Introduction
Moderate to severe traumatic brain injury (TBI) in childhood is often associated with acute and chronic neuropsychological impairments, with demonstrated ramifications for adaptive, academic, and social functioning. At the group level, children with more severe injuries, younger age at injury, lower socioeconomic status (SES), poorer premorbid functioning, and less optimal family environmental contexts tend to be at greatest risk for long-term neuropsychological deficits (Anderson, Catroppa, Morse, Haritou, & Rosenfeld, Reference Anderson, Catroppa, Morse, Haritou and Rosenfeld2005; Donders & Kim, Reference Donders and Kim2019; Ewing-Cobbs et al., Reference Ewing-Cobbs, Fletcher, Levin, Frances, Davidson and Miner1997; Max et al., Reference Max, Schachar, Levin, Ewing-Cobbs, Chapman, Dennis and Landis2005; Narad et al., Reference Narad, Treble-Barna, Peugh, Yeates, Taylor, Stancin and Wade2016; Treble-Barna et al., Reference Treble-Barna, Zang, Zhang, Taylor, Stancin, Yeates and Wade2016; Yeates et al., Reference Yeates, Armstrong, Janusz, Taylor, Wade, Stancin and Drotar2005). Despite these known predictors, there remains substantial unexplained heterogeneity in neuropsychological outcomes after pediatric TBI. Individual genetic variability is emerging as a novel additional source of heterogeneity in outcomes after TBI that may influence the brain’s response to injury, including recovery and repair processes (Kurowski et al., Reference Kurowski, Treble-Barna, Pilipenko, Wade, Yeates, Gerry Taylor and Jegga2019; Zeiler et al., Reference Zeiler, McFadyen, Newcombe, Synnot, Donoghue, Ripatti, Steyerberg, Gruen, McAllister, Rosand, Palotie, Maas and Menon2018). Examination of the influence of genetic predisposition on neuropsychological recovery after pediatric TBI holds potential to move the field towards precision medicine, improving prognostic accuracy and allowing for earlier identification of children at greatest risk for long-term impairment.
The emerging literature examining genetic variation in association with clinical outcomes after TBI is focused on the effects of apolipoprotein E (APOE), genes involved in expression of monoamine neurotransmitters, cytokines, and mitochondrial proteins, as well as brain-derived neurotrophic factor (BDNF; Zeiler et al., Reference Zeiler, McFadyen, Newcombe, Synnot, Donoghue, Ripatti, Steyerberg, Gruen, McAllister, Rosand, Palotie, Maas and Menon2018). The BDNF gene is an especially promising candidate gene for examination in TBI because of its role in both response to injury and neurocognitive and behavioral reserve—both of which are biologic processes enriched with genetic variants implicated in clinical outcomes of TBI (Kurowski et al., Reference Kurowski, Treble-Barna, Pitzer, Wade, Martin, Chima and Jegga2017). BDNF is a neurotrophic growth factor that is released from pre- and post-synaptic neurons after neuronal activity. Because it is synthesized as pro-BDNF and subsequently cleaved to mature BDNF, pro-BDNF and mature BDNF function antagonistically in regulating neuroplasticity (Lee, Kermani, Teng, & Hempstead, Reference Lee, Kermani, Teng and Hempstead2001). Pro-BDNF has pro-apoptotic function, activating pathways involved in apoptosis, reduction of dendritic complexity, and neuronal long term depression (Finan, Udani, Patel, & Bailes, Reference Finan, Udani, Patel and Bailes2018; Teng et al., Reference Teng, Teng, Lee, Wright, Tevar, Almeida, Kermani, Chen, Lee, Kraemer, Nykjaer and Hempstead2005; Yang et al., Reference Yang, Harte-Hargrove, Siao, Marinic, Clarke, Ma, Jing, Bath, Mark, Ballon, Lee, Scharfman and Hempstead2014), whereas mature BDNF has pro-survival function, activating pathways involved in cell survival, dendrite formation, and long-term potentiation (Atwal, Massie, Miller, & Kaplan, Reference Atwal, Massie, Miller and Kaplan2000; Dijkhuizen & Ghosh, Reference Dijkhuizen and Ghosh2005; Finan et al., Reference Finan, Udani, Patel and Bailes2018; Patterson et al., Reference Patterson, Abel, Deuel, Martin, Rose and Kandel1996). A single nucleotide polymorphism (SNP) producing a valine-to-methionine substitution at codon 66 (Val66Met; rs6265) in the BDNF gene is associated with reduced activity-dependent secretion of BDNF (Egan et al., Reference Egan, Kojima, Callicott, Goldberg, Kolachana, Bertolino, Zaitsev, Gold, Goldman, Dean, Lu and Weinberger2003). While findings have been somewhat mixed (Harrisberger et al., Reference Harrisberger, Spalek, Smieskova, Schmidt, Coynel, Milnik, Freytag, Gschwind, Walter, Vogel, Bendfeldt, de Quervain, Papassotiropoulos and Borgwardt2014; Hong, Liou, & Tsai, Reference Hong, Liou and Tsai2011; Notaras, Hill, & Van Den Buuse, Reference Notaras, Hill and Van Den Buuse2015), Val66Met allele status, and especially possession of the Met allele, has been associated with variation in brain structure and function, including smaller brain volumes (Kawasaki et al., Reference Kawasaki, Oishi, Hernandez, Ernst, Wu, Otsuka and Chang2021; Nemoto et al., Reference Nemoto, Ohnishi, Mori, Moriguchi, Hashimoto, Asada and Kunugi2006; Pezawas et al., Reference Pezawas, Verchinski, Mattay, Callicott, Kolachana, Straub, Meyer-Lindenberg and Weinberger2004; Szeszko et al., Reference Szeszko, Lipsky, Mentschel, Robinson, Gunduz-Bruce, Sevy, Napolitano, Bilder, Kane, Goldman and Malhotra2005) and lower connectivity (Park et al., Reference Park, Kim, Namgung, Lee, Kim, Kim, Kim, Kim, Lyoo and Yoon2017; Ueda et al., Reference Ueda, Takemoto, Watanabe, Sugimoto, Ikenouchi, Kakeda, Yoshimura and Korogi2020), poorer neuropsychological functioning (Kambeitz et al., Reference Kambeitz, Bhattacharyya, Kambeitz-Ilankovic, Valli, Collier and McGuire2012; Toh, Ng, Tan, Tan, & Chan, Reference Toh, Ng, Tan, Tan and Chan2018), and greater risk for a multitude of psychiatric and neurological conditions, in non-brain-injured individuals (Brown, Vickers, Stuart, Cechova, & Ward, Reference Brown, Vickers, Stuart, Cechova and Ward2020; Notaras et al., Reference Notaras, Hill and Van Den Buuse2015).
