Introduction
Calcified fossil sponges and microbial carbonates are commonly closely associated in marine limestones (Pitcher, Reference Pitcher1964; Alberstadt et al., Reference Alberstadt, Walker and Zurawski1974; Klappa and James, Reference Klappa and James1980; Fagerstrom, Reference Fagerstrom1987; Desrochers and James, Reference Desrochers, James, Geldsetzer, James and Tebbutt1989; Wood, Reference Wood1990; Brunton and Dixon, Reference Brunton and Dixon1994; Reitner et al., Reference Reitner1995; Neuweiler et al., Reference Neuweiler, Reitner and Monty1997; Liu et al., Reference Liu, Rigby and Zhu2003; Li et al., Reference Li, Li, Zhang and Munnecke2017; Lee and Riding, Reference Lee and Riding2018) and can be confused with one another (Luo and Reitner, Reference Luo and Reitner2016). In some examples, the similarities are superficial, as in the case of Paleozoic stromatoporoid sponges and stromatolites, both of which can form layered domes but are usually readily distinguished by their internal structure since stromatoporoids are characterized by complex calcified skeletons (Stearn, Reference Stearn and Stearn2015a).
In other cases, distinguishing calcified sponges from microbial carbonates may not be so straightforward. Keratosan demosponges, which are widespread in marine environments at the present day (Maldonado and Young, Reference Maldonado and Young1998), provide an instructive example. They lack mineralized spicules and skeletons and rely for support on a flexible spongin network, widely familiar in the bath sponge, Spongia. So far as is currently known, the fossil record of calcified remains of keratosans appears to be due mainly to synsedimentary replacement by CaCO3 that preserves the delicate spongin framework as a microscopic anastomose network in fine-grained carbonate (Luo and Reitner, Reference Luo and Reitner2014). The identity of keratosan spongin preserved as permineralized microscopic sparry network was first recognized by Reitner et al. (Reference Reitner, Hühne and Thiel2001). Using serial sectioning, Luo and Reitner (Reference Luo and Reitner2014) demonstrated the similarity of the three-dimensional architecture of present-day spongin network to that of “putative fossils of keratose demosponges” from the Devonian and Triassic. This led to the realization that Phanerozoic keratosan fossils had probably often been misinterpreted as microbial carbonate (Luo, Reference Luo2015; Luo and Reitner, Reference Luo and Reitner2016) and to the recognition of well-preserved keratosan vermiform fabric (“keratolite”) intercalated within Cambrian–Ordovician stromatolites (Lee and Riding, Reference Lee and Riding2021a, Reference Lee and Ridingb) and occupying cavities in ~890 Ma reefs (Turner, Reference Turner2021).
Spongiostroma Gürich, Reference Gürich1906 is one of a number of enigmatic structures, internally patterned by small but complex fabrics, that form layered and domical masses in the lower Carboniferous of Belgium. Despite their name, which refers to their spongy appearance in thin section, Gürich (Reference Gürich1906, p. 5, 32) provisionally placed spongiostromides in protozoans (foraminifers). This suggestion was not adopted by subsequent workers who variously compared spongiostromides with hydrozoans (Rothpletz, Reference Rothpletz1908) and algae (cyanobacteria) (Garwood, Reference Garwood1914; Twenhofel, Reference Twenhofel1919, p. 340). This led Pia (Reference Pia and Hirmer1927, p. 36) to regard Spongiostroma as a stromatolite and to propose the name Spongiostromata to encompass stromatolites and oncolites in general. This approach was popularized by Johnson (Reference Johnson1961, p. 204). The outcome was that “spongiostromate” became widely used to describe “laminated, poorly differentiated micritic and peloidal microfabric” in carbonate sediments (Flügel, Reference Flügel2004, p. 373). Consequently, for nearly 100 years, Spongiostroma has been widely regarded as a stromatolite.
Here we refigure and redescribe the type material of Spongiostroma mæandrinum Gürich, Reference Gürich1906, the type species of Spongiostroma. Its pillow-like mesostructure is permeated by vermiform fabric and contains canal- and spongocoel-like openings. We interpret S. mæandrinum as the calcified remains of keratosan demosponge. If so, then S. mæandrinum was the first fossil keratosan to be given a name. The second was Vauxia gracilenta Walcott, Reference Walcott1920 from the Burgess Shale, originally described as a glass sponge (hexactinellid) (Walcott, Reference Walcott1920) and subsequently reassigned as a keratosan sponge (Rigby, Reference Rigby1986). Vauxiidae includes seven species of Vauxia and one species of Angulosuspongia, restricted to the Cambrian Burgess Shale-type fauna (Yang et al., Reference Yang, Zhao, Babcock and Peng2017; Luo et al., Reference Luo, Zhao and Zeng2020; Wei et al., Reference Wei, Zhao, Chen, Hou and Cong2021). Recently, Luo et al. (Reference Luo, Yang, Zhuravlev and Reitner2021) suggested archaeocyaths to be hypercalcified keratosan sponges, similar to Recent Vaceletia (Wörheide, Reference Wörheide2008). Recognition of Spongiostroma as a keratosan demosponge (1) enhances recognition of fossil sponges, (2) clarifies longstanding confusion between vermiform fabric and clotted microbial fabrics (Pia, Reference Pia and Hirmer1927; Johnson, Reference Johnson1961; Flügel, Reference Flügel2004; Kershaw et al., Reference Kershaw, Li and Li2021), and (3) qualifies the suggestion that Spongiostroma is not a sponge (Neuweiler et al., Reference Neuweiler, Kershaw, Boulvain, Matysik, Sendino, McMenamin and Woodonline preprint).
Materials and methods
Édouard-François Dupont, Director of the Musée royal d'Histoire naturelle in Brussels (currently the Museum of the Royal Belgian Institute of Natural Sciences), from 1868 to 1909, assembled a large collection of “sedimentary and constructed” limestones from the Devonian and Carboniferous of southern Belgium (Gürich, Reference Gürich1906, p. 5). These were prepared for display as large (mostly 20 × 20 cm, some 20 × 40 and 40 × 40 cm) thin sections on plate glass. In 1903 and 1904, Gürich (Reference Gürich1906, p. 5), motivated by his discovery of what he thought were Carboniferous (later recognized to be Famennian; Wolniewicz, Reference Wolniewicz2009) stromatoporoids near Krakow, Poland, visited Brussels to study the fabrics in the hundreds of “enormous transparent glass plates” displayed at the Museum. These “giant thin plates” were both a boon and a problem. They reveal large-scale structure, but due to their thickness, probably ~100 μm or more, the microstructure is often indistinct, as Rothpletz (Reference Rothpletz1908, p. 3) noted when comparing them with Silurian fossils. In addition, in some cases (e.g., Spongiostroma), the thin sections were only made parallel to bedding.
