Introduction
During their study of woodrat origins, Martin and Zakrzewski (Reference Martin and Zakrzewski2019) briefly reviewed the taxonomic history and dental morphology of an enigmatic Late Miocene cricetid rodent, Pliotomodon primitivus Hoffmeister, Reference Hoffmeister1945, from sites in northern California. The localities were first reported by Stirton (Reference Stirton1939), and Hoffmeister (Reference Hoffmeister1945) later described the new genus and species Pliotomodon primitivus and allocated a few other specimens to Peromyscus sp. Hoffmeister (Reference Hoffmeister1945) regarded the closest morphological match of P. primitivus to be an Old World cricetodontine, Cricetodon sansaniensis Lartet, Reference Lartet1851 (illustrated by Schaub, Reference Schaub1925) from the mid-Miocene Sansan assemblage of France. In his description of a new extinct subgenus of Neotoma, Paraneotoma, Hibbard (Reference Hibbard1967, p. 128) concluded that Pliotomodon primitivus was “…a specialized side branch that split off from the ancestral Neotoma stock during Lower Pliocene time.” Probably based on a general similarity of occlusal dental pattern, Hooper (Reference Hooper1972) suggested that Pliotomodon might be related to the singing mice, Scotinomys. Later, de Bruijn (Reference de Bruijn1976) noted a similarity in dental pattern between the Old World cricetodontine Byzantinia and Pliotomodon, but decided the similarity was due to parallel evolution. Jacobs (Reference Jacobs1977, p. 517) noted that his new genus, Galushamys, from the Late Miocene Redington assemblage of Arizona, “…superficially resembles Pliotomodon…” but also pointed out characters in the dentition of Pliotomodon that precluded a close relationship with Galushamys. Jacobs (Reference Jacobs1977) concluded that similarities of Pliotomodon to Galushamys were the result of parallelism and suggested that Pliotomodon was a Hemphillian Old World immigrant, with some dental similarities to Byzantinia hellenicus Freudenthal, Reference Freudenthal1970 (reported by Jacobs, Reference Jacobs1977, as Ruscinomys hellenicus), an extinct cricetodontine from Greece. May (Reference May1981) reviewed most of the above history and, based on a number of dental characters, concurred with Jacobs (Reference Jacobs1977) that Pliotomodon was an Asian immigrant closely related to Byzantinia. Lindsay (Reference Lindsay, Janis, Gunnell and Uhen2008) classified Pliotomodon with Galushamys in a new tribe of Cricetodontinae, the Galushamyini. Martin and Zakrzewski (Reference Martin and Zakrzewski2019) noted a similarity of the dentition of Pliotomodon to Byzantinia and agreed with previous authors that Pliotomodon was likely an Asian immigrant unrelated to their redefined Galushamyina.
In this study we re-examined the known Pliotomodon fossil material and compared it with undescribed or minimally described specimens of Galushamys and the Old World cricetodontines, resulting in an analysis that more clearly illustrates the potential phylogenetic relationships of Pliotomodon. We also examined an undescribed taxon from the Warm Springs region of the late Oligocene or Early Miocene (late Arikareean) John Day Formation of Oregon that Dingus (Reference Dingus1978) suggested might be allied with the Old World genus Eumyarion, which later was noted by Lindsay (Reference Lindsay, Janis, Gunnell and Uhen2008) as “Deperetomys” sp.
Classification of the Old World muroids, in particular those taxa that historically have been considered cricetids, has long been a challenge. The evolution of cricetid-like rodents is rather complex, with a number of early clades in addition to the modern hamsters and their extinct relatives (Cricetinae). For example, Maridet and Ni (Reference Maridet and Ni2013) recognized a substantial diversity of subfamilies and included Deperetomys in the Cricetopinae of McKenna and Bell (Reference McKenna and Bell1997) rather than in the Cricetodontinae. While admitting to the complexity of early cricetid evolution, López-Guerrero (Reference López-Guerrero2014) and Marković et al. (Reference Marković, de Bruijn, van de Weerd and Wessels2020) chose to retain Deperetomys within the Cricetodontinae, and we tentatively adopt their content of the Cricetodontinae here.
Stratigraphic setting and age of the Mulholland and Warm Springs assemblages
The Pliotomodon specimens reported here were recovered from two localities, UCMP V3611 (Mulholland 2) and UCMP V3303 (Mulholland 1 = Saint Mary's banks), in the town of Moraga, Contra Costa County, California. These localities were previously regarded as occurring in the Mulholland, Orinda, or Tassajara formations (Stirton, Reference Stirton1939; Hoffmeister, Reference Hoffmeister1945; Savage et al., Reference Savage, Ogle and Creely1951; Creely et al., Reference Creely, Savage, Ogle, Ingersoll and Woodburne1982), but Wagner et al. (Reference Wagner, Deino, Edwards, Sarna-Wojcicki, Wan, Sullivan, Sloan, Unruh and Schwartz2021) recently reevaluated the stratigraphy and nomenclature of the formations in the Contra Costa Group of the eastern San Francisco Bay area (Fig. 1.1). In the Wagner et al. (Reference Wagner, Deino, Edwards, Sarna-Wojcicki, Wan, Sullivan, Sloan, Unruh and Schwartz2021) southern section, UCMP V3611 occurs near the base of the Garrity Formation and UCMP V3303 occurs in about the upper third of the Lower Mulholland Member of the Siesta Formation. The Garrity Formation and the Upper Mulholland Member in the southern section are primarily fluvial in origin, consisting of sandstone, conglomerate, siltstone, and mudstone (Wagner et al., Reference Wagner, Deino, Edwards, Sarna-Wojcicki, Wan, Sullivan, Sloan, Unruh and Schwartz2021). In the northern outcrops, Wagner et. al. (Reference Wagner, Deino, Edwards, Sarna-Wojcicki, Wan, Sullivan, Sloan, Unruh and Schwartz2021) recognized two members (lower and upper) in the Garrity Formation. However, in the southern outcrops, these members could not be distinguished, so they referred the southern exposures to the Garrity Formation undifferentiated. The Lower Mulholland Member is dominated by sandstone intercalated with mudstone and siltstone beds, primarily fluvial in origin, along with minor shallow lacustrine and brackish estuarine deposits (Wagner et al., Reference Wagner, Deino, Edwards, Sarna-Wojcicki, Wan, Sullivan, Sloan, Unruh and Schwartz2021).