Clinical studies evaluating the role of BDNF genetic variation in TBI have produced mixed results, with findings appearing to be moderated by age and injury severity. In studies of adults with mild TBI, results are generally consistent with those in non-brain-injured individuals, with Met allele carriers showing poorer behavioral and neuropsychological outcomes (Gabrys, Dixon, Holahan, & Anisman, Reference Gabrys, Dixon, Holahan and Anisman2019; Narayanan et al., Reference Narayanan, Veeramuthu, Ahmad-Annuar, Ramli, Waran, Chinna, Delano-Wood and Ganesan2016; Wang et al., Reference Wang, Chen, Kuo, Wang, Hsu, Wong, Liao, Zhang, Chiang and Chang2018). Studies in older adults with more severe TBI, however, have shown a protective effect of the Met allele on TBI outcomes (Barbey et al., Reference Barbey, Colom, Paul, Forbes, Krueger, Goldman and Grafman2014; Krueger et al., Reference Krueger, Pardini, Huey, Raymont, Solomon, Lipsky, Hodgkinson, Goldman and Grafman2011), though this effect may be moderated by age (Failla, Conley, & Wagner, Reference Failla, Conley and Wagner2016; Failla et al., Reference Failla, Kumar, Peitzman, Conley, Ferrell and Wagner2015). The Met allele was similarly protective in a recent cohort of children studied at 6 months after mild TBI, with carriers having fewer internalizing problems (Gagner, Tuerk, De Beaumont, Bernier, & Beauchamp, Reference Gagner, Tuerk, De Beaumont, Bernier and Beauchamp2020) and better quality of life (Tuerk et al., Reference Tuerk, Gagner, Dégeilh, Bellerose, Lalonde, Landry-Roy, de Beaumont, Gravel, Bernier and Beauchamp2020). In contrast, in the present cohort, we recently observed a differential effect of Val66Met allele status in children with mostly moderate to severe TBI relative to children with orthopedic injuries (OI), such that the Met allele conferred risk for poorer longitudinal behavioral adjustment in children with TBI but not in children with OI (Treble-Barna et al., Reference Treble-Barna, Wade, Pilipenko, Martin, Yeates, Taylor and Kurowski2021). To our knowledge, no studies have yet examined the influence of BDNF Val66Met on neuropsychological outcomes after pediatric TBI. The present study builds on our prior work in this same cohort (Treble-Barna et al., Reference Treble-Barna, Wade, Pilipenko, Martin, Yeates, Taylor and Kurowski2021) by examining performance-based measures of neuropsychological functioning and caregiver ratings of executive function rather than parent-report measures of behavioral adjustment. Prior research in pediatric TBI and other pediatric neurological conditions has shown that neuropsychological and behavioral outcomes are associated with family environmental factors to varying degrees, with behavioral outcomes often being more sensitive to such factors compared to neuropsychological outcomes (Breslau, Reference Breslau1995; Taylor et al., Reference Taylor, Swartwout, Yeates, Walz, Stancin and Wade2008; Yeates, Taylor, Walz, Stancin, & Wade, Reference Yeates, Taylor, Walz, Stancin and Wade2010). The relative sensitivity of behavioral versus neuropsychological outcomes to genetic factors is less well understood.
The primary aim of the present study was to examine the differential effect of the BDNF Val66Met polymorphism on neuropsychological functioning in children who sustained early childhood TBI relative to children who sustained OI but no brain injury. The inclusion of an OI comparison group is a strength of our study design that allows for differentiation of genetic effects specific to TBI recovery from potential premorbid genetic differences. We hypothesized that BDNF Met allele carriers would have poorer neuropsychological functioning and that this association would be moderated by injury type (e.g., TBI vs. OI), with more pronounced effects within the TBI relative to the OI group. The neuropsychological outcomes examined focus on attention, memory, and executive functions due to their vulnerability to the effects of pediatric TBI. Because executive functions are both highly vulnerable to injury and important for children’s ongoing development, special emphasis was placed on measures of this domain.
Methods
Participants
Participants were drawn from the prospective, longitudinal Ohio Head Injury Outcomes (OHIO) study, which examined children who sustained a TBI or OI between 3 and 7 years of age. Participants were recruited from three tertiary care children’s hospitals and one tertiary care general hospital in Ohio. Inclusion criteria were overnight hospitalization for traumatic injury (TBI or OI), no evidence of child abuse as the cause of the injury, no history of prior TBI, documented neurological problems, or developmental delays pre-injury, and English as the primary language spoken in the home. TBI severity was determined using the lowest post-resuscitation Glasgow Coma Scale (GCS) score and clinically obtained neuroimaging findings. Severe TBI was defined as a GCS score ≤8. Moderate TBI was defined as a GCS score of 9–12 with or without abnormal neuroimaging (moderate TBI) or a higher GCS score with abnormal neuroimaging as defined by an intracranial or parenchymal injury or depressed skull fracture (complicated mild TBI). Mild TBI was defined as a GCS score ≥13 without abnormal neuroimaging. Children in the OI group sustained a bone fracture (not including skull fractures), had an overnight hospitalization, and did not exhibit alterations in consciousness or other signs or symptoms of head trauma or brain injury. The study was completed in accordance with Helsinki Declaration, approved by the institutional review boards at each of the participating medical centers, and informed consent was obtained from participating caregivers.