The specimens described by Gürich (Reference Gürich1906) were collected in the area of the river Meuse, ~60 km southeast of Brussels, between Namur and Huy, and south of Huy, and the locations are indicated only very generally by Gürich (Reference Gürich1906, p. 10). The type specimen of S. mæandrinum was collected from the V2d (uppermost Visean; see Groessens, Reference Groessens1989, table 2) near Namêche, 9 km east of Namur (Gürich, Reference Gürich1906, p. 41). We were unable to locate Spongiostroma in outcrops in this area in 2019. Due to their large size (20 × 20 cm), it is not possible to observe the thin sections of the type material with conventional petrographic microscopes. We photographed thin sections at the museum with a digital camera and microscope (Dino-Lite), using a light box for illumination. Our focus here is S. mæandrinum. We have not examined other Spongiostromidæ. So far as we are aware, this is the first time the type material of Spongiostroma has been refigured.
Repository and institutional abbreviation
Type material examined in this study is deposited in the Museum of the Royal Belgian Institute of Natural Sciences (IRSNB), Brussels, Belgium.
Systematic paleontology
Class Demospongiae Sollas, Reference Sollas1885
Order unknown
Family Spongiostromidæ Gürich, Reference Gürich1906
Remarks
Gürich (Reference Gürich1906, p. 5) based the Spongiostromidæ on samples from the Visean near Namur, Belgium. He regarded them as “encrusting organisms” that were neither stromatoporoids nor “stromatactides” even though they contained features he regarded as very similar to stromatoporoids. He suggested they might be protozoans (foraminifers): order Spongiostromaceae, family Spongiostromidæ (Gürich, Reference Gürich1906, p. 35, 53) and recognized five genera: Aphrostroma, Chondrostroma, Malacostroma, Pycnostroma and Spongiostroma.
Two groups of present-day sponge are nonspicular (i.e., lacking mineralized spicules) and supported by spongin fibers. These are the subclass Keratosa (orders Dictyoceratida and Dendroceratida) and the order Verongida in the subclass Myxospongiae (Wörheide et al., Reference Wörheide, Dohrmann, Erpenbeck, Larroux, Maldonado, Voigt, Borchiellini, Lavrov, Becerro, Uriz, Maldonado and Turon2012). Since “most of the taxonomically important features of nonspicular sponges, such as the microscopic structure of the skeletal fibers and the type of choanocyte chambers, are obscured or obliterated by the fossilization process” (Luo and Reitner, Reference Luo and Reitner2014, p. 468), it is not possible to confidently assign Spongiostromidæ to a specific order within Demospongiae, and we here regard Spongiostroma, the type genus of the family Spongiostromidæ, as a nonspiculate demosponge (phylum Porifera, class Demospongiae). Luo and Reitner (Reference Luo and Reitner2014) used “Keratosa” or “keratose sponges” for fossil sponges in the sense of Minchin (Reference Minchin and Lankester1900, p. 153–154), who defined the group as “Demospongiae in which the skeleton consists of fibers of spongin, without ‘proper’ spicules.” In this sense, most verongids could be included in “Keratosa.” Accordingly, we also use Keratosa to indicate the presence of a supportive spongin network. We therefore regard Spongiostroma as a keratosan demosponge sensu Minchin (Reference Minchin and Lankester1900).
S. mæandrinum is the type genus and species of the Spongiostromidæ. It remains to be seen whether other genera such as Aphrostroma, Chondrostroma, Malacostroma, and Pycnostroma, as well as other species of the genus Spongiostroma, including S. bacilliferum Gürich, Reference Gürich1906, S. ovuliferum Gürich, Reference Gürich1906, S. granulosum Gürich, Reference Gürich1906, S. balticum Rothpletz, Reference Rothpletz1908, and S. Holmi Rothpletz, Reference Rothpletz1908, are spongiostromides. Some of these fossils in Gürich's (Reference Gürich1906) illustrations resemble imperfectly preserved microbial carbonate.
Genus Spongiostroma Gürich, Reference Gürich1906
Type species
Spongiostroma mæandrinum Gürich, Reference Gürich1906
Diagnosis
As for the type species by monotypy.
Spongiostroma mæandrinum Gürich, Reference Gürich1906
Figures 1–3
- Reference Gürich1906
Spongiostroma mæandrinum Gürich, p. 41, pl. 6, fig. 1.