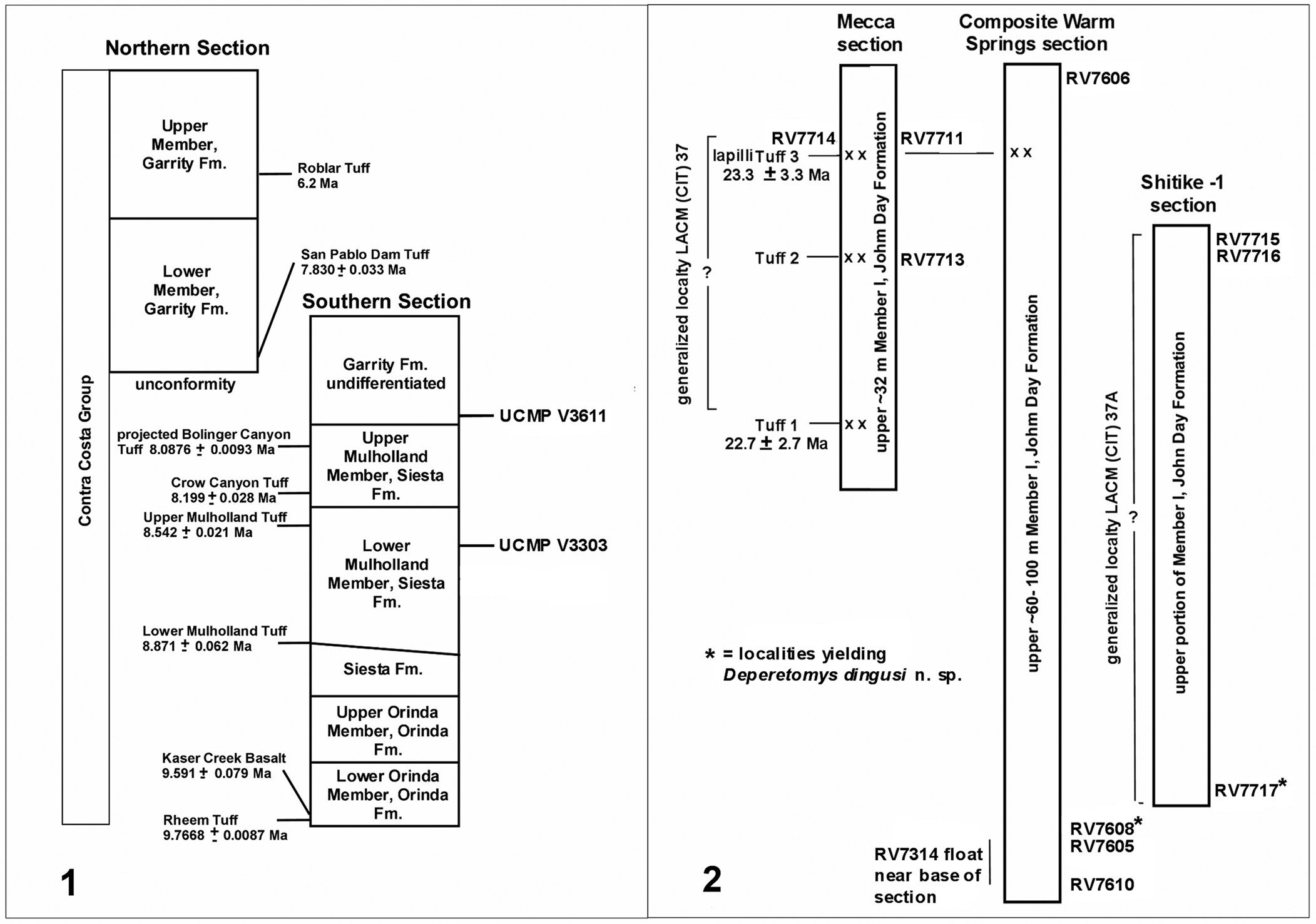
Figure 1. Generalized stratigraphic sections with radioisotopic dated tuffs of the Contra Costa Group (1) in the East San Francisco Bay area, California, and (2) the Warm Springs area, Oregon, showing the relative positions of the localities that yielded Pliotomodon primitivus and Deperetomys dingusi n. sp., respectively (modified from Dingus, Reference Dingus1978, Reference Dingus1990, and Wagner et al., Reference Wagner, Deino, Edwards, Sarna-Wojcicki, Wan, Sullivan, Sloan, Unruh and Schwartz2021).
40Ar/39Ar dates provide an age bracket (Wagner et al., Reference Wagner, Deino, Edwards, Sarna-Wojcicki, Wan, Sullivan, Sloan, Unruh and Schwartz2021) for the localities. UCMP V3611 occurs above the Crow Canyon Tuff (8.2 Ma) in the underlying lower part of the Upper Mulholland Member of the Siesta Formation and the Bolinger Canyon Tuff (8.1 Ma) in the Bolinger Member, which is laterally equivalent to the upper part of the Upper Mulholland Member in the vicinity of UCMP V3611. In the northern Garrity section, the lower Garrity member of the Garrity Formation unconformably overlies the Monterey Group with the San Pablo Dam Tuff (7.8 Ma) occurring ~80 m above the base of the member (Wagner et al., Reference Wagner, Deino, Edwards, Sarna-Wojcicki, Wan, Sullivan, Sloan, Unruh and Schwartz2021). UCMP V3303 occurs between the Upper Mulholland Tuff (8.2 Ma) and the Lower Mulholland Tuff (8.9 Ma) in the Lower Mulholland Member. Thus, an estimated age for UCMP V3611 is ca. 8.0–7.9 Ma and for UCMP V3303 is ca. 8.6–8.5 Ma, the total dated range of Pliotomodon. Additional rodents from UCMP V3611, two specimens of which were reported by Hoffmeister (Reference Hoffmeister1945) as Peromyscus sp., are currently under study and will be reported elsewhere. The material includes cricetids and geomyoids consistent with the Late Miocene age of the localities.
The Deperetomys specimens were recovered from two localities (Fig. 1.2): UCMP RV7717 at the base of the exposure of Member I in the Shitike-1 section and UCMP RV7608 from near the base of the exposure of Member I in the Warm Springs section (= WS-2 section of Dingus, Reference Dingus1990), John Day Formation, Wasco County, Oregon. Dingus (Reference Dingus1990) tentatively correlated an undated tuff at the top of WS-2 with Tuff 3 from the Mecca section. In the absence of radiometric dates for the sections with Deperetomys, a refined age estimation for UCMP RV7717 and UCMP RV7608 must be accomplished primarily via large-mammal biostratigraphy, with the Mecca dates providing a general chronological benchmark.
Mammals recorded collectively from the Warm Springs region (Dingus, Reference Dingus1990) localities include: Archaeolagus cf. A. macrocephalus (Matthew, Reference Matthew1907), Alphagaulus cf. A. vetus (Matthew, Reference Matthew1924), Entoptychus individens Rensberger, Reference Rensberger1971, ?Palaeocastor sp., Parahippus cf. P. leonensis Sellards, Reference Sellards1916, Parahippus pawniensis Osborn, Reference Osborn1918, Archaeohippus sp., Anchitherium sp., cf. Floridaceras whitei Wood, Reference Wood1964, Cynorca sociale (Marsh, Reference Marsh1875), Merycochoerus cf. M. matthewi Loomis, Reference Loomis1924, Merychyus cf. M. arenarum Cope, Reference Cope1884, Merchyus cf. M. calaminthus Jahns, Reference Jahns1940, Michenia deschutensis Dingus, Reference Dingus1990, Barbourmeryx submilleri (Frick, Reference Frick1937), cf. Hypertragulus sp., and Leptomerycini, genus undetermined. Dingus (Reference Dingus1990) regarded some of the large mammals from the Warm Springs localities as typical of the late Arikareean while others as typical of the early to late Hemingfordian. The K/Ar dates averaging ca. 23 Ma would place all of the localities within upper part of the late early (Ar3) Arikareean (Albright et al., Reference Albright, Woodburne, Fremd, Swisher III, MacFadden and Scott2008, fig. 11), but the large standard errors indicate a possible range of ca. 27.6–20 Ma (Ar3–Ar4).
Certain taxa in the large-mammal fauna are consistent with a late Arikareean age. Merycochoerus matthewi is known from the Johnson Canyon Member of the John Day Formation (Ar4) and the Anderson Ranch Formation (late Ar4) of western Nebraska (Albright et al., Reference Albright, Woodburne, Fremd, Swisher III, MacFadden and Scott2008). Merychyus calaminthus of the late Arikareean Tick Canyon Formation, California (Jahns, Reference Jahns1940), was reported from the Harrison Formation (Ar3 or Ar4) of western Nebraska (Lander and Lindsay, Reference Lander and Lindsay2011). Retallack and Samuels (Reference Retallack and Samuels2020) recognized overlapping Entoptychus individens and Merychyus arenarum zones in the Haystack–Balm Creek members of the John Day Formation (late Ar3). The first appearance datum for Cynorca sociale is in the Haystack Member (Ar3) of the John Day Formation, but it is also known from the early to late Hemingfordian of Texas and California (Woodburne, Reference Woodburne1969; Albright et al., Reference Albright, Woodburne, Fremd, Swisher III, MacFadden and Scott2008). Based on taxa with some stratigraphic control, the lower Warm Springs River localities with Deperetomys are likely late Arikareean, but whether they represent subchrons Ar3 or Ar4 cannot be determined.
Materials and methods
This study is based on direct examination of the Pliotomodon and Warm Springs cricetid specimens housed in the University of California, Berkeley collections and the Galushamys redingtonensis Jacobs, Reference Jacobs1977, specimens from the University of Arizona Laboratory of Paleontology collections. Numerous casts of fossil cricetid material provided by the late E. Lindsay (University of Arizona, Tucson) were also studied.