Neuropsychological outcome measures
Participants completed research assessments at a total of six follow-up visits, including the immediate post-acute period (0–3 months after injury; visit 1); 6, 12, and 18 months post-injury (visits 2–4); and ∼3.5 and ∼7 years post-injury (visits 5 and 6). The same performance-based neuropsychological measures were administered at visits 1–4. The fifth visit involved only mail-in parent-report measures. Because participants were significantly older at visit 6, different age-appropriate performance-based measures of similar neuropsychological constructs were administered. Neuropsychological constructs, associated measures, and scores analyzed are shown in Table 1. Lower scores indicate poorer neuropsychological function for all measures. While most measures are commonly used in pediatric neuropsychological practice, the Shape School and Verbal Paired Associates measures are described in previous papers from our group (Ganesalingam et al., Reference Ganesalingam, Yeates, Taylor, Walz, Stancin and Wade2011; Treble-Barna, Zang, et al., Reference Treble-Barna, Zang, Zhang, Taylor, Yeates and Wade2017).
Table 1. Neuropsychological performance-based measures and scores analyzed by study visit
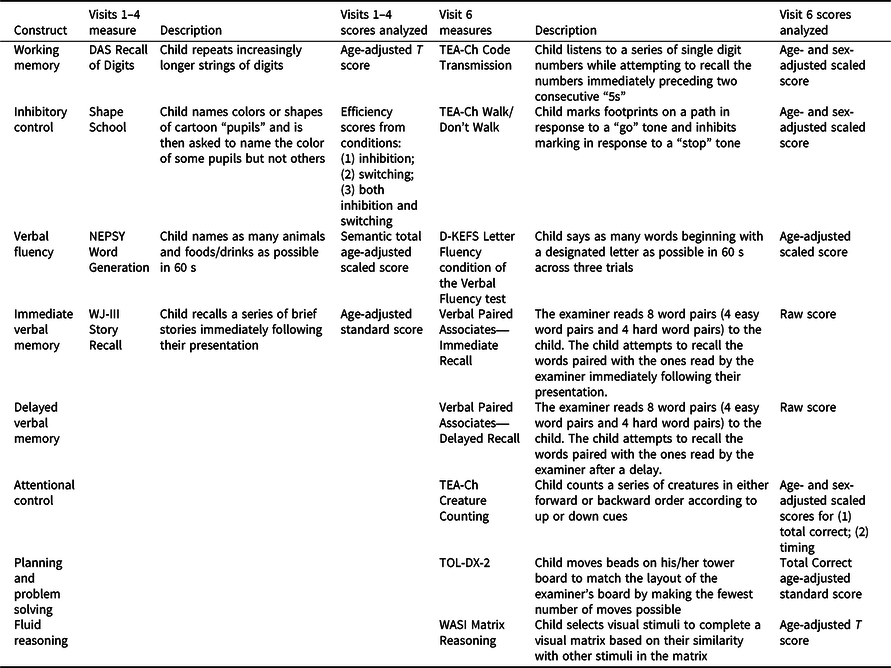
Note. A Developmental NEuroPSYchological Assessment (NEPSY; Korkman, Kirk, & Kemp, Reference Korkman, Kirk and Kemp1998); Delis-Kaplan Executive Function System (D-KEFS; Delis, Kaplan, & Kramer, Reference Delis, Kaplan and Kramer2001); Differential Ability Scales (DAS; Elliott, Reference Elliott1990); Shape School (Espy, Reference Espy1997); Test of Everyday Attention for Children (TEA-Ch; Manly, Robertson, Anderson, & Nimmo-Smith, Reference Manly, Robertson, Anderson and Nimmo-Smith1999); Tower of London-Drexel, Second Edition (TOL-DX-2; Culbertson & Zillmer, Reference Culbertson and Zillmer1998); Verbal Paired Associates (Gonzalez, Anderson, Wood, Mitchell, & Harvey, Reference Gonzalez, Anderson, Wood, Mitchell and Harvey2007); Wechsler Abbreviated Scale of Intelligence (WASI; Wechsler, Reference Wechsler1999); Woodcock-Johnson Tests of Achievement, Third Edition (WJ-III; Woodcock, McGrew, & Mather, Reference Woodcock, McGrew and Mather2001).
In addition to performance-based measures, parents completed the age-appropriate version of the Behavior Rating Inventory of Executive Functioning (BRIEF) (Gioia, Isquith, Guy, Kenworthy, & Baron, Reference Gioia, Isquith, Guy, Kenworthy and Baron2000) at all visits, the first of which involved retrospective recall of the child’s executive functioning prior to injury to control for pre-injury differences in child functioning. The BRIEF is a standardized rating scale on which parents report the frequency of children’s dysexecutive behaviors. T-scores on the General Executive Composite (GEC) Scale were used as the dependent variable. Higher T-scores indicate more dysexecutive behaviors, with T-scores 65 or greater indicating clinically elevated problems.
DNA collection and genotyping
DNA was collected from saliva samples and purified using Oragene OG-500 self-collection tubes (DNA Genotek, Ottawa, Canada). The HumanExome v1.1 Bead Chip (Illumina, San Diego, CA) was used to perform genotyping using the Illumina iScan system to identify the BDNF rs6265 SNP. Genotypes were AA (methionine/methionine homozygote), GA (valine/methionine heterozygote), and GG (valine/valine homozygote). Consistent with prior BDNF val66met studies and to preserve power, we used a dominant model combining individuals with GA and AA genotypes into one group of Met allele carriers based on seminal studies demonstrating that the presence of just one copy of the BDNF Met allele leads to decreased activity-dependent BDNF secretion (Chen et al., Reference Chen, Patel, Sant, Meng, Teng, Hempstead and Lee2004). Principal component analysis was employed to confirm European and African continental ancestry, which aligns with self-reported White and Black race, using 200 validated ancestry informative markers and HapMap genotypic data from individuals of known ancestry as referent groups. Concordance with self-reported race was >95%. Results of analyses were similar when including only participants of European ancestry; therefore, both ancestral groups were retained to preserve power and to be more inclusive of diverse populations (Peterson et al., Reference Peterson, Kuchenbaecker, Walters, Chen, Popejoy, Periyasamy, Iyegbe, Strawbridge, Brick, Carey, Martin, Meyers, Su, Chen, Edwards, Kalungi, Koen, Majara, Schwarz and Duncan2019). Self-reported race was used as a covariate in analyses to control for any differences in allele frequency or linkage disequilibrium (LD) patterns relating to ancestry, as well as the association of race with neuropsychological performance (Olson & Jacobson, Reference Olson and Jacobson2015).
