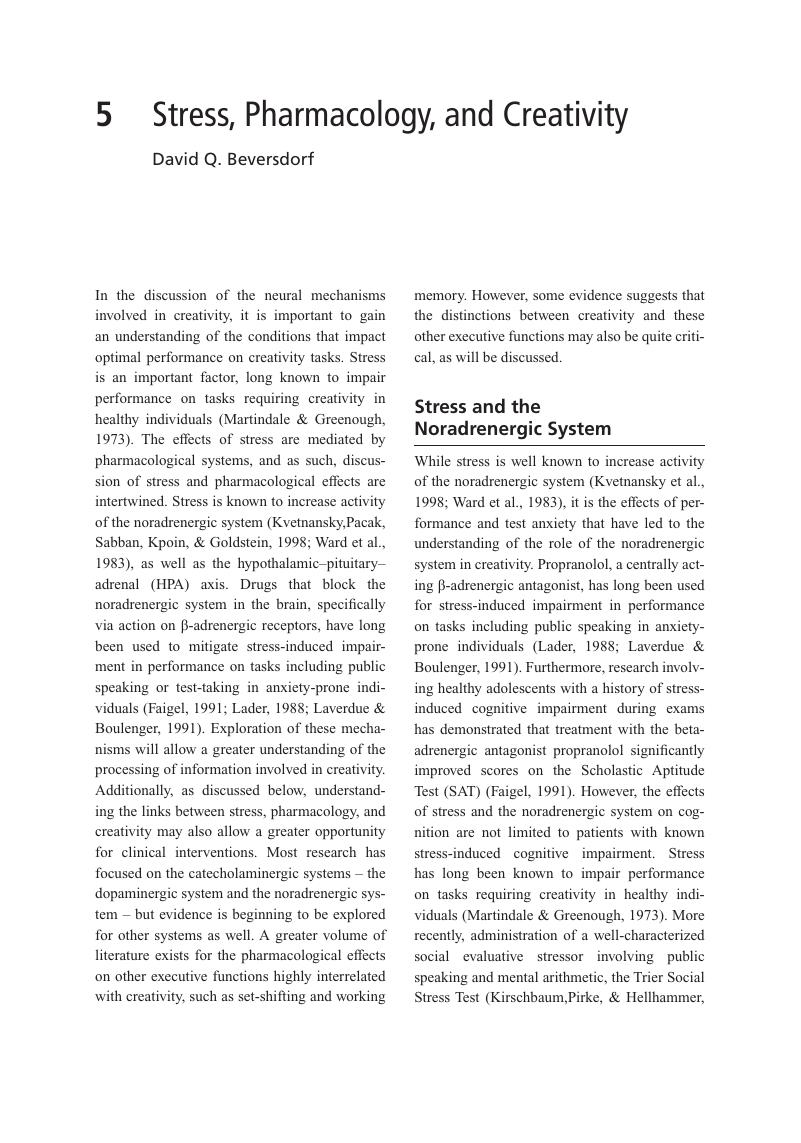
- Type
- Chapter
- Information
- The Cambridge Handbook of the Neuroscience of Creativity , pp. 73 - 91Publisher: Cambridge University PressPrint publication year: 2018
References
- 2
- Cited by