Cricetid dental terminology, which is provided in Figure 2, is based on Reig (Reference Reig1977), Kelly et al. (Reference Kelly, Martin and Ronez2020), and Martin et al. (Reference Martin, Peláez-Campomanes, Ronez, Barbière, Kelly, Lindsay, Baskin, Czaplewski and Pardiñas2020), and applied to Pliotomodon primitivus (Fig. 3). Whenever either a proto-mesolophule/id or mesoloph/id extends to the tooth border, the reentrant fold anterior to the structure is referred to as the mesoflexus/id, and the reentrant fold posterior to the structure as either the metaflexus (uppers) or entoflexid (lowers). In the absence of the proto-mesolophule/id or mesoloph/id, or when these structures are significantly reduced in length, the resulting single reentrant fold is referred to as the metaflexus (uppers) or entoflexid (lowers).

Figure 2. Cricetid dental occlusal terminology (based on Reig, Reference Reig1977; Kelly et al., Reference Kelly, Martin and Ronez2020; Martin et al., Reference Martin, Peláez-Campomanes, Ronez, Barbière, Kelly, Lindsay, Baskin, Czaplewski and Pardiñas2020).

Figure 3. Upper (left, holotype, UCMP 36030) and lower (right, UCMP 37535) molars of Pliotomodon primitivus Hoffmeister, Reference Hoffmeister1945.
Primary enamel crests associated with major cusps that wear to enamel channels filled with dentin are termed lophs (upper molars) and lophids (lower molars). Accessory projections from cusps are termed lophules and lophulids. Upper and lower molars are identified by upper- and lower-case letters and are numbered consecutively. L and R connote left and right when combined with molar designations; otherwise, L = length. Enamel rings with hollow centers are referred to as “atolls,” equal to the “pits,” “fossettes,” and “islands” of other authors. Prior to wear, the crown of cricetid occlusal structures is represented by a series of enamel ridges and seams that wear to the characteristic pattern of each species as dentin becomes exposed. Thus, the protocone and hypocone of M1 and the protoconid and hypoconid of m1 in ancestral cricetids typically express a curved chevron-shaped enamel ridge arranged in a generally anterior-posterior direction relative to the tooth midline that wears to a central cusp with two associated primary lophs or lophids, whereas the paracone and metacone on M1 and the metaconid and entoconid on m1 express a cusp plus a single primary loph or lophid generally directed medially. Lophs/ids may differ in their position (e.g., more anterior or posterior) in connection to other structures among taxa, and various accessory lophules/ids may appear and disappear within cricetid clades. Flexa/ids may turn anteriorly (provergent) or posteriorly (postvergent) or be generally horizontal with respect to the tooth midline. Other characteristic cricetid dental structures and terminology follow Martin and Zakrzewski (Reference Martin and Zakrzewski2019) and Kelly et al. (Reference Kelly, Martin and Ronez2020).
A lingual extension from the base of the protoconid on m1 that may connect with the metaconid in archaic cricetids such as Witenia and Deperetomys (de Bruijn et al., Reference de Bruijn, Marković, Wessels and van de Weerd2019) is considered in the Eurasian literature an extension of protolophid 2 (= posterior arm of the protoconid). However, examination of unworn and lightly worn m1s suggests the extension from the base of the protoconid is a distinct lophid, as can be seen (Fig. 4) in a small series of lower dentitions of Deperetomys hagni Fahlbusch, Reference Fahlbusch1964, from Petersbuch 48, Germany (Prieto, Reference Prieto2012) (this is especially obvious in the unworn m3 in Fig. 4.2, but also seems clear through examination of the other molars in Fig. 4).

Figure 4. (1–3) Three lower dentitions of Deperetomys hagni from the Middle Miocene of Petersbuch 48, Germany (from Prieto, Reference Prieto2012). Numbers with arrows reflect proposed homologies: 1 = protolophid 1; 2 = protoconid; 3 = protolophid 2; 4 = proto-mesolophulid, 5 = remnant mesolophid.
Only recently have we begun to appreciate the information that can be provided by examining unworn (essentially embryonic) patterns. A new occlusal terminology, ICAMER classification, has developed from examination of unworn molars (Barbière et al., Reference Barbière, Ronez, Ortiz, Martin and Pardiñas2019). In that terminology, the lingual extension from the base of the protoconid is labeled a proto-mesolophulid and a similar extension from the hypoconid is named the hypo-mesolophulid. Given this interpretation, it appears likely that the structure labeled in the cricetid literature as a mesolophid of most extinct and extant cricetid rodents in which the murid is absent or reduced, including many New World cricetids, is not homologous to the mesolophid of pappocricetodontines (e.g., Witenia; de Bruijn et al., Reference de Bruijn, Marković, Wessels and van de Weerd2019), eucricetodonines (e.g., Eucricetodon; Li et al., Reference Li, Meng and Wang2016; Leidymys; Korth, Reference Korth2010), or early cricetodontines such as the ancient species of Deperetomys (D. calefactus Marković et al., Reference Marković, de Bruijn, van de Weerd and Wessels2020, D. magnus de Bruijn, Marković, and Wessels, Reference de Bruijn, Marković and Wessels2013; Marković et al., Reference Marković, de Bruijn, van de Weerd and Wessels2020), in which both a proto-mesolophulid and mesolophid are observed.
Although size relations can be depicted through comparisons of dental or skeletal dimensions, in addition to providing raw measurement data we converted lower first molar (Lm1) length to body mass based on a revised equation from that of Martin (Reference Martin1996). Mass scales to a linear dental dimension in rodents at least as a cube power (Martin, Reference Martin1996; Martin et al., Reference Martin, Peláez-Campomanes and Mecklin2012, Reference Martin, Fox, Urevig, Dean, Rountrey and Peláez-Campomanes2021). Consequently, as Lm1 increases or decreases, mass changes disproportionately. As first summarized by Peters (Reference Peters1983), almost every important physiological and ecological variable in mammals is highly correlated with body mass (W in the literature; M is taken by metabolism). Since small changes in linear dimensions will result in large changes in mass, it seems both prudent and important to estimate the variable on which natural selection is most likely to operate. In short, generating estimates of average mass provides a more realistic biological context for size comparisons.
Body mass of Pliotomodon and other cricetids was estimated by an equation relating body mass to length of the first lower molar in extant New World cricetids. The equation was generated from 76 specimens representing 16 species (Supplementary Data 1). Length of the m1 and body mass data were taken from the same specimens housed in the U.S. National Museum in Washington, D.C. The formula generated is as follows:

Where W = mass in g and Lm1 = length of the m1 in mm. R2 = 0.88.
Scanning
All Pliotomodon specimens and the Deperetomys holotype mandible were scanned on a Phoenix Nanotom-M nanofocus X-ray computed tomography (CT) system in the Functional Anatomy and Vertebrate Evolution Laboratory at the University of California. Reconstructed slices were cropped in Fiji 1.53q (Schindelin et al., Reference Schindelin, Arganda-Carreras, Frise, Kaynig and Longair2012), rendered in three dimensions using Slicer 4.11 (Fedorov et al., Reference Fedorov, Beichel, Kalpathy-Cramer, Finet and Fillion-Robin2012) using the SlicerMorph extension (Rolfe et al., Reference Rolfe, Pieper, Porto, Diamond, Winchester, Shan, Kirveslahti, Boyer, Summers and Maga2021), segmented, and the segmentation exported as an stl file. The resultant STL was imported into Meshlab (Cignoni et al., Reference Cignoni, Callieri, Corsini, Dellepiane, Ganovelli, Ranzuglia, Scarano, De Chiara and Erra2008) and decimated to approximately one-third the number of original faces to produce smaller, web-viewable mesh files using their variant of quadric edge-collapse decimation (Garland and Heckbert, Reference Garland and Heckbert1997). Both the cropped CT-stack and mesh files were uploaded to MorphoSource.org (Table 1).
Table 1. Unique online identifiers for 3D scans and associated meshes at MorphoSource.org for specimens described here.