Statistical analysis
All statistical analyses were conducted using SAS 9.4 (SAS, Cary, NC), except computation of conditional R 2 for mixed models, which was performed in R 4.0.2 (https://www.r-project.org). Prior to analyses, outcome scores 4 SDs above the sample mean were winsorized to 4SD (n = 1 score for 1 participant) to reduce the potential influence of outliers. For group comparisons, t-tests, chi-square, or Fisher’s exact tests were employed.
Because some child outcomes were collected longitudinally and some at only one study visit (visit 6 measures), we used two approaches. For neuropsychological outcomes collected longitudinally (visits 1–4 for performance-based measures and visits 1–6 for the BRIEF), we examined genetic associations with outcome by injury group using longitudinal mixed models including all available time points while accounting for the within-subject correlation with an unstructured covariance random effect. Because longitudinal mixed models do not require complete data for all participants, participants with only a subset of study visits were retained. We examined the association of the BDNF rs6265 polymorphism (Met carriers vs. Val/Val homozygotes) with each score on neuropsychological measures and the moderating effect of injury group (TBI vs. OI) on these associations (e.g., allele status × injury group interaction). The three-way interaction of allele status × injury group × time was not examined due to insufficient power. Mixed models included random intercepts, slopes, and subjects. Random slopes were modeled across time. Socioeconomic status (SES; defined by averaging sample Z scores for maternal education and median census tract income (Yeates et al., Reference Yeates, Taylor, Walz, Stancin and Wade2010) was tested as a covariate in base models without allele status and then trimmed if p > .10. Time since injury was covaried in all mixed models except for the Shape School models, in which age at each visit was instead covaried because these were raw scores rather than age-adjusted scores; we did not covary both age and time since injury in the Shape School models to avoid multicollinearity. The child’s pre-injury functioning as assessed at the immediate post injury visit was covaried in the BRIEF model. Self-reported race (dichotomized as White versus African) was covaried in all models. Conditional R 2 for mixed models were examined in R using function “r2_nakagawa” from the Performance package. Conditional R 2 describes the proportion of variation accounted for by both fixed and random effects (Nakagawa & Schielzeth, Reference Nakagawa and Schielzeth2013). To understand interaction effects involving injury group, we plotted least square means for dependent variables for each group by allele status to visualize the direction of effects and conducted post-hoc stratified mixed models within each injury group.
For neuropsychological outcomes collected only at visit 6, we examined genetic associations with outcome by injury group using cross-sectional general linear models, including the allele status × injury group interaction. SES was tested as a covariate in base models without allele status and then trimmed if p > .10. Age was covaried in models of Verbal Paired Associates because these were raw scores rather than age-adjusted scores. Self-reported race was covaried in all models. Proportion of variance accounted for by cross-sectional models was examined using η 2.
To provide effect size estimates, we standardized all continuous variables (M = 0, SD = 1) other than age and time since injury prior to analyses and obtained parameter estimates based on the final model for each dependent variable. The resulting coefficients are akin to standardized regression coefficients for continuous predictors and to standardized mean differences (e.g., Cohen’s d) for categorical variables. Because standardized regression coefficients can be scaled to correlations (Cohen, Reference Cohen1988), we used conventional definitions of effect size to characterize the magnitude of standardized parameter estimates for continuous predictors (i.e., 0.1 is small, 0.3 is medium, and 0.5 is large). Likewise, we used conventional definitions of effect size for mean differences to characterize the magnitude of parameter estimates for categorical predictors and any interactions involving them (i.e., 0.2 is small, 0.5 is medium, 0.8 is large).
We recognize that the number of tests performed may result in an inflated type 1 error rate in the absence of corrections for multiple testing. The Bonferroni correction, which is often used for genetic association studies, assumes that multiple tests are independent of each other. Because our outcomes are not independent, we used the Dubey/Armitage-Parmar procedure (Sankoh, Huque, & Dubey, Reference Sankoh, Huque and Dubey1997) to identify an appropriate correlation-adjusted Bonferroni correction. For longitudinal models, the overall correlation between the seven outcomes was 0.13 yielding a multiple testing threshold for statistical significance of α = 0.007. For cross-sectional models, the overall correlation was 0.27 between the 9 models, yielding a multiple testing threshold for statistical significance of α = 0.012. However, because the probability of detecting interaction effects in non-experimental research designs is low (McClelland & Judd, Reference McClelland and Judd1993) and to limit type II error in this exploratory study, we also considered results of interactions of allele status by injury group with nominal significance (p < .05) or at least medium effect sizes (e.g., ≥0.5 for interactions) noteworthy and further explored these in post-hoc analyses.
Results
Sample description
Of the 221 participants enrolled in the original study, 217 were eligible for the final study visit at which DNA samples were collected. We successfully re-established contact with 163 participants, 142 of whom provided DNA samples. More detailed attrition data for the total sample is reported elsewhere (Narad et al., Reference Narad, Riemersma, Wade, Smith-Paine, Morrison, Taylor, Yeates and Kurowski2019, Reference Narad, Treble-Barna, Peugh, Yeates, Taylor, Stancin and Wade2016). Participants with genetic data did not differ significantly from those without genetic data in demographic characteristics or on various study measures, as previously reported (Treble-Barna, Wade, et al., Reference Treble-Barna, Wade, Martin, Pilipenko, Yeates, Taylor and Kurowski2017). Of those with genetic data, 141 of 142 participants had covariate and outcome data for at least one outcome visit to be included in the present analyses. Ten had mild TBI, 43 had complicated mild to moderate TBI, 16 had severe TBI, and 72 had OI. The TBI and OI groups were comparable in race, sex, age at injury, age at assessment, and SES, and differed by mechanism of injury (Table 2). Descriptive data for the neuropsychological measures are provided in Supplemental Table 1. Injury group comparisons between the various neuropsychological measures with appropriate covariates have been reported previously (Ganesalingam et al., Reference Ganesalingam, Yeates, Taylor, Walz, Stancin and Wade2011; Gerrard-Morris et al., Reference Gerrard-Morris, Taylor, Yeates, Walz, Stancin, Minich and Wade2010; Narad et al., Reference Narad, Treble-Barna, Peugh, Yeates, Taylor, Stancin and Wade2017; Taylor et al., Reference Taylor, Swartwout, Yeates, Walz, Stancin and Wade2008; Treble-Barna, Zang, et al., Reference Treble-Barna, Zang, Zhang, Taylor, Yeates and Wade2017).
