Introduction
The noxious and invasive weeds hoary cress [Lepidium draba L.; also known as Cardaria draba (L.) Desv. or heart-podded hoary cress] and hairy whitetop (Lepidium appelianum Al-Shehbaz; also known as globe-podded hoary cress) belong to the Brassicaceae (Francis and Warwick Reference Francis and Warwick2008). These closely related species rank 8th out of the 45 most frequently listed noxious weeds of agricultural land, pastures, and riparian and waste areas in the western United States and Canada (Supplementary Figure S1; Mulligan Reference Mulligan2002; Mulligan and Findlay Reference Mulligan and Findlay1974; Skinner et al. Reference Skinner, Smith and Rice2000). Both L. draba and L. appelianum are native to Eurasia and have high competitiveness and invasiveness in their native, expanded, and introduced ranges (Francis and Warwick Reference Francis and Warwick2008; Hinz et al. Reference Hinz, Schwarzlander, McKenney, Cripps, Harmon and Price2012). Lepidium draba and L. appelianum occur on a variety of soil types, including saline soils where moisture is in at least moderate supply (Darbyshire Reference Darbyshire2003; Hooks et al. Reference Hooks, Picchioni, Schutte, Shukla and Daniel2018). They grow in regions with abundant irrigation (Francis and Warwick Reference Francis and Warwick2008), wet and dry grasslands, scrublands, and arid regions with alkaline soils (Mulligan Reference Mulligan2002; Mulligan and Findlay Reference Mulligan and Findlay1974). The reproductive biology of L. draba is, at least in part, responsible for its wide distribution, but very little is known about the underpinning mechanisms. What is known about it is only based on studying the germination and seedling growth of “isolated” seeds, that is, seeds manually extracted from their fruits (Hooks et al. Reference Hooks, Picchioni, Schutte, Shukla and Daniel2018; Rezvani and Zaefarian Reference Rezvani and Zaefarian2016; Rezaee et al. Reference Rezaee, Lahouti, Maleki and Ganjeali2018). However, a key feature of L. draba and L. appelianum is that they produce indehiscent fruits that do not open to release seeds (Mühlhausen et al. Reference Mühlhausen, Lenser, Mummenhoff and Theissen2013; Mummenhoff et al. Reference Mummenhoff, Polster, Muhlhausen and Theissen2009). This means that L. draba and L. appelianum seeds are dispersed encased in their fruit coat (pericarp) as indehiscent fruits (Figure 1C and D). Neither the dormancy and germination mechanisms of these indehiscent fruits nor the possible role of the pericarp in the control of fruit germination timing have been studied.

Figure 1. Seed and fruit structure and germination of Lepidium draba and Lepidium appelianum. Seeds tightly adhere to the fruit wall in L. draba but not in L. appelianum. (A) Lepidium draba seed (oval); (B) L. appelianum seed (oval and flattened); (C) L. draba fruit (heart-podded); (D) L. appelianum fruit (globe-podded); (E) L. draba manually opened fruits, seeds are tightly adhered to the pericarp (fruit wall); and (F) L. appelianum manually opened fruits, seeds are loosely adhered to the fruit wall. Radicle emergence through the ruptured testa and endosperm marks the completion of germination of imbibed seeds of L. draba (G) and L. appelianum (H). (I) Pericarp rupture and radicle emergence as visible events marking the completion of L. draba fruit germination. (J) Pericarp rupture and radicle emergence following the seed germination within the L. appellianum fruits. A Leica M165 FC Fluorescence Classic Stereomicroscope (Wetzlar, Germany) was used to take pictures of seeds and fruits.
Molecular phylogenetic studies within the genus Lepidium revealed that indehiscent fruits (not releasing seeds) evolved independently several times from dehiscent fruits, that is, fruits that open at maturity to release seeds (Mühlhausen et al. Reference Mühlhausen, Lenser, Mummenhoff and Theissen2013; Mummenhoff et al. Reference Mummenhoff, Polster, Muhlhausen and Theissen2009). These very closely related study species with indehiscent fruits, L. draba and L. appelianum, are therefore embedded in an abundant number of Lepidium species with dehiscent fruits. Indehiscent fruits may have evolved for several reasons: escape in time and space (Eriksson Reference Eriksson2008; Hu et al. Reference Hu, Yu, Yang, Wu, Wang and Boelt2010), protection of seeds against predation (Mamut et al. Reference Mamut, Tan, Baskin and Baskin2014; Ohadi et al. Reference Ohadi, Mashhadi and Tavakol-Afshari2011), or high soil-surface temperatures (Mamut et al. Reference Mamut, Tan, Baskin and Baskin2014; Moreira and Pausas Reference Moreira and Pausas2012). Alternatively, fruits may control germination timing via pericarp-imposed dormancy to ensure that seedling establishment occurs in the right season (Hu et al. Reference Hu, Yu, Yang, Wu, Wang and Boelt2010; Ohadi et al. Reference Ohadi, Mashhadi and Tavakol-Afshari2011; Mamut et al. Reference Mamut, Tan, Baskin and Baskin2014; Sperber et al. Reference Sperber, Steinbrecher, Graeber, Scherer, Clausing, Wiegand, Hourston, Kurre, Leubner-Metzger and Mummenhoff2017).