Phylogenetic analysis
To test the relationships of Pliotomodon and the Warm Springs cricetid to Old World cricetodontines, we performed a phylogenetic analysis based on the same 103 dental characters and character states that López-Guerrero (Reference López-Guerrero2014, appendix 10.1) utilized in her analyses of Old World cricetodontines. Because López-Guerrero's (Reference López-Guerrero2014) dental terminology for certain structures (e.g., lophs, lophids, lophules, and lophulids) differs from that used in our study, some descriptions of her characters and character states were modified to match our terminology (Supplementary Data 3). The resulting character state matrix is presented in Supplementary Data 4.
Following López-Guerrero (Reference López-Guerrero2014), Democricetodon franconicus Fahlbusch, Reference Fahlbusch1966, from the Calatavud-Daroca Basin of Spain was used for the outgroup. López-Guerrero's (Reference López-Guerrero2014) analysis included 53 ingroup taxa, only two of which were from Asia (Cricetodon volkeri Wu et al., Reference Wu, Meng, Jie, Ni, Bi and Wei2009, C. wanhei Qiu, Reference Qiu2010). In our analysis, we included all of her taxa plus three additional Asian taxa (C. fengi Qiu and Li, Reference Qiu and Li2016, C. sonidensis Qiu and Li, Reference Qiu and Li2016, and Gobicricetodon). Gobicricetodon flynni Qiu, Reference Qiu1996, was selected to represent the genus because it is the type species and has the most complete hypodigm with all tooth positions represented. With the addition of C. sonidensis and C. fengi, our analysis includes all currently recognized Asian species of Cricetodon.
The analysis using parsimony was performed with the TNT program of the Willi Hennig Society (Goloboff et al., Reference Goloboff, Farris and Nixon2008; Goloboff and Catalano, Reference Goloboff and Catalano2016) using the new technology search algorithm. All character states were treated as unordered (non-additive) to avoid biases that might be introduced due to subjective ordering of character state transformations. Branch support was determined using bootstrap resampling (10,000 replicates with a ≥50% cutoff).
Repositories and institutional abbreviations
Specimens examined or reported are located in the following institutions: UALP, University of Arizona, Paleontology Lab; UCMP, University of California, Berkeley, Museum of Paleontology; UCMP RV, designation for localities originally part of University of California-Riverside collections, transferred to UCMP; FAM, Frick Collection, American Museum of Natural History; UF, University of Florida, Florida State Museum; LACM (CIT), California Institute of Technology specimens/localities transferred to the Natural History Museum of Los Angeles County.
Systematic paleontology
Pliotomodon primitivus is a large cricetid rodent demonstrating an occlusal morphology of upper and lower molars most similar to that of Eurasian cricetodontines such as Hispanomys and Byzantinia, with superficial similarities to North American Galushamys, Miotomodon, and Repomys. Characteristic features of Pliotomodon are seen in the connection of the paracone to the metacone in the upper molars and the deep flexids on the lowers, resulting in oblique orientation of the major cusps and long connections of the cusps and their associated lophids in the latter. In addition to the paracone-metacone connection, enamel bridges from the procingulum on M1 and anterior cingula on M2-M3 close the paraflexus as well as the metaflexus, resulting in two enamel atolls surrounding the spaces representing the ancestral (open) paraflexus and metaflexus and a selenodont-like pattern seen in a number of rodent clades, particularly the Old World cricetodontines (Marković et al., Reference Marković, de Bruijn, van de Weerd and Wessels2020). Atolls also form in the lower dentition (Fig. 3). The metaconid on m1 is widely confluent with the procingulid on m1, and combined with the deep, highly provergent entoflexid, results in a characteristic elongate form of the procingulid-metaconid combination, seen in many Old World cricetodontines. Because the paracone and metacone are also fused in the M1 of Galushamys and the molars tend to be hypsodont and planed, we initially considered Galushamys and Pliotomodon to be closely related. However, after careful comparison we have concluded the similarities between Galushamys and Pliotomodon to be the result of parallelism, which is discussed more fully in the sections below. In the following section we begin our analysis by providing a detailed description of Pliotomodon primitivus.
Order Rodentia Bowdich, Reference Bowdich1821
Family Cricetidae Fischer von Waldheim, Reference Fischer von Waldheim1817
Subfamily ?Cricetodontinae Stehlin and Schaub, Reference Stehlin and Schaub1951
Genus Pliotomodon Hoffmeister, Reference Hoffmeister1945
Type species
Pliotomodon primitivus Hoffmeister, Reference Hoffmeister1945. Late Miocene (Hemphillian), California.
Pliotomodon primitivus Hoffmeister, Reference Hoffmeister1945
Figures 3, 5.1, 6, 7.1
Holotype
UCMP 36030, partial left maxilla with M1–M3.

Figure 5. Lower and upper dentitions of Pliotomodon primitivus and Old World cricetodontines. (1) P. primitivus (this study), (2) Hispanomys moralesi (from López-Antoñanzas et al., Reference López-Antoñanzas, Peláez-Campomanes, Angeles Álvarez Sierra and García-Paredes2010), (3) H. decedens (Schaub, Reference Schaub1925) (from López-Antoñanzas and Mein, Reference López-Antoñanzas and Mein2011), (4) Deperetomys hagni (from Marković et al., Reference Marković, de Bruijn, van de Weerd and Wessels2020), (5) Cricetodon sansaniensis (from López-Guerrero, Reference López-Guerrero2014), (6) C. nievei (from López-Guerrero et al., Reference López-Guerrero, Ángeles Álvarez Sierra, García-Paredes and Peláez-Campomanes2014), (7) Byzantinia pikermiensis de Bruijn, Reference de Bruijn1976 (from Ünay et al., Reference Ünay, de Bruijn and Suata-Alpaslan2006), (8) Eumyarion bifidus Fahlbusch, Reference Fahlbusch1964 (from de Bruijn, Reference de Bruijn2009), (9) Gobicricetodon arshanensis Qiu and Li, Reference Qiu and Li2016 (from Qiu and Li, Reference Qiu and Li2016). Illustrations not to scale.

Figure 6. Micro CT cross-sectional scans of Pliotomodon primitivus (1) holotype maxilla UCMP 36030 and (2) mandible UCMP 37535 showing number and position of roots.

Figure 7. Comparison of (1) Pliotomodon primitivus molars with those of (2) Miotomodon mayi Korth, Reference Korth2011 (from Korth, Reference Korth2011), (3) Repomys gustelyi May, Reference May1981 (from May, Reference May1981), (4) Galushamys redingtonensis (this study; see Fig. 8 for specimen numbers), and (5) Scotinomys teguina (Alston, Reference Alston1876) (UF 31150, Monte Verde, Costa Rica). Illustrations not to scale.

Figure 8. New photographs of molars of Galushamys redingtonensis from the Redington assemblage, Arizona. (1) FAM 98958, holotype RM1-M2 (reversed); (2) UALP 6013, RM1 (reversed); (3) UALP 6015 RM1; (4) UALP 6015, RM1, labial view (reversed); (5) UALP 6014 RM1, labial view (reversed); (6) UALP 6014, RM1 (reversed); (7) UALP 6016, RM2 (reversed); (8) UALP 6017, LM2; (9) UALP 6018, LM2; (10) UALP 6019, LM2 (reversed); (11) UALP 6020, LM3; (12) UALP 6020, LM3, lingual view; (13) UALP 6021, partial Rm1 (reversed); (14) UALP 6022, Lm2. Scale = 1 mm.
Paratypes
From Loc. V3611. UCMP 37632, partial right maxilla with worn M1 and partial M2; UCMP 37633, partial right maxilla with partial M1 and M2; UCMP 37534, isolated partial Rm2.