Table 2. Demographic, outcome, and Val66Met characteristics by injury group
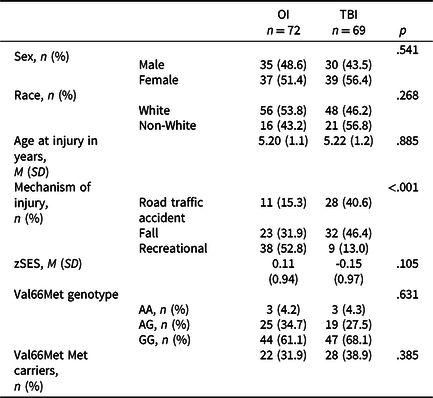
TBI = traumatic brain injury; M = mean; OI = orthopedic injury; SD = standard deviation; zSES = socioeconomic status Z score.
The BDNF rs6265 SNP did not violate Hardy–Weinberg equilibrium. No significant differences in genotype distribution or Met allele status were found between the TBI and OI groups (Table 2). TBI severity groups were also comparable for Met allele status (Fisher’s exact test p = .104; Met allele carriers, mild = 1, moderate = 13, severe = 8).
Longitudinal mixed models
Table 3 shows results of longitudinal mixed models testing the allele status x injury group interactions. Of the seven models, the allele status x injury group interaction was statistically significant for NEPSY Word Generation with a medium effect (standardized estimate = 0.72, p = .007). No other allele status × injury group interactions were nominally significant or of at least medium effect size. These results suggest a differential effect of Met carrier status on longitudinal verbal fluency between the TBI and OI groups.
Table 3. Results of longitudinal mixed models testing the allele status × injury group interaction
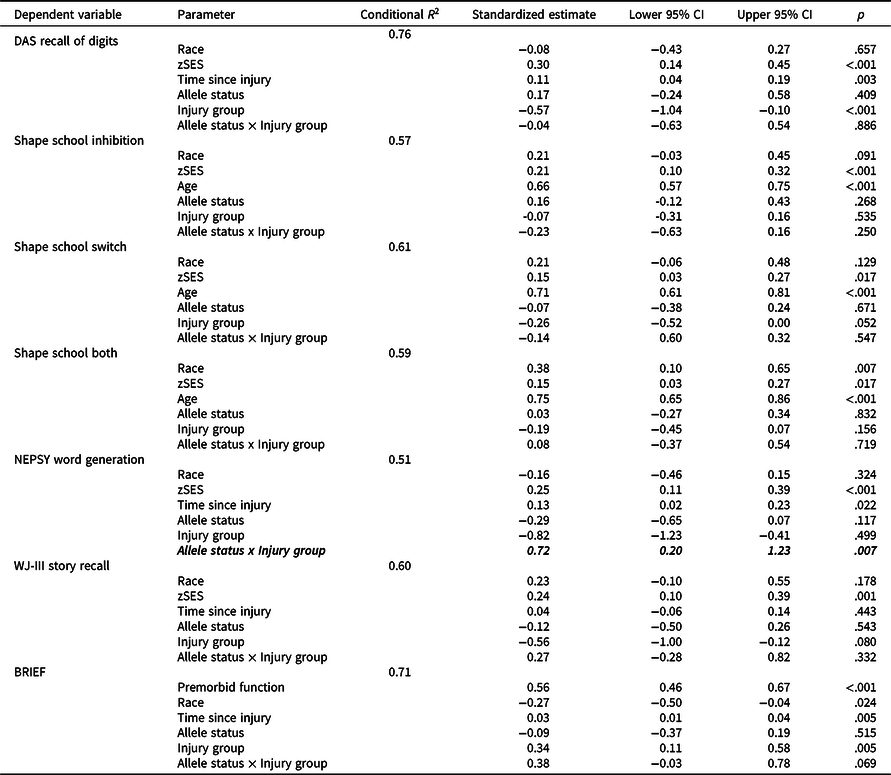
TBI = traumatic brain injury; OI = orthopedic injury; zSES = socioeconomic status Z score.
Allele status × injury group interactions with p < .007 (correlation-adjusted Bonferroni correction for multiple testing threshold) are bolded.
Allele status × injury group interactions with at least medium effect sizes (e.g. ≥0.5) are italicized.
Figure 1 plots least square means for NEPSY Word Generation for each group by allele status and Table 4 provides results of post-hoc stratified models. Met carriers in the TBI group had poorer verbal fluency relative to Val/Val homozygotes, with a small effect (standardized estimate = −0.39, p = .039); whereas the opposite trend was observed in the OI group, though the effect was small and nonsignificant (standardized estimate = 0.28, p = .162).