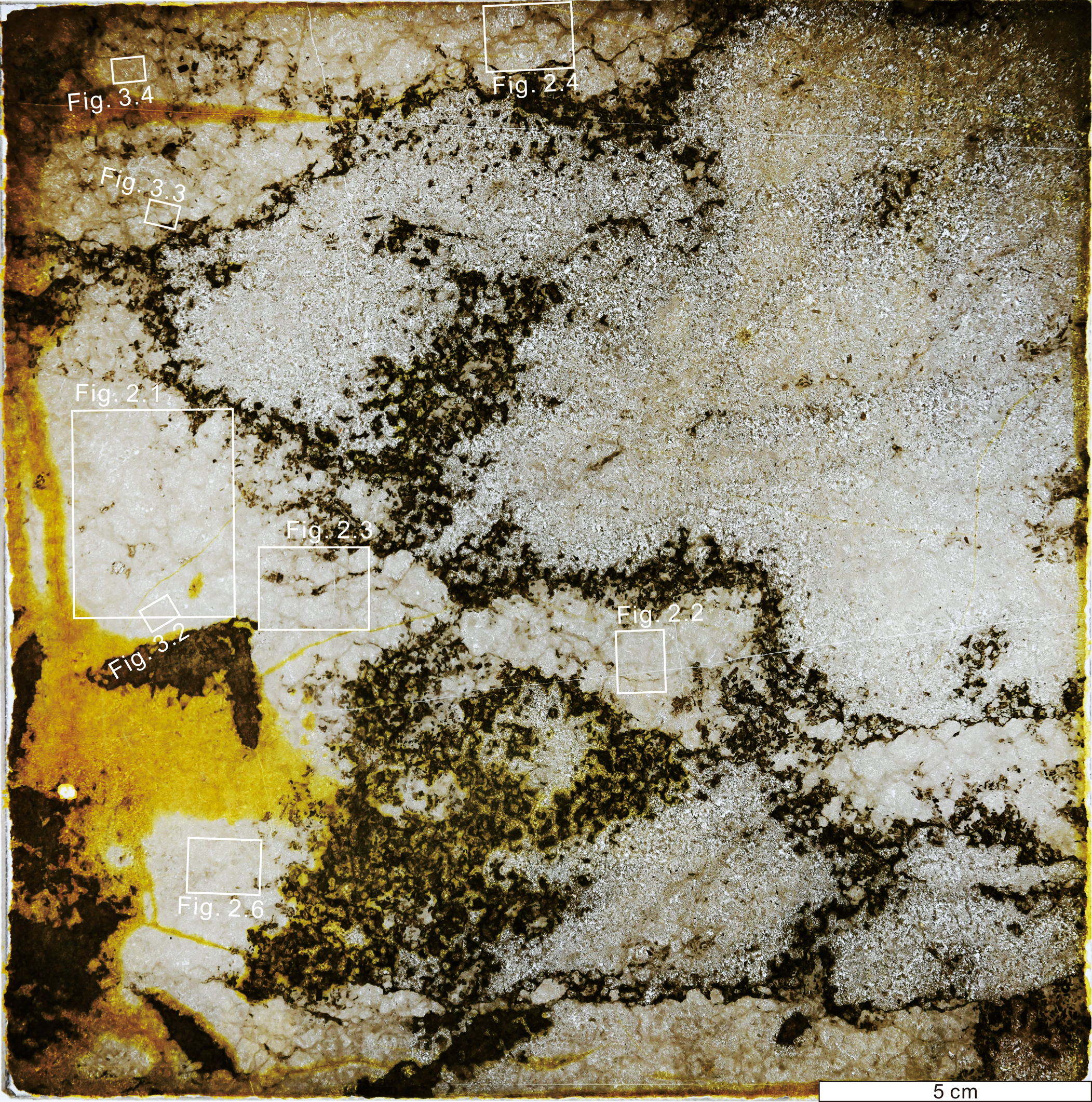
Figure 1. Type specimen of Spongiostroma mæandrinum (stored at the Museum of Natural Sciences of Belgium, IRSNB a 11037). The lighter areas, bounded by opaque material (possibly diagenetic kerogen), are locally sufficiently well preserved (e.g., top left, center left) to reveal the rounded to ovoid outlines of numerous juxtaposed millimetric pillow-like masses (interpreted as papillae) that constitute larger (≥5 cm) sponge lobes within carbonate sediment. Yellow areas are aged resin used in section preparation. White boxes indicate the areas shown in subsequent figures.

Figure 2. Photomicrographs of Spongiostroma mæandrinum. (1, 2) General views showing numerous juxtaposed millimetric pillow-like masses (interpreted as papillae). (2) Reproduction of Gürich (Reference Gürich1906, pl. 6, fig. 1). (3, 4) Closely juxtaposed papillae of differing size and shape, with darker interspaces occupied by micritic sediment, locally with opaque material. Contacts between papillae range from arcuate to linear. (5, 6) Details of papillae containing round to elongate areas filled with peloidal packstone (yellow dotted lines), interpreted as possible spongocoels. Rounded spar-filled areas (arrowed) in (4, 5) may represent canals.

Figure 3. (1) Detail of Figure 2.2 showing that the area figured by Gürich (Reference Gürich1906, pl. 6, fig. 1) is dominated by coarse (probably neomorphically enlarged) vermiform fabric. (2) Sediment-filled areas interpreted as possible spongocoels are outlined by yellow lines. Arrow indicates spar-filled area interpreted as a canal. (3) Examples of small papillae of varied size and shape bounded by thin darker micritic areas. (4) Branching structure (arrowed), possibly a canal, traversing vermiform fabric. (5, 6) Details of vermiform fabric, showing characteristic network. The fibers are variably, probably neomorphically, enlarged.
Holotype
Museum of the Royal Belgian Institute of Natural Sciences (plaque 229) (IRSNB a 11037).
Diagnosis
Millimetric, irregularly rounded, pillow-shaped juxtaposed papillae, consisting of thin anastomose sparry microscopic network in fine-grained groundmass (vermiform fabric); with sporadic round to stellate canals and locally traversed by rounded elongate curved spongocoels.
Occurrence
Uppermost Visean (V2d), Namêche, Belgium. Exact locality unknown.
Description
Horizontal section of clusters of irregularly rounded to amalgamate lobes (~5–15 cm) in peloidal to intraclastic micritic matrix. Each lobe consists of numerous juxtaposed subrounded polygonal to irregular rounded pillow-shaped papillae, ~1–10 mm (typically ~3–5 mm) across, outlined and bounded by diffuse sinuous, locally discontinuous, borders of darker micritic peloidal sediment up to ~200–500 μm wide. Papillae consist of a sparry network of irregularly anastomose fibers (vermiform fabric), mainly 25–45 μm wide and with branch internode distances mainly between 50–120 μm (Fig. 4) in generally micritic groundmass, locally patterned by faint and irregularly diffuse 30–50 μm size darker micritic bodies. Papillae locally contain rounded to stellate, locally branched, sparite-filled canals, 200–800 μm across, and are occasionally traversed by larger rounded curvilinear areas (spongocoel) ~1 mm wide, filled by peloidal sediment.