Emended diagnosis
Large cricetid rodent (LM1 and Lm1 > 3.0 mm; estimated mass >200 g) with planed, hypsodont molars. Dentine channels connect all major cusps after moderate wear. Anterolophule and paralophule on M1-M2 close paraflexus and metaflexus, respectively, creating internal enamel atolls. Deep anteromedian fissure on M1 defines two anteroconules. Mesoloph absent on upper molars, proto-mesolophulid reduced on lowers. Hypoflexus, metaflexus, and posteroflexus on M3 enclosed by enamel bridges, creating three atolls. Hypoflexus and paraflexus confluent on M3. Metalophid and entolophid on m1 directed anteriorly. Entoflexid on m1 highly provergent. Metalophid on m1 confluent with procingulid; entolophid on m1 confluent with base of hypolophid 1 and protolophid 2. Mesoflexid, entoflexid, and posteroflexid on m3 enclosed by enamel bridges. M1–M3 with 3 roots; m1–m3 with 2 roots. Pliotomodon primitivus differs from all large, hypsodont cricetodontines (Hispanomys, Byzantinia, Ruscinomys) in hypoflexus, metaflexus, and posteroflexus on M3 enclosed by enamel bridges; mesoflexid, entoflexid, and posteroflexid on m3 closed by enamel bridges and retaining primitive number of 3 roots on M1, rather than 4–5 in hypsodont cricetodontines.
Occurrence
Mulholland site 1, UCMP Loc. V3303; Mulholland site 2, CA, UCMP Loc. V3611 (type locality) (ca. 8.6–7.9 Ma. Hemphillian; Hh-1).
Description
The following descriptions are based on the holotype upper dentition (UCMP 36030) and lower dentition, UCMP 37535 (Fig. 5) and reflect updated dental nomenclature. Neither the maxillary nor dentary bones retain any taxonomically useful features.
M1
The M1 is moderately worn and dentine channels connect most major cusps by various lophs. The procingulum includes labial and lingual conules of approximately equal size. An anteromedian fissure separates the conules. A labial anterolophule abuts the lingual anteroconule with the paracone but does not connect by a dentine channel. The protocone is large and triangular-shaped. Protoloph 1 connects to the lingual anteroconule; protoloph 2 connects to the paraloph and anterior end of hypoloph 1. The hypocone is also large, and hypoloph 1 connects to the base of the paraloph and base of protoloph 2. Hypoloph 2 is widely confluent with a fused metaloph/posteroloph dentine field. The mesoloph is considered to be absent; the dentine channel lingual to the base of the paracone is considered to be an extension of the paraloph, as is seen in Cricetodon nievei López-Guerrero et al., Reference López-Guerrero, Ángeles Álvarez Sierra, García-Paredes and Peláez-Campomanes2014, and other cricetodontines in which the mesoloph is absent (Fig. 5). A distinct posteroloph is absent, as it is in other dentally derived cricetodontines (Fig. 5). A posterior paralophule extends from the base of the paraloph to the metacone. The paralophule connects widely with both the paraloph and the lingual end of the metacone. Two enamel atolls are created on the occlusal surface by closure of the paraflexus and metaflexus. The enamel is thick and relatively undifferentiated. The M1 has three roots (Fig. 6).
M2
The anterior end of M2 is composed of an anterior cingulum and two slight conules that may represent part of the undeveloped M1 procingulum region. From this point posteriorly, the M2 is identical to M1 with the exception of a very small lophule at the lingual base of hypoloph 1 that could be a vestigial mesoloph. The M2 has three roots.
M3
The M3 is reduced relative to M2 but still relatively large as compared with the M3 of many other cricetids. The most difficult region to interpret is the area including the base of the paraloph and its connection to the hypocone. We interpret this area to represent either a hybrid structure composed of the base of the paraloph and the mesoloph or just part of the paraloph. Instead of connecting with both the mesoloph and hypoloph 1, protoloph 1 connects only with hypoloph 1. An unusual feature of the M3, which separates it from almost all other cricetids, is the absence of an external hypoflexus. The internal extension of the hypoflexus may have been captured by the paraflexus, suggesting the anterior atoll of M3 is not entirely homologous to that of M2. A third atoll is created on M3 by a reduction in size of the metacone and an anterior shift of the metaloph, connecting at the base of a reduced hypoloph 2. The maxilla is broken posterior to M3, and although only two alveoli are observable from a ventral perspective (Fig. 6), a third posterolabial root is present, broken at the base of the crown.
m1
The procingulid of m1 was likely relatively small when unworn, composed of a single conulid, and dentine channels connect broadly to both the metaconid by an anteriorly directed metalophid, and to the protoconid by protolophid 1. The metaflexid is shallow. The entoflexid is wide at the lingual tooth border but forms a narrow, provergent form as it extends beneath the lingual border of the protoconid. The entolophid is directed anteriorly and joins the base of protolophid 2 and hypolophid 1. A small, lingually directed lophulid likely represents the vestiges of a proto-mesolophulid. The posteroflexid is wide and deep, with an anterior extension defined by the posterior enamel border of the entoconid. The hypoflexid is deep, slightly provergent, and extends beyond the tooth midline. Hypolophid 1 is relatively long and joins the base of the entolophid. Hypolophid 2 also widely joins the posterolophid. The m1 has two roots.
m2
The anterior lingual region of m2 is broken. A small protoflexid is observable. The occlusal morphology of m2 is similar to that of m1 posterior to the procingulid, with the exception of a small enamel atoll at the confluence of protolophid 2, the entolophid, and hypolophid 1. A lightly defined atoll is present between the hypoconid and the posterolophid. The posteroflexid is narrow and provergent. The m2 has two roots.
m3
This molar is relatively large; the ratio of m3/m2 length = 0.78. A small atoll, likely representing an isolated metaflexid, is present in the center of an anterior cingulid. The metaconid is small, connecting anteriorly with the anterior cingulid. A lophid extending labially from protolophid 2 is considered to be the retention of a proto-mesolophulid. The mesoflexid is closed lingually, forming an atoll anterior to the proto-mesolophulid (this is best seen in the scan of Fig. 5.1). Likewise, a small atoll forms anterior to the entolophid, because the entoflexid is also closed by an enamel bridge. The entolophid is short, connecting hypolophid 1 and a reduced entoconid. The posteroflexid is small and closed lingually. The m3 has two roots.
Referred material
From Mullholland site 1, UCMP Loc. V3303 (ca. 8.6–8.5 Ma. Hemphillian; Hh-1); UCMP 37535, part left mandible with m1–m3.
Measurements
Measurements of Pliotomodon primitivus specimens (length, width in mm): UCMP 36030 (holotype): M1 (3.67, 2.04), M2 (2.73, 1.91), M3 (2.05, 1.65); UCMP 37632 (paratype): M1 (3.62, 2.09), M2 (—, 2.06); UCMP 37633 (paratype): M1 (—, 2.16), M2 (2.61, 2.01); UCMP 37535: m1 (3.01, 1.85), m2 (2.67, 2.01), m3 (2.50, 1.71); UCMP 37634 (paratype): m2 (—, 2.02).
Remarks
Supplementary Data 2 provides a comparison of the M1 and m1 lengths and body mass estimates in a number of cricetids and cricetodontines. Pliotomodon primitivus at 209 g was equal in size to large Old World cricetodontines such as Cricetodon albanensis Mein and Freudenthal, Reference Mein and Freudenthal1971 (192 g), and in North America would have been the largest cricetid on the continent during the Late Miocene. Within the New World cricetids, only the derived, hypsodont woodrats, Neotoma, which did not appear until the Blancan, would eventually become larger.
The dentition of Pliotomodon primitivus is compared with a variety of Eurasian cricetodontines (Fig. 5). Pliotomodon shares with the more derived cricetodontines such as Hispanomys, Byzantinia, and later Cricetodon, the following features: (1) relatively large size (average M1 length >3.0 mm), (2) unreduced m3/M3, (3) loss or reduction of mesolophid/mesoloph (except in some Byzantinia), (4) metalophid on m1 widely confluent with procingulid (except some Cricetodon species), (5) bilobed procingulum on M1, and (6) well-developed labial anterolophule and paralophule on M1 (anterolophule may connect with paracone and paralophule may connect with metacone, isolating two enamel atolls). Despite these similarities, Pliotomodon expresses unique traits that indicate it evolved in isolation from the known advanced cricetodontines, as follows: (1) reduction of the metaflexid and metaconid and almost complete fusion of the metaconid and procingulid on m1, (2) closure of both the entoflexid and posteroflexid on m3, (3) closure of the hypoflexus and posteroflexus on M3, and (4) three roots on M1–M3.