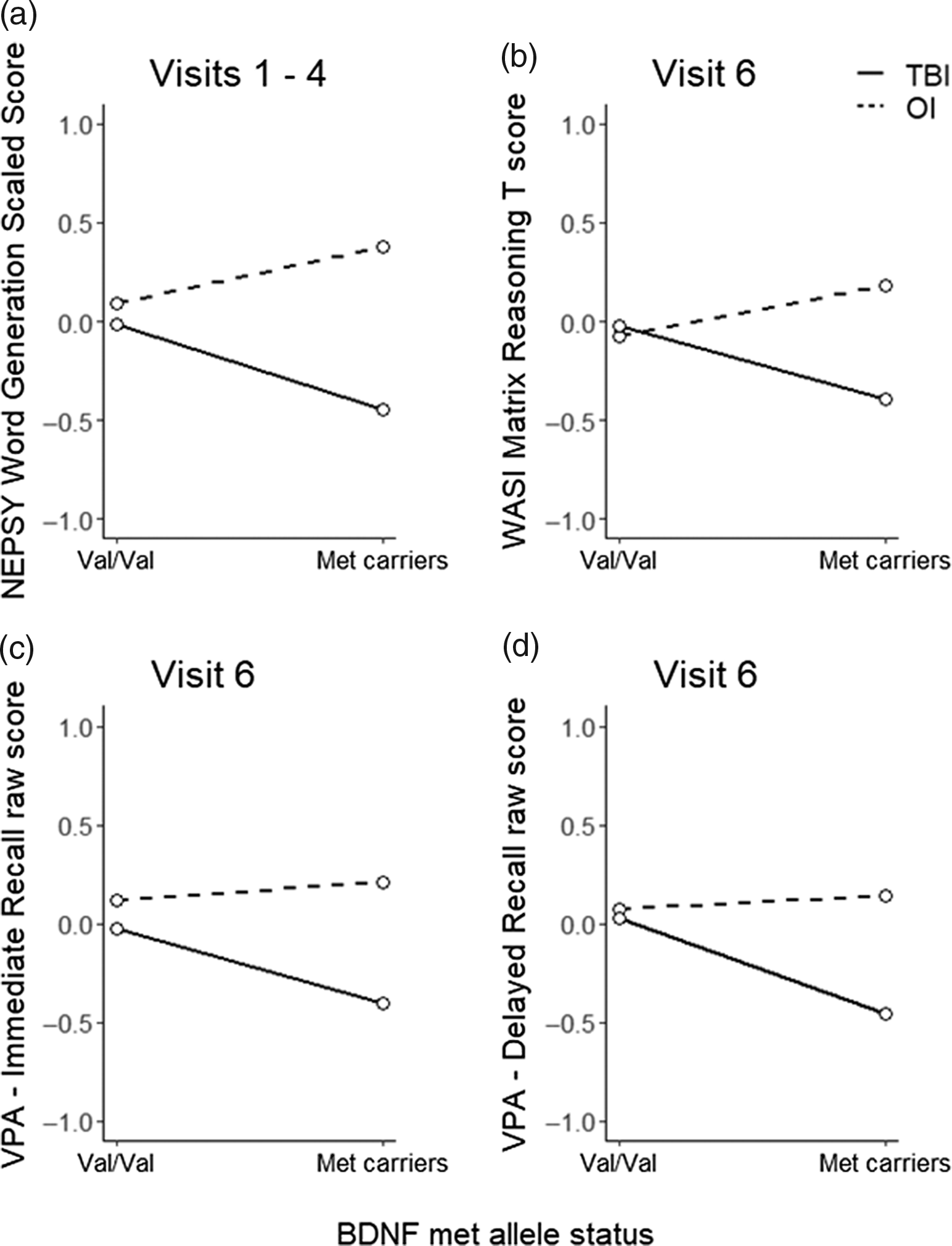
Figure 1. Least square means for dependent variables by injury group and allele status for significant and non-significant trend allele status x injury group interactions. BDNF, brain-derived neurotrophic factor; NEPSY, A Developmental NEuroPSYchological Assessment; OI, orthopedic injury; TBI, traumatic brain injury; VPA, Verbal Paired Associates; WASI, Wechsler Abbreviated Scale of Intelligence.
Table 4. Results of longitudinal mixed models post-hoc stratified analyses
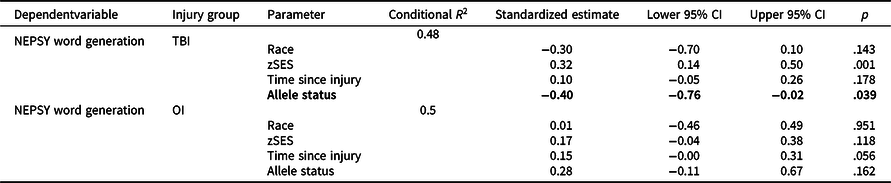
OI = orthopedic injury; TBI = traumatic brain injury; zSES = socioeconomic status Z score.
Allele status effects with p < .05 are bolded and with at least medium effect sizes (e.g. ≥0.5) italicized.
Cross-sectional general linear models
Table 5 shows results of cross-sectional general linear models testing the allele status x injury group interactions for measures administered at visit 6 only. Of the nine models, the allele status x injury group interaction was not statistically or nominally significant in any model; however, models for WASI Matrix Reasoning (standardized estimate = -0.63, p = .074), Verbal Paired Associates—Immediate Recall (standardized estimate = −0.46, p = .209), and Verbal Paired Associates—Delayed Recall (standardized estimate = −0.55, p = .131) showed non-significant medium effects for the allele status × injury group interaction and were further explored in post-hoc analyses.
Table 5. Results of cross-sectional general linear models testing the allele status × injury group interaction
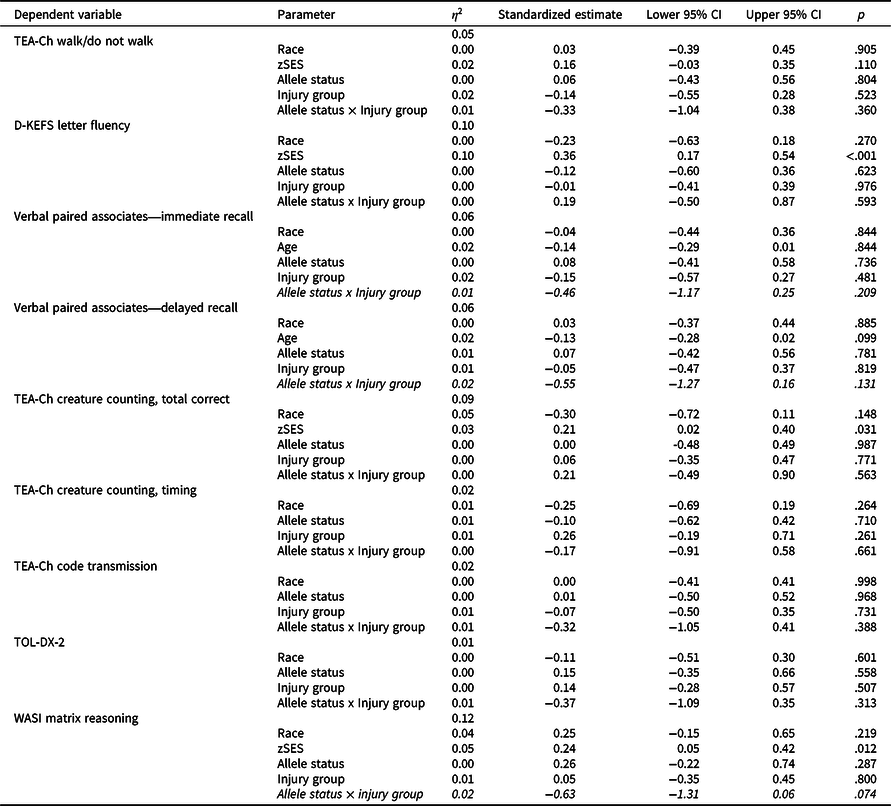
TBI = traumatic brain injury; OI = orthopedic injury; zSES = socioeconomic status Z score.