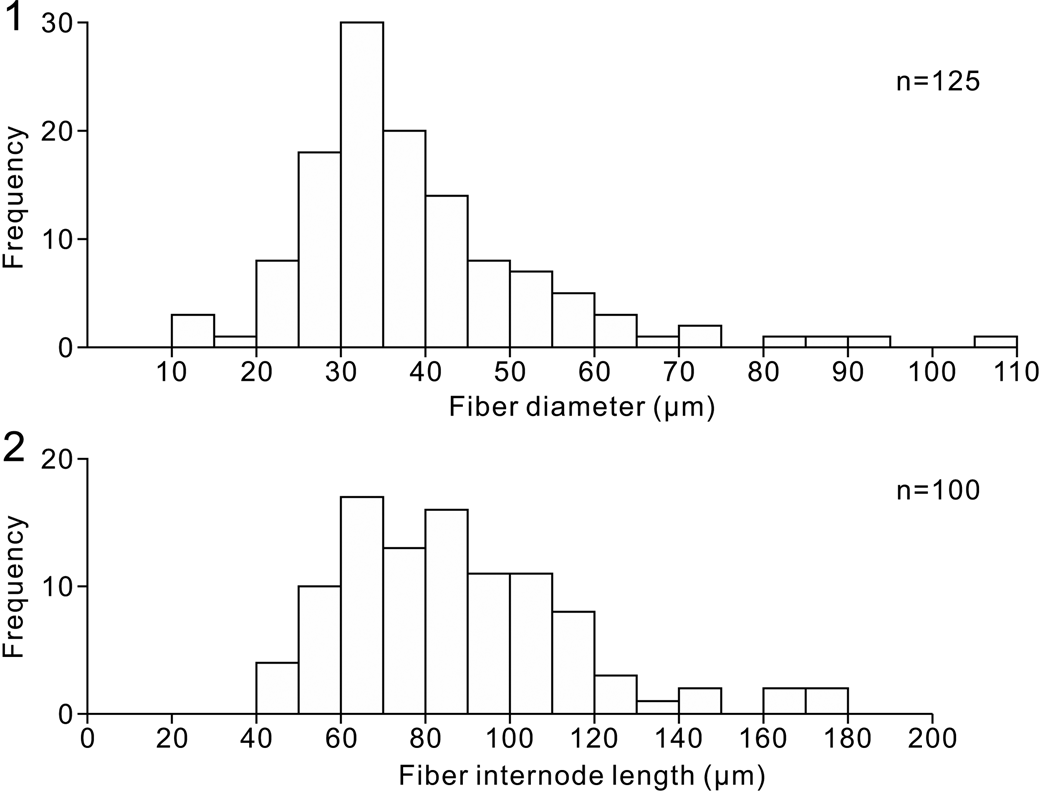
Figure 4. Vermiform fiber dimensions measured from Figure 3.6. (1) Histogram of vermiform fiber widths. (2) Histogram of vermiform fiber network internode distance.
Remarks
Gürich (Reference Gürich1906, p. 41) described Spongiostroma mæandrinum as follows: “Le tissu est relativement homogène et compact. Les granulations descendent jusqu'à 1/20 m et mesurent, en moyenne, 1/15 mm. Les canaux du tissu ont environ 1/40 mm. de diamètre. Dans le plan de la coupe, ils forment des dessins allongés ou ramifiés, en forme de couronnes ou de lignes brisées, constituant, en certains endroits, un réseau à mailles plus serrées et rappelant le tissu des Éponges. Les canaux coloniaux ne sont, ni nombreux, ni grands; très souvent des canaux coloniaux, d'environ 1/15 mm de diamètre, montrent une disposition circulaire.” (The fabric is relatively homogeneous and compact. The granules go down to about 1/20 m [authors’ comment: this appears to be a mistake for mm] and measure, on average, 1/15 mm. The tissue canals are about 1/40 mm in diameter. In the plane of the section, they form elongated or branched patterns, in the form of crowns or broken lines, constituting, in certain places, a tight network reminiscent of the fabric of sponges. The colonial canals are neither numerous nor large; very often the colonial canals, about 1/15 mm in diameter, show a circular arrangement.) Gürich (Reference Gürich1906, p. 41, 54) emphasized that the type specimen of S. mæandrinum is a horizontal section.
Two fabric features emphasized by Gürich (Reference Gürich1906) are “granulations” and “canaux du tissu.” The faintly patterned groundmass (“granulations” of Gürich, Reference Gürich1906, p. 40), barely discernible in Figure 3.5, 3.6, might reflect initial soft-tissue degradation by bacteria such as sulfate reducers (Luo and Reitner, Reference Luo and Reitner2014). They could also be an artifact of the relatively thick (maybe >100 μm) section that likely contains superimposed sparitic fibers. Further work is required to resolve this question. Here we consider the “canaux du tissu” (tissue canals) to be vermiform fabric and interpret them as calcified remains of keratosan spongin network (Luo and Reitner, Reference Luo and Reitner2014). Opaque material prominent between lobes and along their margins in the type specimen (Fig. 1), and locally between papillae (Figs. 2.1, 2.3, 2.4, 3.3), is probably diagenetic kerogen. Yellow areas (Fig. 1) are aged resin used in section preparation.
In S. mæandrinum, in addition to “canaux du tissu,” Gürich (Reference Gürich1906, p. 41) described “canaux coloniaux,” respectively ~25 and ~67 μm in diameter. Our measurements of the type specimen do not distinguish these and instead indicate that these vermiform fabric fibers are mainly 25–45 μm in width, within an overall size range of 10–110 μm (Fig. 4.1). These data can be compared with fibers that display more consistent width in other Mississippian examples, ~20 μm (Luo and Reitner, Reference Luo and Reitner2016), and in Ordovician examples, 35–50 μm (Park et al., Reference Park, Lee, Hong, Choh, Lee and Lee2015). Fibers with similarly uneven diameters occur in earliest Triassic examples (Wu et al., Reference Wu, Chen, Su, Fang and Yang2022, fig. 7). We therefore suggest that there is only a single population of “canals” in S. mæandrinum, representing vermiform fabric that was locally influenced by diagenetic enlargement, as Walter (Reference Walter1972, p. 12) observed. Nonetheless, we cannot exclude the possibility that these fibers might also reflect the size and shape of the original spongin network.
Gürich (Reference Gürich1906) did not mention the pillow-shaped mammiform papillae, and his single illustration (Gürich, Reference Gürich1906, pl. 6, fig. 1; Fig. 2.2) does not show them clearly, but they are evident elsewhere in the same section of the type specimen (Figs. 1, 2). We interpret S. mæandrinum as a lobate keratosan sponge composed of juxtaposed mammiform papillae in which adjacent papillae merge or are separated by diffuse and discontinuous peloidal micrite borders. We therefore regard the pervasive vermiform fabric of the papillae, evident in the type specimen (Figs. 2.2, 3.1, 3.5), as the calcified remains of spongin network; papillae are locally traversed by sediment-filled spongocoel (central cavities) (Figs. 2.5, 2.6, 3.2) and possibly by smaller canal systems preserved as rounded to stellate and branched spar-filled tubes (Figs. 2.4, 2.5, 3.2, 3.4). We infer that sediment-filled spongocoels were probably open to the exterior, whereas spar-filled canals were more isolated within the interior of the papillae.