Shared size and dental morphology of Pliotomodon suggest that P. primitivus is a cricetodontine rodent, originating from an Asian ancestor during the Late Miocene (8–9 Ma). No western North American late Neogene sediments have produced a Cricetodon-like species, but cricetodontines in Europe and Anatolia at that time include Byzantinia, Cricetodon, Hispanomys, and, if considered a cricetodontine, Eumyarion (Supplementary Data 2). Asian Miocene cricetodontines include Cricetodon and Gobicricetodon (Qiu, Reference Qiu1996; Sen and Erbajeva, Reference Sen and Erbajeva2011; Qiu and Li, Reference Qiu and Li2016). Highly derived cricetodontines with selenodont-like dental morphology apparently evolved from one or more Cricetodon species with a more generalized pattern, with a tendency towards hypsodonty and closure of the paraflexus and metaflexus of M1-M2 by a posterolabially extended anterolophule and paralophule. Derived cricetodontines with a selenodont-like pattern, such as Hispanomys, Byzantinia, and Ruscinomys, added accessory roots to M1-M2, expressing either 4 or 5 roots on these molars. Archaic Cricetodon species, such as C. fikreti Çinar Durgut and Ünay, Reference Çinar Durgut and Ünay2016, C. kasapligili de Bruijn et al., Reference de Bruijn, Fahlbusch, Saraç and Ünay1993, and C. versteegi de Bruijn et al., Reference de Bruijn, Fahlbusch, Saraç and Ünay1993, retain the ancestral 3 roots on M1-M2 (Çinar Durgut and Ünay, Reference Çinar Durgut and Ünay2016).
The dentition of Pliotomodon primitivus is compared with a variety of North American extinct and extant taxa demonstrating somewhat similar occlusal morphology in Figure 7. New photos of Galushamys redingtonensis are provided in Figure 8. All of the latter taxa are currently classified in the Galushamyina, a subtribe of the neotominin neotomines (Martin and Zakrzewski, Reference Martin and Zakrzewski2019). Taxa illustrated share the following characters: (1) molars planed and mesodont to hypsodont, (2) metalophid confluent with procingulid on m1 after moderate wear, (3) protolophid 2 and entolophid confluent on m1 after moderate wear, (4) entoflexid on m1 deeply provergent, (5) vestigial proto-mesolophulid often present, and (6) labial anterolophule or paralophule often developed on M1 (connects procingulum with paracone and paracone with metacone in Scotinomys). Despite these similarities, which are shared also with Eurasian cricetodontines, significant differences from Pliotomodon include: (1) small size (there is no overlap in either m1 or M1 measurements between the galushamyinans and P. primitivus); (2) the m3 and M3 of the galushamyinans are significantly reduced in size from those of P. primitivus and their presumed ancestor, Protorepomys (Fig. 8); and (3) with the exception of Scotinomys, the anterolophule and paralophule on upper molars do not isolate the paraflexus and metaflexus into internal atolls. Scotinomys has been genetically linked to Baiomys in the neotomine tribe Baiomyini (Miller and Engstrom, Reference Miller and Engstrom2008; Keith, Reference Keith2015; Steppan and Schenk, Reference Steppan and Schenk2017), and the Scotinomys dentition was likely derived from the more general Baiomys morphology. We conclude from these observations that the Galushamyina collectively are smaller neotomine rodents that evolved in North America and are related to Miocene taxa such as Lindsaymys, Tsaphanomys, and Protorepomys (Kelly and Martin, Reference Kelly and Martin2022), and are unrelated to Pliotomodon.
Order Rodentia Bowditch, Reference Bowdich1821
Family Cricetidae Fischer von Waldheim, Reference Fischer von Waldheim1817
Subfamily Cricetodontinae Stehlin and Schaub, Reference Stehlin and Schaub1951
Genus Deperetomys Mein and Freudenthal, Reference Mein and Freudenthal1971
Type species
Deperetomys hagni, Kleineisenbach, Germany. Early Miocene (MN7/8).
Holotype
UCMP 316883 (formerly UCR 16883), partial left mandible with I, m1–m3.

Figure 9. Lower dentition and mandible of UCMP 316883, holotype partial right mandible with m1–m3 of Deperetomys dingusi n. sp. (1) Occlusal view, (2) labial view (reversed), (3) lingual view, (4) ventral view of diastemal region and incisor; note one central and two labial ridges, (5) UCMP 316525, paratype Lm3.

Figure 10. Comparison of dental structures between archaic (D. calefactus, D. magnus) and derived (D. hagni, D. dingusi n. sp.) Deperetomys species. 1 = proto-mesolophulid, 2 = mesolophid, 3 = entolophid, 4 = central murid. Note rotation of entolophid base from hypolophid 1 in D. calefactus and D. magnus to the proto-mesolophulid in D. hagni. Position of entolophid in D. dingusi n. sp. is intermediate. Deperetomys calefactus and D. magnus from Marković et al. (Reference Marković, de Bruijn, van de Weerd and Wessels2020); D. hagni from Prieto (Reference Prieto2012); D. dingusi n. sp., this study. Photos not to scale.
Paratype
UCMP 316525, Lm3.
Diagnosis
Medium-sized Deperetomys without mesolophid. Proto-mesolophulid on m1 long, curves anteriorly and fuses with metaconid on m1-m2. Ectomesolophid well developed on m1-m2, reduced on m3. Entolophid connects to base of hypolophid 1 rather than to proto-mesolophulid in m1–m3. Posterior half of m3 narrow, reduced in size; posteroflexid wide and open. Differs from D. calefactus, D. saltensis Marković et al., 2019, and D. magnus in the absence of a true mesolophid on lower molars. Differs from D. anatolicus de Bruijn et al., Reference de Bruijn, Fahlbusch, Saraç and Ünay1993, D. intermedius de Bruijn et al., Reference de Bruijn, Fahlbusch, Saraç and Ünay1993, and D. hagni in that the entolophid does not fuse with the proto-mesolophulid. Further differs from D. hagni in the separation of protolophid 1 from the procingulid; connected in D. hagni. The narrow configuration of m3 in D. dingusi n. sp., in which the posterolophid does not connect strongly to the entoconid, is also unique among Deperetomys species.
Occurrence
UCMP Locs. RV7608 and RV7717 (type locality), Warm Springs region of John Day Formation, Wasco Co., Oregon, late Oligocene or early Miocene (ca. 23 Ma. Arikareean; Ar3 or Ar4).
Description
The distinguishing generic characteristics of Deperetomys as provided by Marković et al. (Reference Marković, de Bruijn, van de Weerd and Wessels2020) are observed on the lower dentition of D. dingusi n. sp. (Figs. 9, 10), and are described in detail below.
Mandible
The mandible is broken posterior to m3. From labial aspect, the anterior and posterior masseteric scars meet in a small anterior masseteric crest (amc) under m1. The mental foramen is located on the labial side of the diastema, basically in line with the amc.
Incisor
The incisor is broad and displays three ridges; a single ventral ridge and two closely aligned ventrolabial ridges.
m1
The procingulid is rounded with a single main cusp and a small, lingual anteroconulid. The metalophid connects anteriorly with the procingulid. The proto-mesolophulid extends lingually and anteriorly to fuse with the metaconid. The mesolophid is absent. An ectomesolophid extending to the labial tooth border is developed between the protoconid and hypoconid. The entolophid connects with the base of protolophid 1. A round posterolophid extends posteriorly from the posterior end of m1. The entoflexid and posteroflexid are deep and provergent. The cusps are alternate. The m1 has two roots.
m2
The m2 is basically a replicate of m1. An anterior cingulid replaces the procingulid, forming a well-developed protoflexid. The m2 has two roots.
m3
The m3 (Fig. 9.1, 9.5) is large and, as compared with m2, narrows posteriorly. Protolophid 2 is obliterated in the entoflexid. As in m1-m2, the entolophid connects with the base of hypolophid 1. The posteroflexid is wide and the enamel of the posterolophid does not connect with the entoconid as in m2. The m3 has two roots.
Etymology
For Lowell Dingus, who recovered and described the Warm Springs cricetid, and first recognized its relationship to Old World taxa.