Allele status × injury group interactions with p < .05 are bolded and with at least medium effect sizes (e.g. ≥0.5) italicized.
Figure 1 plots least square means for each group by allele status and Table 6 provides results of post-hoc stratified models. Although Met carriers in the TBI group tended to have poorer fluid reasoning (standardized estimate = −0.34, p = .268), immediate verbal memory (standardized estimate = 0.32, p = .310), and delayed verbal memory (standardized estimate = 0.42, p = .198) relative to Val/Val homozygotes, these within group effects were small and non-significant. In contrast, in the OI group, allele status had a small non-significant effect on fluid reasoning in the opposite direction relative to TBI and no effect on immediate verbal memory (standardized estimate = 0.03, p = .899) or delayed verbal memory (standardized estimate = 0.00, p = .990).
Table 6. Results of cross-sectional general linear models post-hoc stratified analyses
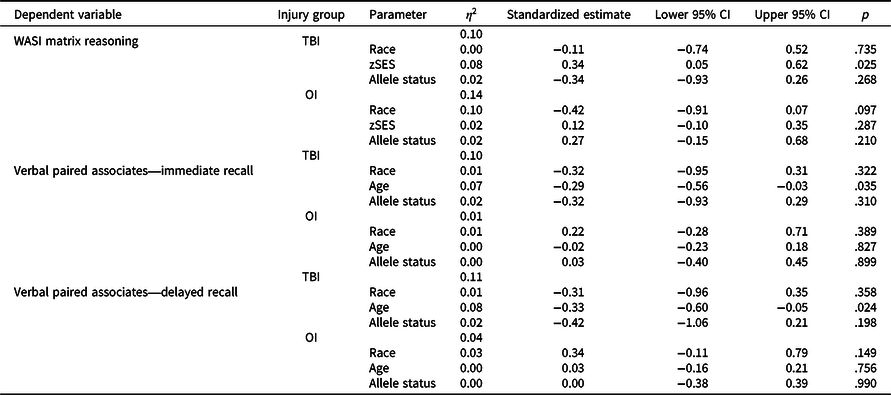
OI = orthopedic injury; TBI = traumatic brain injury; zSES = socioeconomic status Z score.
Discussion
The present study investigated the differential effect of the BDNF Val66Met polymorphism on neuropsychological functioning in children who sustained early TBI relative to children who sustained OI. Longitudinal mixed model analyses revealed a significant allele status × injury group interaction with a medium effect for verbal fluency, and cross-sectional models revealed medium but non-significant allele status × injury group interactions for fluid reasoning and immediate and delayed verbal memory. These effects were over and above the effects of covariates, which included race, SES, and time since injury or age, depending on the model. Post-hoc analyses of the notable interactions suggested a consistent pattern of poorer neuropsychological functioning in Met carriers relative to Val/Val homozygotes in the TBI group, with small effects that reached statistical significance for verbal fluency but not for fluid reasoning or verbal memory. In contrast, the opposite trend or no appreciable effect of allele status was observed in the OI group. The results may suggest a differential effect of the BDNF Val66Met polymorphism on verbal fluency, and possibly on fluid reasoning, and verbal memory, in children with early TBI relative to OI, and that the Met allele—associated with reduced activity-dependent secretion of BDNF—may confer risk for poorer neuropsychological functioning in children with TBI. Although preliminary, and requiring validation with larger and more homogeneous samples, these findings raise the potential of the Met allele serving as a marker for neuropsychological risk following pediatric TBI.
The present results add to an emerging literature showing that the role of BDNF in recovery from TBI is complex and dynamic, with effects appearing to vary by injury severity and age at injury (Barbey et al., Reference Barbey, Colom, Paul, Forbes, Krueger, Goldman and Grafman2014; Failla et al., Reference Failla, Conley and Wagner2016, Reference Failla, Kumar, Peitzman, Conley, Ferrell and Wagner2015; Gabrys et al., Reference Gabrys, Dixon, Holahan and Anisman2019; Krueger et al., Reference Krueger, Pardini, Huey, Raymont, Solomon, Lipsky, Hodgkinson, Goldman and Grafman2011; Narayanan et al., Reference Narayanan, Veeramuthu, Ahmad-Annuar, Ramli, Waran, Chinna, Delano-Wood and Ganesan2016; Wang et al., Reference Wang, Chen, Kuo, Wang, Hsu, Wong, Liao, Zhang, Chiang and Chang2018). Adult studies have shown poorer outcomes in Met carriers with mild TBI (Gabrys et al., Reference Gabrys, Dixon, Holahan and Anisman2019; Narayanan et al., Reference Narayanan, Veeramuthu, Ahmad-Annuar, Ramli, Waran, Chinna, Delano-Wood and Ganesan2016; Wang et al., Reference Wang, Chen, Kuo, Wang, Hsu, Wong, Liao, Zhang, Chiang and Chang2018). In adults with severe TBI, however, the Met allele appears to confer risk in younger adults but protection in older adults (Failla et al., Reference Failla, Conley and Wagner2016, Reference Failla, Kumar, Peitzman, Conley, Ferrell and Wagner2015). In contrast, Met carriers have shown fewer internalizing problems (Gagner et al., Reference Gagner, Tuerk, De Beaumont, Bernier and Beauchamp2020) and better quality of life (Tuerk et al., Reference Tuerk, Gagner, Dégeilh, Bellerose, Lalonde, Landry-Roy, de Beaumont, Gravel, Bernier and Beauchamp2020) in a cohort of children with mild TBI. The present results are consistent with our prior report in this cohort (Treble-Barna et al., Reference Treble-Barna, Wade, Pilipenko, Martin, Yeates, Taylor and Kurowski2021), suggesting that the Met allele (associated with reduced activity-dependent secretion of BDNF) may confer risk for poorer neuroplasticity and repair mechanisms after TBI, affecting both longitudinal behavioral adjustment and neuropsychological functioning. These inconsistent findings regarding risk versus protection of the Met allele are not unique to TBI, with similar complex and conflicting findings in other clinical conditions (Hong et al., Reference Hong, Liou and Tsai2011; Notaras et al., Reference Notaras, Hill and Van Den Buuse2015), perhaps most relevantly in stroke (Rezaei, Asgari Mobarake, & Saberi, Reference Rezaei, Asgari Mobarake and Saberi2020; Rezaei, Mobarake, Saberi, & Keshavarz, Reference Rezaei, Mobarake, Saberi and Keshavarz2020). Reviews of the effect of Val66Met in other populations have cited heterogeneity in confounders such age, sex, environmental factors, sample size, ethnicity, and phenotype assessment as likely accounting for some of the mixed findings (Hong et al., Reference Hong, Liou and Tsai2011; Notaras et al., Reference Notaras, Hill and Van Den Buuse2015). In stroke, Val66Met status has shown significant interactions with age, dominant hemisphere lesions, degree of cerebral atrophy, number of lesions, recovery