Since the type specimen of S. mæandrinum is a horizontal thin section (Gürich, Reference Gürich1906, p. 41, 54), it does not show layering, and it is unclear whether the original sample was layered. The specimens assembled and prepared by Dupont were examined as thin sections by Gürich in the Museum (Gürich, Reference Gürich1906, p. 2). During a brief visit to Namur, Gürich (Reference Gürich1906, p. 10) noted that he “found Spongiostromides in the quarries immediately to the north of Namur, at many points, from Saint-Antoine, above Herbatte, to Grands-Malades station.” There is no indication that he visited the type locality of S. mæandrinum at Namêche.
Discussion
There is a long history of confusion between fossil calcified sponges and microbial carbonates. Stromatoporoids provide a classic example. These major components of mid-Paleozoic reefs are now widely regarded as calcified sponges comparable to coralline sponges (Stearn, Reference Stearn1972, Reference Stearn and Stearn2015b). However, their ability to form laminated calcareous layers and domes led to early comparisons with stromatolites (Fenton and Fenton, Reference Fenton and Fenton1937; Galloway and St. Jean, Reference Galloway and St. Jean1955; Kaźmierczak, Reference Kaźmierczak1980). In some cases, sponge versus stromatolite affinities of Ordovician domical fossils continue to be debated (Keller and Flügel, Reference Keller and Flügel1996; Stearn et al., Reference Stearn, Webby, Nestor and Stock1999, p. 23), but these uncertainties are usually resolved by distinct differences in internal structure since stromatoporoids often preserve skeletal elements such as laminae and pillars (Stearn et al., Reference Stearn, Webby, Nestor and Stock1999; Stearn, Reference Stearn and Stearn2015a), whereas stromatolite microfabrics are largely characterized by clotted and porostromate fabrics and trapped grains (Pia, Reference Pia and Hirmer1927; Flügel, Reference Flügel2004, p. 373). By contrast, the differences between calcified remains of keratosan sponge fossils and microbial carbonates have taken much longer to recognize. Calcified keratosans are characterized by microscopic anastomose fibers that represent the outlines of original spongin skeletal network. This “vermiform” fabric was initially interpreted as algal in origin (Walter, Reference Walter1972), and keratosan sponges were often misidentified as microbial until this fabric was recognized as spongin network (Reitner et al., Reference Reitner, Hühne and Thiel2001; Luo and Reitner, Reference Luo and Reitner2014).
Spongiostroma as a foraminifer or hydrozoan.—Spongiostroma, together with other encrusting, locally domical, genera (Aphrostroma, Pycnostroma, Chondrostroma, Malacostroma), was described by Gürich (Reference Gürich1906, Reference Gürich1907) from Mississippian limestones of Belgium. Gürich (Reference Gürich1906, p. 35) emphasized their layered spongy fabrics “traversé, sans aucune régularité, par des canaux plus fins … et par des canaux plus larges” (traversed without any regularity by narrow and wider canals). He realized that they were neither stromatoporoids nor “stromatactides” and placed them in new taxa: the family Spongiostromidæ under the order Spongiostromaceae (Gürich, Reference Gürich1906, p. 32). He recognized spicule-like structures in Pycnostroma (Gürich, Reference Gürich1906, pl. 6, fig. 2) (we note that these resemble originally siliceous demosponge spicules that are now disarticulated) but decided that they were canals. Gürich (Reference Gürich1906, Reference Gürich1907) seriously considered the possibility that Spongiostroma could be a sponge, but he was unable to identify the body cavity. Consequently, he provisionally referred the Spongiostromaceae as a whole to the Protozoa (foraminifers). He explained his reasoning as follows: “D'autre part, le système général des canaux clairs du tissue montre, en certains endroits, un aspect rappelant la structure des Spongiaires. La masse claire de calcite serait, alors, le squelette de l'éponge, et la masse trouble, les indications de la substance organique. Mais, alors, les espaces pour la cavité du corps manqueraient. Ce n'est que chez les Protozoaires qu'on peut attendre aussi peu de régularité dans la distribution du squelette, et je propose, à cause de cela, de placer, provisoirement, le groupe entier des Spongiostromacés parmi les Protozoaires, ou ils représenteraient un ordre special” (On the other hand, the general system of the clear canals of the tissue shows, in certain places, an aspect reminiscent of the structure of sponges. The mass of clear calcite would then be the skeleton of the sponge, and the cloudy mass an indication of the organic material. But, then, the spaces for the cavity of the body would be missing. It is only in the Protozoa that one can expect so little regularity in the distribution of the skeleton, and because of this, I propose to provisionally place the entire group of Spongiostromaceae among the Protozoa, where they represent a special order) (Gürich, Reference Gürich1906, p. 32). This comparison may have been encouraged by Dawson's (Reference Dawson1865, Reference Dawson1876) foraminiferal interpretation of Neoproterozoic Eozoön from Québec, Canada, most specimens of which have since been interpreted as inorganic (O'Brien, Reference O'Brien1970; Hofmann, Reference Hofmann1971; Adelman, Reference Adelman2007).
Gürich's (Reference Gürich1906) comparison of Spongiostromidæ with protozoans was not accepted by subsequent researchers. Rothpletz (Reference Rothpletz1908, p. 3–4) described oncoid-like nodules from the Silurian of Gotland, Sweden. In addition to Girvanella and Solenopora, he observed a fossil broadly resembling calcareous algae, sponges, and hydrozoans that he was unable to identify until he read Gürich (Reference Gürich1906). Consequently, in addition to Solenopora and Sphaerocodium, Rothpletz (Reference Rothpletz1908, p. 17) recognized two new species of Spongiostroma. He placed them in the hydrozoans, noting that the nodules they form are “easily confused with those of Sphaerocodium and Solenopora” but that in detail they lack both the radial-fibrous structure of Solenopora and the delicate filaments typical of Sphaerocodium. Rothpletz's (Reference Rothpletz1908, pls. 5, 6) illustrations of these fossils (Spongiostroma balticum and S. holmi) show complex chambered and/or tubiform fabrics. These fabrics do not closely resemble those of S. mæandrinum, although they could be similar to some of the other spongiostromides described by Gürich (Reference Gürich1906). Rothpletz (Reference Rothpletz1913, pl. 7, fig. 3) again figured Spongiostroma with Sphaerocodium (Rothpletzella) from the Silurian of Gotland and continued to regard it as a hydrozoan. The magnification of this illustration is too low to resolve the details.