Lepidium species with dehiscent fruits are known to have either physiologically dormant (PD) or nondormant (ND) seeds (Baskin and Baskin Reference Baskin and Baskin2014; Finch-Savage and Leubner-Metzger Reference Finch-Savage and Leubner-Metzger2006; Willis et al. Reference Willis, Baskin, Baskin, Auld, Venable, Cavender-Bares, Donohue and Rubio de Casas2014). Garden cress (Lepidium sativum L.) is an example of a species that produces ND seeds, and its endosperm acts as a constraint to radicle protrusion (Linkies et al. Reference Linkies, Müller, Morris, Turečková, Cadman, Corbineau, Strnad, Lynn, Finch-Savage and Leubner-Metzger2009; Müller et al. Reference Müller, Tintelnot and Leubner-Metzger2006; Steinbrecher and Leubner-Metzger Reference Steinbrecher and Leubner-Metzger2017). ND seeds have the capacity to germinate over the widest range of normal physical environmental conditions. Warty peppercress (Lepidium papillosum F. Muell.) and mouse-ear cress [Arabidopsis thaliana (L.) Heynh.] are Brassicaceae species that produce PD seeds (Graeber et al. Reference Graeber, Linkies, Müller, Wunchova, Rott and Leubner-Metzger2010, Reference Graeber, Voegele, Büttner-Mainik, Sperber, Mummenhoff and Leubner-Metzger2013). This form of dormancy provides seasonal cueing to ensure that germination occurs only upon specific environmental triggers (Baskin and Baskin Reference Baskin and Baskin2004, Reference Baskin and Baskin2014; Finch-Savage and Leubner-Metzger Reference Finch-Savage and Leubner-Metzger2006; Willis et al. Reference Willis, Baskin, Baskin, Auld, Venable, Cavender-Bares, Donohue and Rubio de Casas2014). Rupture of the testa (seed coat) and rupture of the endosperm are two sequential events during the germination of L. sativum and A. thaliana (Graeber et al. Reference Graeber, Linkies, Steinbrecher, Mummenhoff, Tarkowská, Turečková, Ignatz, Sperber, Voegele, de Jong, Urbanová, Strnad and Leubner-Metzger2014; Steinbrecher and Leubner-Metzger Reference Steinbrecher and Leubner-Metzger2017). Abscisic acid (ABA) specifically inhibits the endosperm rupture of these two Brassicaceae species (Graeber et al. Reference Graeber, Linkies, Steinbrecher, Mummenhoff, Tarkowská, Turečková, Ignatz, Sperber, Voegele, de Jong, Urbanová, Strnad and Leubner-Metzger2014; Linkies et al. Reference Linkies, Müller, Morris, Turečková, Cadman, Corbineau, Strnad, Lynn, Finch-Savage and Leubner-Metzger2009; Müller et al. Reference Müller, Tintelnot and Leubner-Metzger2006; Steinbrecher and Leubner-Metzger Reference Steinbrecher and Leubner-Metzger2017; Voegele et al. Reference Voegele, Linkies, Müller and Leubner-Metzger2011). The seed dormancy–specific gene Delay of Germination1 (DOG1) is widespread, including within the genus Lepidium, and together with ABA controls the dormant state and seed response toward environmental conditions (Graeber et al. Reference Graeber, Linkies, Müller, Wunchova, Rott and Leubner-Metzger2010, Reference Graeber, Voegele, Büttner-Mainik, Sperber, Mummenhoff and Leubner-Metzger2013, Reference Graeber, Linkies, Steinbrecher, Mummenhoff, Tarkowská, Turečková, Ignatz, Sperber, Voegele, de Jong, Urbanová, Strnad and Leubner-Metzger2014). While ABA inhibits germination and maintains PD, the antagonistically acting gibberellins (GA) release PD and promote germination of ND and PD seeds (Finch-Savage and Leubner-Metzger Reference Finch-Savage and Leubner-Metzger2006). Rezvani and Zaefarian (Reference Rezvani and Zaefarian2016) demonstrated that GA treatment replaces the light required for the germination of L. draba “isolated” (i.e., manually removed from fruits) seeds, but nothing is known about the hormonal and pericarp-associated mechanisms underpinning the germination of L. draba and L. appelianum indehiscent fruits.
