Book contents
- Liquid Cell Electron Microscopy
- Advances in Microscopy and Microanalysis
- Liquid Cell Electron Microscopy
- Copyright page
- Contents
- Contributors
- Preface and Acknowledgements
- Part I Technique
- 1 Past, Present, and Future Electron Microscopy of Liquid Specimens
- 2 Encapsulated Liquid Cells for Transmission Electron Microscopy
- 3 Imaging Liquid Processes Using Open Cells in the TEM, SEM, and Beyond
- 4 Membrane-Based Environmental Cells for SEM in Liquids
- 5 Observations in Liquids Using an Inverted SEM
- 6 Temperature Control in Liquid Cells for TEM
- 7 Electron Beam Effects in Liquid Cell TEM and STEM
- 8 Resolution in Liquid Cell Experiments
- Part II Applications
- Part III Prospects
- Index
- References
4 - Membrane-Based Environmental Cells for SEM in Liquids
from Part I - Technique
Published online by Cambridge University Press: 22 December 2016
- Liquid Cell Electron Microscopy
- Advances in Microscopy and Microanalysis
- Liquid Cell Electron Microscopy
- Copyright page
- Contents
- Contributors
- Preface and Acknowledgements
- Part I Technique
- 1 Past, Present, and Future Electron Microscopy of Liquid Specimens
- 2 Encapsulated Liquid Cells for Transmission Electron Microscopy
- 3 Imaging Liquid Processes Using Open Cells in the TEM, SEM, and Beyond
- 4 Membrane-Based Environmental Cells for SEM in Liquids
- 5 Observations in Liquids Using an Inverted SEM
- 6 Temperature Control in Liquid Cells for TEM
- 7 Electron Beam Effects in Liquid Cell TEM and STEM
- 8 Resolution in Liquid Cell Experiments
- Part II Applications
- Part III Prospects
- Index
- References
Summary
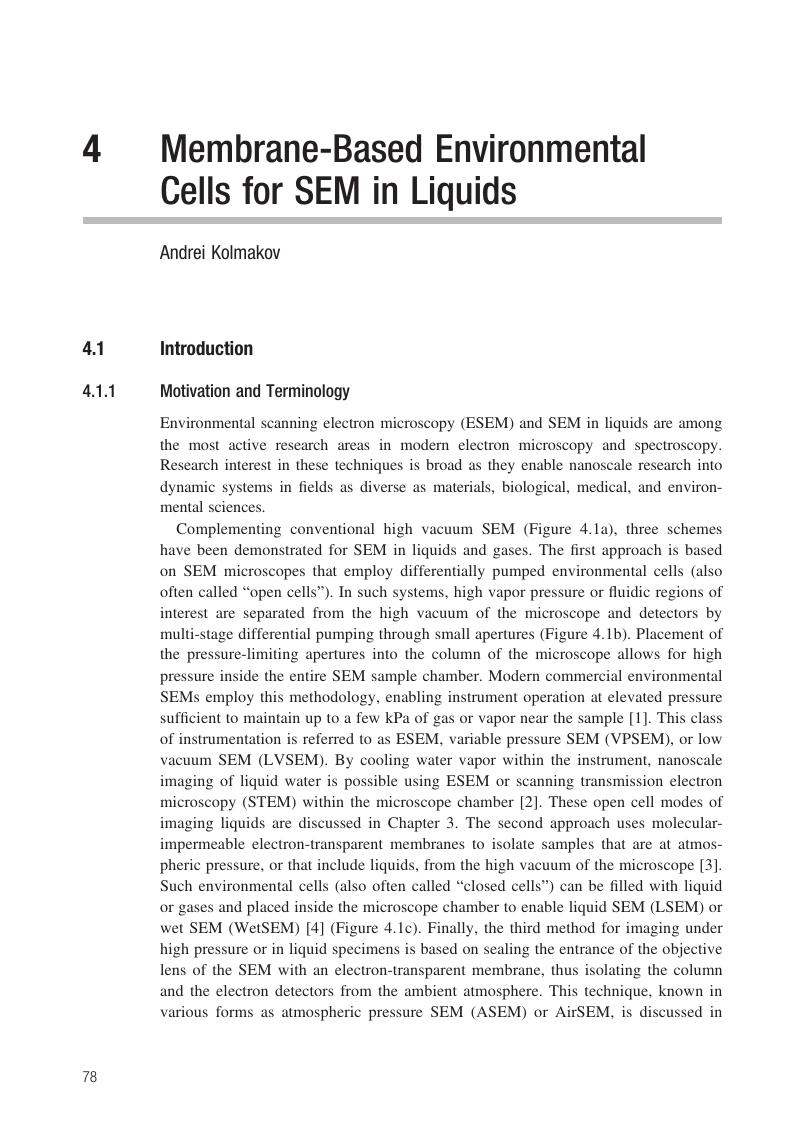
- Type
- Chapter
- Information
- Liquid Cell Electron Microscopy , pp. 78 - 105Publisher: Cambridge University PressPrint publication year: 2016
References
- 3
- Cited by