Spongiostromata as cyanobacteria
Garwood (Reference Garwood1914, p. 269) described Gürich's (Reference Gürich1906) suggestion of a protozoan (foraminiferal) affinity for Spongiostroma as “decidedly speculative,” adding “the features which he selects for the subdivision of the different forms into genera and species are very indefinite.” Garwood (Reference Garwood1914) broadly compared “Spongiostroma” with fabrics in crusts and nodules associated with Aphralysia and Ortonella in a lower Carboniferous “Algal Layer” in northern England. He figured a specimen attributed to Spongiostroma cf. Malacostroma concentricum (Garwood, Reference Garwood1914, pl. 21, fig. 1) that he suggested (Garwood, Reference Garwood1914, p. 271) could be “a flocculent precipitate of carbonate of lime.” Similarly, Twenhofel (Reference Twenhofel1919, p. 3439) considered Spongiostroma, together with Cryptozoön, to be algal (cyanobacterial).
Pia (Reference Pia and Hirmer1927, p. 36–37) regarded Spongiostroma as a stromatolite and created the Spongiostromata as an overarching term to encompass “Stromatolithi” and “Oncolithi.” He categorized Spongiostromata as calcified cyanobacterial (thallophyte) deposits that in general “show no clear organic structure, but often characteristic growth-forms.” This approach was adopted by Johnson (Reference Johnson1942, Reference Johnson1946, Reference Johnson1951, Reference Johnson1961, Reference Johnson1963), who placed stromatolite-like forms in the family or “section” Spongiostromata under Cyanophyta. Johnson (Reference Johnson1951, p. 29) wrote: “Pia erected this family to include the great number of fossils which form spongy colonies of molds of algal threads.” Commenting on Spongiostromata and stromatolites, Johnson (Reference Johnson1961, p. 204) noted that “the two terms have become more or less synonymous.” adding “These fossils show no clear or usable microstructure.” Monty (Reference Monty and Flügel1977, p. 16) regarded spongiostromids as stromatolitic, and “spongiostromate microstructures” as “cryptalgal” (Monty, Reference Monty1981, p. 2).
Spongiostromate and clotted fabric
Stromatolites (Kalkowsky, Reference Kalkowsky1908) can be described according to their internal layering and external shape (“form genera and form-species,” Fenton and Fenton, Reference Fenton and Fenton1933; Semikhatov and Raaben, Reference Semikhatov, Raaben, Riding and Awramik2000), as well as with respect to their microfabrics (Pia, Reference Pia and Hirmer1927). Both approaches have long been employed in stromatolite description, with “spongiostromate” (Johnson, Reference Johnson1963) widely used to broadly describe common microfabrics. Hofmann (Reference Hofmann1978, p. 572) wrote: “The two independent and fundamentally different concepts associated with stromatolitology since the beginning of this century are the spongiostrome concept of Gürich (Reference Gürich1906) and the stromatolite concept of Kalkowsky (Reference Kalkowsky1908). Spongiostromid ‘genera’ (and ‘species’) were defined on the basis of microstructure (see Gürich (Reference Gürich1906), pp. 53–55; (Reference Gürich1907), pp. 137, 138), whereas stromatolite ‘genera’ (Pia, Reference Pia and Hirmer1927, p. 37) were based on gross morphology and lamina shape.” Pratt (Reference Pratt1982, p. 1216) described “clotted, spongiform microstructure consisting of often indistinct, silt-sized micrite peloids separated by interparticle and tiny fenestral pores.” Flügel (Reference Flügel2004, p. 373) stated: “Spongiostromate refers to a laminated, poorly differentiated micritic and peloidal microfabric.” Spongiostromate continues to be used in this sense (e.g., Martindale et al., Reference Martindale, Corsetti, James and Bottjer2015; Vennin et al., Reference Vennin2019; Bosence and Gallois, Reference Bosence and Gallois2022; Claussen et al., Reference Claussen, Munnecke and Ernst2022), although “grumous” has been recommended as the preferred term (Grey and Awramik, Reference Grey and Awramik2020, p. 213, 224). This latter term, derived from “structure grumeleuse” (clotted structure), was originally used to describe Mississippian limestones by Cayeux (Reference Cayeux1935, p. 271) as “many little clots of an extremely finely crystalline calcite, standing out as dark grey in a matrix of colourless granular calcite” (Bathurst, Reference Bathurst1976, p. 511). Cayeux (Reference Cayeux1935, p. 271) suggested that it was a product of incomplete recrystallization. However, as noted by Bathurst (Reference Bathurst1976, p. 512–513), other interpretations are possible and need to consider that “Structure grumeleuse is common in algal stromatolites.”
Whereas vermiform fabric is relatively uniform, clotted fabric can show more variation. Clotted structure (structure grumeleuse) and clotted peloidal fabric are characterized by merging of micrite patches (Bathurst, Reference Bathurst1976, fig. 350) and can be described as “irregular micritic peloidal aggregates surrounded and traversed by microspar” (Riding and Tomás, Reference Riding and Tomás2006, p. 23, fig. 7). These “peloidal aggregates” are characterized by a wide size range (~5–70 μm) and varied density of peloid spacing (Riding and Tomás, Reference Riding and Tomás2006), in contrast to vermiform fabric with spar-filled areas of more regular width (Figs. 3.5, 3.6, 4). Clotted fabric has long broadly been suggested to be a product of bacterial calcification (Kaisin, Reference Kaisin1925; Chafetz, Reference Chafetz1986, fig. 3; Riding, Reference Riding2000, p. 187). Our recognition of vermiform fabric, canals, and other openings in the type specimen support recent suggestions (Lee and Hong, Reference Lee and Hong2019, fig. 6a; Lee and Riding, Reference Lee and Riding2021b, table 1) that Spongiostroma could be a keratosan sponge. If this is correct, then it would be inappropriate and confusing to continue to use “spongiostromate” to describe clotted-peloidal fabrics in stromatolites and other microbial carbonates.