The pericarp (fruit coat) can control germination and seedling establishment timing by inhibiting or delaying water uptake (Cousens et al. Reference Cousens, Young and Tadayyon2010; Sperber et al. Reference Sperber, Steinbrecher, Graeber, Scherer, Clausing, Wiegand, Hourston, Kurre, Leubner-Metzger and Mummenhoff2017); via germination-inhibiting chemicals, including ABA (Baskin and Baskin Reference Baskin and Baskin2014; Mamut et al. Reference Mamut, Tan, Baskin and Baskin2014; Sari et al. Reference Sari, Oguz and Bilgic2006); or by other means of inhibiting radicle protrusion, including pericarp-imposed mechanical dormancy (Cousens et al. Reference Cousens, Young and Tadayyon2010; Lu et al. Reference Lu, Zhou, Tan, Baskin and Baskin2015; Neya et al. Reference Neya, Hoekstra and Golovina2008; Sperber et al. Reference Sperber, Steinbrecher, Graeber, Scherer, Clausing, Wiegand, Hourston, Kurre, Leubner-Metzger and Mummenhoff2017; Steinbrecher and Leubner-Metzger Reference Steinbrecher and Leubner-Metzger2017). Within the Brassicaceae, the known cases of pericarp-imposed dormancy are not due to complete water impermeability of fruit and seed coats, and the encased seeds are either ND or PD. In lesser swinecress (Lepidium didymum L.) fruits, for example, the ND seeds are encased by a hard pericarp that confers a mechanical constraint to full water uptake required for the completion of germination by radicle protrusion (Sperber et al. Reference Sperber, Steinbrecher, Graeber, Scherer, Clausing, Wiegand, Hourston, Kurre, Leubner-Metzger and Mummenhoff2017). In the case of L. didymum, the tight encasement of the seeds by the pericarp, which prevails even after the pericarp-mediated dormancy has been released, does not allow the seeds to germinate within the fruits. It is therefore the fruit itself that eventually completes germination, with visible radicle emergence through all the layers covering the seed (endosperm, testa) and the fruit (pericarp). Similar cases in which the germination of PD or ND seeds and their radicle emergence from the dispersed indehiscent fruits are restrained by the water-permeable pericarp have been described (Cousens et al. Reference Cousens, Young and Tadayyon2010; Lu et al. Reference Lu, Zhou, Tan, Baskin and Baskin2015, Reference Lu, Tan, Baskin and Baskin2017; Zhou et al. Reference Zhou, Lu, Tan, Baskin and Baskin2015). There are, however, cases in which the seed or fruit coats confer complete water impermeability (Baskin and Baskin Reference Baskin and Baskin2003; Gama-Arachchige et al. Reference Gama-Arachchige, Baskin, Geneve and Baskin2013; Smykal et al. Reference Smykal, Vernoud, Blair, Soukup and Thompson2014; Steinbrecher and Leubner-Metzger Reference Steinbrecher and Leubner-Metzger2018). This—and only this—is then called physical dormancy, which has water-impermeable seeds and/or fruit coats as its hallmark (Baskin and Baskin 2003, Reference Baskin and Baskin2014; Steinbrecher and Leubner-Metzger Reference Steinbrecher and Leubner-Metzger2017, Reference Steinbrecher and Leubner-Metzger2018).
The properties, possible roles, and mechanisms of the pericarp in the germination of indehiscent fruits of L. draba and L. appelianum have not been studied. Because noxious and invasive weeds are a major concern for agriculture and biodiversity, knowing the ecophysiological mechanisms of the indehiscent fruits of L. draba and L. appelianum is important to inform effective management strategies.
Materials and Methods
Seed Sources
Two L. draba accessions from two continents, KM 1296 (from Logan’s Market, Malheur, OR, USA) and KM 1568 (from a vineyard near Hayesdorf, Austria) were used for this work. Freshly harvested mature fruits of L. draba (KM 1296 and KM 1568) and L. appelianum (KM 1754; obtained from J Gaskin, USDA, Fremont County, Wyoming, USA) were collected from plants cultivated in the Botanical Garden, Osnabrueck University, Germany, in 2014 to 2015. In addition, fresh mature fruits were harvested for both L. draba accessions in 2015 to 2016, and further for KM 1568 in 2016 to 2017. After drying at room temperature for 10 d, fruits with encased seeds were sealed in aluminum bags, vacuumized, and stored at −20 C for up to 3 wk to retain their fresh mature status until experiments were initiated, following a protocol described by Baskin and Baskin (Reference Baskin and Baskin2014). Initial tests with freshly harvested material revealed that the 3-wk storage did not affect the maximum germination of the fruits, demonstrating the fresh mature state was preserved during the storage at −20 C.