Measurements
Measurements of specimens (length, width in mm): UCMP 316883 (holotype): m1 (length, width in mm) (2.62, 1.73), m2 (2.29, 1.87), m3 (2.39, 1.80); UCMP 316525 (paratype): m3 (2.25, 1.78).
Remarks
The most recent evaluation of Deperetomys dental morphology and taxonomy was provided by Marković et al. (Reference Marković, de Bruijn, van de Weerd and Wessels2020). They emended the generic diagnosis of Mein and Freudenthal (Reference Mein and Freudenthal1971) and de Bruijn et al. (Reference de Bruijn, Fahlbusch, Saraç and Ünay1993). Because we lack upper molars of the Warm Springs cricetid, we refer only to the section of the diagnosis applying to the lower dentition, as follows (Marković et al., Reference Marković, de Bruijn, van de Weerd and Wessels2020, p. 833): “The m3 and m2 are about equally long. The m1 is rounded anteriorly. Anteroconid blade-shaped with a small cusp. The posterior arm of the protoconid of the lower cheek teeth is usually long and may connect with the metaconid. The m1 and/or m2 of the Oligocene representatives may have a short mesolophid and/or posterior arm of the hypoconid, in the Miocene species these elements are fused.” As noted above, in our terminology the posterior arms of the protoconid and hypoconid are referred to as protolophid 2 and hypolophid 2 (Fig. 2).
In the chronology illustrated by Marković et al. (Reference Marković, de Bruijn, van de Weerd and Wessels2020), the earliest known Deperetomys appeared during the Oligocene, ca. 34–33 Ma (MP21–22). The earliest named species, D. calefactus, is encountered between 27–25 Ma (MP27–28). Dentally derived species appear soon after, between ca. 25–23 Ma (MP29–MN1). Deperetomys hagni is recorded much later, ca. 12 Ma (MN7/8). The Warm Springs biota of the John Day Formation is considered to have existed ca. 23 Ma (Dingus, Reference Dingus1990). Based on occlusal pattern, D. dingusi n. sp. combines both ancestral and derived characters, suggesting it represents a lineage distinct from other derived species such as D. hagni, D. intermedius, and D. anatolicus. In archaic species, represented by D. calefactus, D. saltensis, and D. magnus, the proto-mesolophulid most often does not fuse with the metaconid, a small mesolophid may be present, and the entolophid fuses with a central murid posterior to the mesolophid at the anterior base of hypolophid 1 (Figs. 9, 10). A distinct ectomesolophid is seen only in D. magnus and some m1s of D. saltensis. In derived Deperetomys, including D. intermedius, D. anatolicus, and D. hagni, the entolophid has rotated anteriorly, fusing with the proto-mesolophulid. In D. dingusi n. sp. the proto-mesolophulid connects with the metaconid (derived), and the entolophid is more anterior in position than in D. calefactus, D. saltensis, and D. magnus, but it does not connect with the proto-mesolophulid (intermediate). An ectomesolophid is present (underived). The posterior half of the m3 is narrow and the posteroflexid is wide and open (derived).
Dingus (Reference Dingus1978) found considerable similarity of the Warm Springs cricetid to Eumyarion, a genus with a complex taxonomic history that will not be examined in detail here (see de Bruijn, Reference de Bruijn2009; Marković et al., Reference Marković, de Bruijn, van de Weerd and Wessels2020). Eumyarion is similar in dental morphology to a number of early cricetids, including Deperetomys, but also demonstrates sufficient differences from all of them that Ünay-Bayraktar (Reference Ünay-Bayraktar1989) classified Eumyarion in the monotypic subfamily Eumyarioninae. The m1–m3 of Eumyarion is similar in morphology to the earliest Deperetomys, such as D. calefactus, in possessing both a true mesolophid and a proto-mesolophulid that does not connect with the metaconid, but differs from D. dingusi n. sp., in which the mesolophid is absent and the proto-mesolophulid strongly connects with the metaconid. The metaconid, entoconid and posterior cingulid of m3 in Eumyarion are usually connected by enamel ridges that close off the posteroflexid and entoflexid; these flexids are widely open in D. dingusi n. sp. The m3 of Eumyarion is reduced in size relative to m2; in D. dingusi n. sp. the m3 is slightly longer than m2.
Results of phylogenetic analysis
The TNT analysis resulted in a single most parsimonious tree of 843 steps with a Consistency Index (CI) of 0.180 and Retention Index (RI) of 0.582 (Fig. 11; see Supplementary Data 5 for lists of terminal taxa apomorphies and node synapomorphies). The analysis placed the Warm Springs cricetid securely nested within the Deperetomys clade, supporting its referral to the genus. Eleven species of Hispanomys plus Cricetodon klariankae Hir, Reference Hir2007, were placed as successive sister taxa to Pliotomodon primitivus and it was placed as the closest sister clade to a Ruscinomys-Hispanomys adroveri Agustí, Reference Agustí1986, clade. The recognition of a Byzantinia clade separate from a Hispanomys clade was also found in the analyses of López-Guerrero (Reference López-Guerrero2014) and López-Antoñanza and Peláez-Campomanes (Reference López-Antoñanzas and Peláez-Campomanes2022).

Figure 11. Single most parsimonious tree of 843 steps using new technology search algorithm of TNT program, CI = 0.180, RI = 0.582. Values below branches are bootstrap support when <50%. See Supplementary Data 5 for list of apomorphies for terminal taxa and node synapomorphies.
In the analysis of López-Guerrero (Reference López-Guerrero2014) and our similar results, Hispanomys adroveri is nested within a Ruscinomys clade, resulting in Ruscinomys as paraphyletic. However, Agustí et al. (Reference Agustí, Garcés and Krijgsman2006, p. 8) noted a possible relationship of H. adroveri to Ruscinomys, stating “… in situ evolution of the first Ruscinomys species from middle Turolian H. adroveri cannot be excluded.” Van Dam et al. (Reference van Dam, Furió and van Balen2014) also regarded H. adroveri from La Celia as related to Ruscinomys schaubi Viilalta and Crusafont Pairó, Reference Villalta and Crusafont Pairó1956. Hispanomys adroveri (van de Weerd, Reference van de Weerd1976; Agustí, Reference Agustí1986; Freudenthal et al., Reference Freudenthal, Lacomba and Martín Suárez1991; López-Guerrero, Reference López-Guerrero2014) shares a number of dental characters with Ruscinomys, including: (1) large size (M1 L = 3.6–3.8 mm for topotypic H. adroveri); (2) selenodonty; (3) hypsodonty; (4) width of M1-2 narrowed relative to their length; (5) M1 5-rooted; (6) M1 protoflexus open; (7) M3 reduced relative to M1-2 and lacking anterior cingulum; (8) m1 lacking labial projection from the procingulid (= anterolophid of van de Weerd, Reference van de Weerd1976) and ectomesolophid; (9) m1 procingulid reduced and labially positioned; and (10) m3 lacking anterolabial cingulid and protoflexid. Additional characters seen in Ruscinomys and in certain derived Hispanomys (e.g., H. moralesi López-Antoñanzas et al., Reference López-Antoñanzas, Peláez-Campomanes, Angeles Álvarez Sierra and García-Paredes2010), are complete labial connections on M1-M2 and oblique rotation of the paraloph and metaloph. Although these shared characters strongly suggest that H. adroveri could be transferred to Ruscinomys, a comprehensive analysis including all species of Hispanomys and Ruscinomys is needed to confirm this proposal.