Vermiform fabric and keratosan spongin network
Describing Cambrian fossils that he considered to be the stromatolites Madiganites mawsoni and Ilicta composita, Walter (Reference Walter1972, p. 82) noted a distinctive microfabric that he named vermiform microstructure: “It consists of narrow, sinuous areas of sparry carbonate surrounded by darker, usually finer-grained, carbonate. In the known examples …. the sparry patches are less than 100 μ wide and reach a millimetre or more in length” (Walter, Reference Walter1972, p. 24). He interpreted vermiform fabric as the remains of filamentous algae: “The filaments responsible for the vermiform microstructure were coarse, about 30–60 μ wide. …. Although the vermiform structures are unusually coarse for blue-green algae, they are finer than the coarsest extant blue-greens. They are comparable in size to the small filamentous red and green algae” (Walter, Reference Walter1972, p. 87). Vermiform fabric has since been recognized as the calcified outlines of the originally proteinaceous spongin network of keratosan sponges (Reitner et al., Reference Reitner, Hühne and Thiel2001; Luo and Reitner, Reference Luo and Reitner2014, Reference Luo and Reitner2016, p. 565; Lee and Riding, Reference Lee and Riding2021a, Reference Lee and Ridingb). Well-preserved examples of vermiform fabric show that, unlike algal filaments, it is anastomose, i.e., interconnected (Luo and Reitner, Reference Luo and Reitner2014; Lee and Riding, Reference Lee and Riding2021b, fig. 8c). Further work is required to discover whether Madiganites and Ilicta are keratosan sponges or, perhaps, as in the example of Cryptozoön (Lee and Riding, Reference Lee and Riding2021a), interlayered keratosan and microbial carbonate.
Walter (Reference Walter1972, p. 12) noted that vermiform microstructure is readily obscured by recrystallization: “With slight alteration it forms a grumous microstructure.” This could help account for Pia's (Reference Pia and Hirmer1927) creation of the term “Spongiostromata” to encompass stromatolites and oncolites composed of a wide range of micritic–sparitic fabrics that he described as showing “no clear organic structure” (Pia, Reference Pia and Hirmer1927, p. 36). As observed in Spongiostroma, enlargement—presumably diagenetic—of vermiform fabric fibers is likely to have increased their width (Fig. 4). This may have contributed to the development of micritic areas that locally appear to be surrounded by interconnected sparry fenestrae (Fig. 3.5, 3.6). This supports Walter's (Reference Walter1972) observation that diagenetic alteration can both modify and obscure vermiform fabric.
Fossil keratosan macrostructure
Recognition of well-preserved vermiform fabric is a testament to the ability of the outlines of delicate organic fabrics to be preserved in carbonate sediments throughout the Phanerozoic and longer (Luo and Reitner, Reference Luo and Reitner2016; Turner, Reference Turner2021). However, for a long time, failure to recognize other macro-features of fossil calcified keratosans hindered their identification, and reliance on vermiform fabric alone has led to uncertainty (Kershaw et al., Reference Kershaw, Li and Li2021; Neuweiler et al., Reference Neuweiler, Kershaw, Boulvain, Matysik, Sendino, McMenamin and Woodonline preprint).
Spongiostroma exemplifies the difficulties encountered by early researchers in endeavoring to distinguish calcified fossils of sponges from foraminifers, hydrozoans, and microbial carbonates, even though—as we show here—S. mæandrinum appears to preserve not only vermiform microfabric but also macrostructural sponge features such as canals and spongocoel, as well as lobate outlines with mammiform papillae similar to some modern sponges (Fig. 5). In hindsight, it appears that, in addition to vermiform fabric (Luo and Reitner, Reference Luo and Reitner2016), a variety of additional morphological evidence supporting keratosan recognition is already available. In the following, we summarize some reported examples of macrofabrics that have been interpreted as remains of calcified fossil keratosan sponges, other than Spongiostroma.
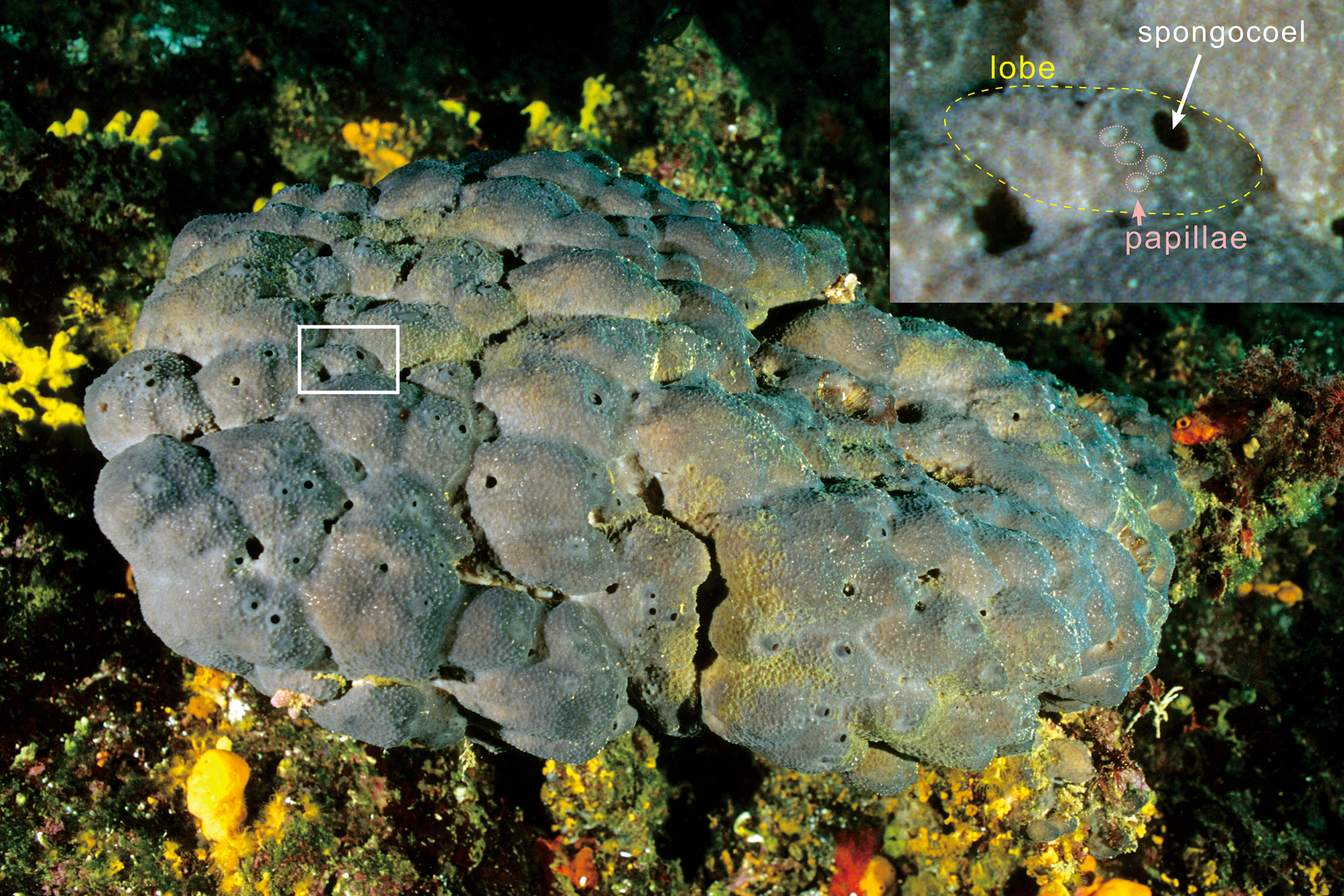
Figure 5. Present-day keratosan sponge (Spongia officinalis Linnaeus, Reference Linnaeus1759) (from WILDLIFE GmbH, 2002; location and scale unknown), organized into multiple juxtaposed lobes with spongocoel openings and patterned by innumerable papillae. We envisage that this morphology could approximate that of Spongiostroma mæandrinum.