stage, and family history of dementia in predicting neuropsychological outcomes (Balkaya & Cho, Reference Balkaya and Cho2019; Rezaei, Asgari Mobarake, et al., Reference Rezaei, Asgari Mobarake and Saberi2020; Rezaei, Mobarake, et al., Reference Rezaei, Mobarake, Saberi and Keshavarz2020). Within TBI, age-related differences in the effect of the BDNF gene on outcomes have been suggested as potentially related to reductions in BDNF levels (Islam, Mulsant, Voineskos, & Rajji, Reference Islam, Mulsant, Voineskos and Rajji2017) and regional balances of BDNF pro- vs. mature receptor ratios across the lifespan (Failla et al., Reference Failla, Kumar, Peitzman, Conley, Ferrell and Wagner2015; Finan et al., Reference Finan, Udani, Patel and Bailes2018; Tapia-Arancibia, Aliaga, Silhol, & Arancibia, Reference Tapia-Arancibia, Aliaga, Silhol and Arancibia2008). Additional research is needed to further elucidate effects of BDNF on neurobehavioral recovery after TBI and factors moderating its complex association with outcomes.
Several study limitations should be considered in interpretation of the results. Due to the exploratory nature of the study, we corrected for multiple testing but also explored nominally significant and non-significant interaction effects with at least medium effect sizes. We found only one statistically significant allele status × injury group interaction that survived multiple testing correction and had a medium effect size, as well as three additional non-significant interactions of medium effect size. The power to detect these interactions was likely somewhat limited due to the relatively small sample size. We, therefore, urge caution in interpretation of the results. Notably, both significant and non-significant findings were consistent in the direction of effects within injury groups and consistent with our hypotheses. Nevertheless, the effect of the Val66Met allele on neuropsychological functioning after pediatric TBI appears to be modest. Modest results are not unexpected as the effects of single SNPs on complex phenotypes are often small and interact with the effects of many other potential genes and biological processes. For BDNF in particular, studies have shown interactive effects with APOE, COMT, serotonin transporter polymorphisms, and other neurotrophic factor genes in association with neurological and psychiatric outcomes (Chen et al., Reference Chen, Chen, Xia, Wu, Chen, He, Xue, Wang, He and Dong2016; Harkness et al., Reference Harkness, Strauss, Michael Bagby, Stewart, Larocque, Mazurka, Ravindran, Wynne-Edwards, Rector and Kennedy2015; Stonnington et al., Reference Stonnington, Velgos, Chen, Syed, Huentelman, Thiyyagura, Lee, Caselli, Locke, Lu, Reiman, Su and Chen2020). Future research into the effects of the BDNF gene and others on TBI outcomes would benefit from the concurrent examination of multiple genes, such as through polygenic risk score and systems-biology informed gene-enrichment (Kurowski et al., Reference Kurowski, Treble-Barna, Pilipenko, Wade, Yeates, Gerry Taylor and Jegga2019) approaches. In addition, the effect of the BDNF gene on brain structure and function has been shown to be moderated by environmental factors. Investigation is underway to examine the combined effect of childhood environmental factors and the BDNF gene on neurobehavioral recovery after pediatric TBI by studying BDNF epigenetics (Treble-Barna et al., Reference Treble-Barna, Patronick, Uchani, Marousis, Zigler, Fink, Kochanek, Conley and Yeates2020).
Another notable limitation is that our TBI group was heterogeneous. While the sample was representative of the spectrum of TBI severity, the effects we identified might be present only in a subgroup (e.g., mild or severe). Due to the relatively small sample size, we were unable to examine the moderating effects of injury severity, other injury-related medical variables, or individual background characteristics on the results, but future studies should consider such impact. Indeed, while the present cohort represents one of the largest investigations of the influence of genetic factors on pediatric TBI outcomes to date, larger sample sizes are needed to replicate the findings. Additional limitations are that the neuropsychological test battery included different tests at the earlier and final visits, precluding examination of longitudinal models across the entire study period, and the lack of performance validity testing.
The results provide preliminary evidence for the differential effect of variation in the BDNF Val66Met polymorphism on neuropsychological functioning in children with TBI relative to children with OI. Investigation of genetic biomarkers of pediatric TBI recovery has the potential to advance the field of pediatric TBI toward precision medicine, improving prognostic accuracy and providing earlier identification of children at greatest risk for long-term impairment to provide intervention. A better understanding of the role of BDNF in recovery from TBI may enable translation of pharmacological and non-pharmacological interventions targeting the BDNF pathway that have improved cognitive outcomes in experimental models of TBI.
Financial support
This study was supported in part by the National Institute for Child Health and Human Development (NICHD) grants K12 HD001097-16, K01 HD097030, and R01 HD42729. Additional support was provided by the National Institute of Neurological Disorders and Stroke grant 1R01NS096053, and grant 8 UL1 TR000077 from the National Center for Advancing Translational Sciences (NCATS). Keith Yeates is supported by the Ronald and Irene Ward Chair in Pediatric Brain Injury, funded by the Alberta Children’s Hospital Foundation. The content is solely the responsibility of the authors and does not necessarily represent the official views of the funders. No competing financial interests exist.
Conflicts of interest
None.