Despite Pliotomodon being well nested within the derived cricetodontines in the analysis, its proposed phylogenetic placement requires further discussion. Pliotomodon primitivus exhibits the following M1 character states that are also seen in derived cricetodontines, such as Hispanomys and Ruscinomys: (1) large size; (2) hypsodonty; (3) a complete labial connection of the procingulum and paracone via the labial anterolophule, resulting in a paraflexus atoll; (4) a complete labial connection of the paracone and metacone via the posterolabial paralophule, resulting in a metaflexus atoll; and (5) oblique rotation of the paraloph and metaloph. However, Pliotomodon differs from all other derived cricetodontines by having a 3-rooted M1, which is the ancestral number seen in archaic species of Cricetodon (e.g., C. kasapligili, C. versteegi, C. tobieni de Bruijn et al., Reference de Bruijn, Fahlbusch, Saraç and Ünay1993, C. volkeri, C. fengi, C. sonidensis). All dentally derived cricetodontines included in the analysis have 4–5 roots on M1. This fact suggests that either (1) M1 root count is reversible or (2) there are unknown Asian or Eurasian cricetodontine lineages that retained three roots on M1 as they increased in hypsodonty and developed a derived dental morphology. There is no evidence in the fossil muroid rodent literature documenting that trends in increased dental root count can reverse direction (e.g., Martin, Reference Martin1979; López-Guerrero, Reference López-Guerrero2014), consequently the second option seems more likely. Additionally, as noted in the description above, the M3 and m3 of Pliotomodon express unique characters not seen in other cricetodontines. As autapomorphies, these features contain no useful phylogenetic information except, in our interpretation, they represent derived conditions compared to Hispanomys, Ruscinomys, and Byzantinia. There are no large cricetodontine-like potential ancestral muroids in North America for Pliotomodon, and we assume it (or an ancestral species) must have dispersed across Beringia. This dispersal pattern implies a lengthy ancestral history in eastern Asia, or perhaps initially in Asia Minor. Currently there are no recognized advanced cricetodontines known from Asia beyond the archaic Cricetodon-like dental grade (i.e., C. wanhei, C. fengi, C. sonidensis, C. volkeri, Gobicricetodon). Given that there is a long history in Europe and Anatolia of muroids with both the Pliotomodon occlusal pattern and size, and an absence of such potential ancestors in North America, we interpret the features of P. primitivus to indicate that it likely originated in Asia from a currently unknown Cricetodon-like taxon with three roots on M1 and derived M3/m3 characters that was not ancestral to later advanced cricetodontines (Hispanomys, Byzantinia, Ruscinomys). In this phylogenetic scenario, the derived dental similarities of Pliotomodon to the later advanced cricetodontines represent parallel evolution, and the allocation of Pliotomodon to the Cricetodontinae remains tentative.
Discussion
Above, we concluded that two late Neogene North American muroid rodents characterized by previous taxonomic uncertainty represent separate dispersals of cricetodontine-like muroids across Beringia. In order to complete an understanding of the relationships of Pliotomodon primitivus and Deperetomys dingusi n. sp., it is necessary to place them in a modern taxonomic context of other extinct North American cricetid and cricetid-like muroids. Recent workers (e.g., Lindsay, Reference Lindsay, Janis, Gunnell and Uhen2008; Steppan and Scheck, Reference Steppan and Schenk2017; Ronez et al., Reference Ronez, Martin and Pardiñas2020) recognized the New World cricetids and their Old World relatives as the Cricetidae, a usage we follow here, but different classification schema within the family have been proposed as new molecular phylogenies and paleontological information have been published.
The latest attempt at classifying extinct North American cricetids was by Lindsay (Reference Lindsay, Janis, Gunnell and Uhen2008). Martin and Zakrzewski (Reference Martin and Zakrzewski2019) redefined Lindsay's (Reference Lindsay, Janis, Gunnell and Uhen2008) Galushamyini, restricting it to include the genera Galushamys, Protorepomys, Repomys, Miotomodon, and Nelsonia. This reconstituted grouping was referred by Martin and Zakrzewski (Reference Martin and Zakrzewski2019) to the neotomine tribe Neotomini as the subtribe Galushamyina, balanced by the subtribe Neotomina, including Tsaphanomys, Neotoma, Hodomys, and Xenomys. Microtoscoptes and Goniodontomys, included in Lindsay's (Reference Lindsay, Janis, Gunnell and Uhen2008) Galushamyini, are now considered “microtoid-like” cricetids of uncertain ancestry by Fejfar et al. (Reference Fejfar, Heinrich, Kordos and Maul2011). Paronychomys and Basirepomys recently have been shown to be ancient sister groups to the Neotomini, unrelated to either Onychomys or Repomys (Kelly and Martin, Reference Kelly and Martin2022), and Onychomys and its extinct relative Acrolophomys are now recognized as the tribe Onychomyini (Kelly et al., Reference Kelly, Martin, Ronez, Canon and Pardiñas2022). Lindsay (Reference Lindsay, Janis, Gunnell and Uhen2008) included Copemys with Peromyscus and some other New World taxa in his new tribe Democricetodontini, noting the similarity in dental features of Copemys with Old World Democricetodon, but we now understand that Copemys includes a variety of taxa that may not be closely related, and its relationships at the subfamily level remain to be determined (Ronez et al., Reference Ronez, Martin and Pardiñas2020). Copemys mariae (Baskin and Korth, Reference Baskin and Korth1996) and C. esmeraldensis (Clark, Dawson, and Wood, Reference Clark, Dawson and Wood1964) were transferred to the new genus Honeymys, and both may represent early sigmodontines (Kelly et al., Reference Kelly, Martin and Ronez2020; Martin et al., Reference Martin, Peláez-Campomanes, Ronez, Barbière, Kelly, Lindsay, Baskin, Czaplewski and Pardiñas2020). Lindsay's (Reference Lindsay, Janis, Gunnell and Uhen2008) Cricetodontinae is a subfamily now including only some Old World archaic cricetids (e.g., Cricetodon, Deperetomys, Hispanomys, Byzantinia, Ruscinomys) and the species described in this study. As noted earlier, there is considerable disagreement among Eurasian investigators on the content of the Cricetodontinae (Sen and Erbajeva, Reference Sen and Erbajeva2011; López-Guerrero et al., Reference López-Guerrero, Ángeles Álvarez Sierra, García-Paredes and Peláez-Campomanes2014; Marković et al., Reference Marković, de Bruijn, van de Weerd and Wessels2020; Prieto et al., Reference Prieto, Rummel, Schotz and Mein2022) and we have not evaluated all the genera occasionally allocated to this subfamily.
Numerous genetic studies have demonstrated the independence of extant New World cricetids from extant Old World cricetines (Jansa and Weksler, Reference Jansa and Weksler2004; Miller and Engstrom, Reference Miller and Engstrom2008; Keith, Reference Keith2015; Steppan and Schenk, Reference Steppan and Schenk2017), resulting in the use of Neotominae for most North American cricetids (Table 2), Sigmodontinae for the predominantly South American cricetids and relatives in North America, and Tylomyinae for the ambiguous Central American vesper and climbing rats. We follow the latter taxonomy here, with the understanding that further study is necessary to elucidate the relationships of Democricetodon to New World cricetids. We cannot comment on the phylogeny or classification of taxa included in Lindsay's (Reference Lindsay, Janis, Gunnell and Uhen2008) Eucricetodontinae or Eumyini, because the North American taxa in these units have not been evaluated recently. A comparison of Lindsay's (Reference Lindsay, Janis, Gunnell and Uhen2008) cricetid classification to our current interpretation is provided in Table 2.
Table 2. Classification of North American cricetid genera with extinct species according to Lindsay (Reference Lindsay, Janis, Gunnell and Uhen2008) and this study. * = provisional, ** = plus relatives considered distinct genera (e.g., Podomys, Isthmomys).

Acknowledgments
The late E. Lindsay provided gifts of many casts of fossil cricetids. We are grateful to L. Jacobs and J. Saunders for a loan of the UALP Galushamys specimens for study. W. Maddison provided helpful comments on our phylogenetic results. J. Kastigar, L. Flynn, L. Jacobs, Y. Kimura, and an anonymous reviewer provided constructive editorial input. Funding and training for CT scanning were provided by the Doris O. and Samuel P. Welles Fund of the UCMP, Department of Integrative Biology, and the FAVE/Tseng Lab.
Declaration of competing interests
The authors report no competing interest for this study.
Data availability statement
Body mass data for New World cricetid rodents used to generate equation 1, body mass estimates for some New and Old World cricetodontine rodents, list of characters/character states, character state matrix used in cladistic analysis, and lists of terminal taxa apomorphies/node synapomorphies are available as Supplementary Data files 1-5 from the Dryad Digital Repository at https://doi.org/10.5061/dryad.f7m0cfz0r