Vermiform fabric in cavities and interspaces
Vermiform fabric has been reported as cavity fill within reefs or shells (e.g., Reitner et al., Reference Reitner, Hühne and Thiel2001; Hong et al., Reference Hong, Choh and Lee2014; J.-H. Lee et al., Reference Lee, Chen, Choh, Lee, Han and Chough2014, Reference Lee, Hong, Lee and Choh2016, Reference Lee, Dattilo, Mrozek, Miller and Riding2019; Park et al., Reference Park, Lee, Hong, Choh, Lee and Lee2017; M. Lee et al., Reference Lee, Elias, Choh and Lee2019; Turner, Reference Turner2021). Within the shells, it often fills the lower part of the cavity. In this situation it can create “geopetal” structures overlain by peloidal cavity fills that are again overlain by spar-filled areas (e.g., Lee et al., Reference Lee, Hong, Lee and Choh2016, figs. 4c, 5b; Park et al., Reference Park, Lee, Hong, Choh, Lee and Lee2017, fig. 3e; M. Lee et al., Reference Lee, Elias, Choh and Lee2019, fig. 5b, f). Vermiform fabric also occurs between Early Triassic crystal fans and dendrolites (e.g., Friesenbichler et al., Reference Friesenbichler, Richoz, Baud, Krystyn, Sahakyan, Vardanyan, Peckmann, Reitner and Heindel2018; Heindel et al., Reference Heindel2018; Pei et al., Reference Pei, Duda, Schönig, Luo and Reitner2021; Wu et al., Reference Wu, Chen, Su, Fang and Yang2022).
Millimetric layers intercalated with microbial carbonate
Vermiform fabric has been recognized forming very thin layers alternating with microbial carbonate (Luo and Reitner, Reference Luo and Reitner2016; Stock and Sandberg, Reference Stock and Sandberg2019; Lee and Riding, Reference Lee and Riding2021a, Reference Lee and Ridingb; Pei et al., Reference Pei, Duda, Schönig, Luo and Reitner2021, Reference Pei, Hagdorn, Voigt, Duda and Reitner2022). Lee and Riding (Reference Lee and Riding2021a) suggested that steep margins observed in late Cambrian Cryptozoön could reflect the ability of keratosan sponges to encrust steep overhanging surfaces.
External morphology
Distinctive lenticular outlines of keratosans (often initially described as non-lithistid demosponges) on millimetric–centimetric scales have been illustrated within fine-grained carbonates from both shallow- and relatively deep-water environments, e.g., upper Cambrian, Korea (Lee et al., Reference Lee, Cho, Jung, Choh and Lee2021, fig. 6), Upper Ordovician of South China (Park et al., Reference Park, Lee, Hong, Choh, Lee and Lee2015, figs. 4, 6), Permian/Triassic boundary of Iran (Luo, Reference Luo2015, fig. 5.6). External morphology is also locally visible in keratosans in reef cavities, e.g., Lower Ordovician of Korea (Hong et al., Reference Hong, Choh and Lee2014, figs. 2, 3) and upper Cambrian of Nevada (J.-H. Lee et al., Reference Lee, Dattilo, Mrozek, Miller and Riding2019, fig. 7a, f).
Canals
In addition to the canal-like structures reported here in Spongiostroma, cylindrical microspar-filled branching structures about 10 times larger than the vermiform fabric have also been described as canals (Luo and Reitner, Reference Luo and Reitner2016, fig. 5b, e, h).
Spongocoel
The presence of spongocoel, filled by micrite, has been reported in Cambrian reef framework in Shandong, China (Lee et al., Reference Lee, Chen, Choh, Lee, Han and Chough2014, fig. 7b; Lee and Hong, Reference Lee and Hong2019, fig. 3e).
Conclusions
Gürich (Reference Gürich1906) compared Mississippian Spongiostroma with sponges but was unable to identify canal-like openings, and provisionally assigned it to Foraminifera. Here we show that the type species, S. mæandrinum, is organized into papillae-like structures ~2–10 mm across that are pervasively composed of vermiform fabric and are traversed by narrow canals and contain larger spongocoel openings. We therefore interpret Spongiostroma as the calcified remains of a keratosan demosponge that originally had a fibrous spongin network.
Recognition of the poriferan nature of Spongiostroma, based on macrostructural features as well as its vermiform microfabric, provides criteria to assist discrimination between keratosan demosponges and stromatolite. Vermiform fabric is interpreted as calcified remains of spongin network. Changes to its size and regularity caused by neomorphic diagenesis can produce fabrics somewhat resembling microbial clotted peloidal fabric. This has resulted in a century of confusion during which time the term “spongiostromate” became a synonym for clotted-peloidal microbial fabrics. However, since S. mæandrinum, the type species of the family Spongiostromidæ and of the genus, is not a stromatolite, it is inappropriate to use the term “spongiostromate” to describe microbial microfabrics.
Acknowledgments
For assistance and access to collections at the Museum of the Royal Belgian Institute of Natural Sciences in Brussels, we thank A. Folie in 2019 and the late A. Dhondt, who hosted R.R. in 1991. We are grateful to J. Denayer (Université de Liège) for showing us field localities between Namur and Liège. M.C. Díaz (Florida Atlantic University, USA) and K.J. Lee (National Institute of Biological Resources, Incheon, Korea) kindly provided advice on present-day keratosans. We thank S. Kershaw and an anonymous reviewer for their comments and O. Vinn for his helpful editorial advice. J.H.L. was supported by a grant from Chungnam National University.
Competing Interests
The authors declare none.