Germination Assays, Afterripening Storage, and Treatments
Germination was quantified using “isolated seeds” (seeds manually removed from the fruits by mechanically opening the pericarp; Figure 1) and indehiscent fruits (seeds enclosed within pericarps). Three technical replicates, each containing 25 fresh isolated seeds and 25 fruits as biological replicates were assigned to germination assays as follows. Germination assays were carried out under a 12/12 h light regime (white light at ~100 µmol m−2 s−1) at optimum temperature (25/15 C, 12/12 h) for the species studied. Isolated seeds and fruits (seeds within pericarp) were incubated for 28 d, and visible protrusion of the radicle was recorded as the completion of germination (Baskin and Baskin Reference Baskin and Baskin2014; Mamut et al. Reference Mamut, Tan, Baskin and Baskin2014; Tang et al. Reference Tang, Tian and Long2010; Zhou et al. Reference Zhou, Lu, Tan, Baskin and Baskin2015). To determine whether dormancy is released during dry afterripening storage, isolated seeds and fruits were stored in laboratory conditions (25 ± 2 C, 51% relative humidity) for 0 (fresh as the control), 4, 8, 12, and 16 wk. To investigate whether cold stratification releases dormancy, isolated seeds and fruits were incubated in the imbibed state in darkness at 4 C for 0 (fresh as the control), 4, 8, 12, and 16 wk. To investigate how gibberellic acid (GA3; CAS: 77-06-5, A4586, AppliChem GmbH, Darmstadt, Germany) or (±)-abscisic acid (ABA; CAS: 14375-45-2, A1049, Sigma-Aldrich, USA) affects germination, isolated seeds and fruits were incubated without (distilled water as the control) or with a defined hormone concentration dissolved in dimethyl sulfoxide (ca. 0.01% v/v) added.
Water Uptake, Mechanical Constraint, and Chemical Inhibitor Experiments
To investigate whether the pericarp is water permeable or not, water imbibition was compared between fresh isolated seeds and fruits. Three replicates of 20 fresh isolated seeds and three replicates of 10 intact fruits were compared. Each replicate was weighed using an electronic balance and placed on filter paper moistened with distilled water in petri dishes. Before being weighed, seeds were blotted with paper towels to remove excess moisture at 0, 1, 3, 6, 9, 12, and 24 h, and at 2-d intervals thereafter until the final constant mass was achieved, following a methodology described by Mamut et al. (Reference Mamut, Tan, Baskin and Baskin2014). Percentage of increase in mass was calculated as [(Wi − Wd)/Wd)] × 100, where Wi is mass of imbibed seeds within pericarp or fresh isolated seeds and Wd is mass of dry seeds within pericarps or of fresh isolated seeds (Baskin and Baskin Reference Baskin and Baskin2014; Liu et al. Reference Liu, Liu, Ji, Chen and Xu2015; Mamut et al. Reference Mamut, Tan, Baskin and Baskin2014). To test whether the pericarp mechanically constrains germination or not, the following germination tests were compared: (1) The pericarp was completely removed without damaging the seeds (Lu et al. Reference Lu, Zhou, Tan, Baskin and Baskin2015; Mira et al. Reference Mira, Veiga-Barbosa and Pérez-García2015). (2) The whole dispersal unit (fruit) was tested as a control (Hu et al. Reference Hu, Yu, Yang, Wu, Wang and Boelt2010; Liu et al. Reference Liu, Liu, Ji, Chen and Xu2015). (3) The pericarp was mechanically scarified with a razor blade without damaging the seeds to test whether it prevents the protrusion of the radicle or not (Mira et al. Reference Mira, Veiga-Barbosa and Pérez-García2015). This scarification removed a small piece of pericarp layer in the region where the radicle end of the seed is localized. (4) Surface sterilization of fruits was used as another comparison (Sperber et al. Reference Sperber, Steinbrecher, Graeber, Scherer, Clausing, Wiegand, Hourston, Kurre, Leubner-Metzger and Mummenhoff2017). To test whether the pericarp confers a chemical dormancy to constrain germination or not, the following germination tests were compared: (1) The pericarp was completely removed without damaging the seeds (Lu et al. Reference Lu, Zhou, Tan, Baskin and Baskin2015; Mira et al. Reference Mira, Veiga-Barbosa and Pérez-García2015). (2) The whole dispersal unit (seeds within pericarp) was tested as a control (Hu et al. Reference Hu, Yu, Yang, Wu, Wang and Boelt2010; Liu et al. Reference Liu, Liu, Ji, Chen and Xu2015). (3) Scarified fruits with the mechanical constraint completely removed were analyzed to determine whether soluble chemicals would inhibit germination by leach out from the pericarp (Baskin and Baskin Reference Baskin and Baskin2014; Mamut et al. Reference Mamut, Tan, Baskin and Baskin2014). (4) Seeds within the pericarp were washed for 24 h to show whether chemical inhibitors are removed by washing with a large volume of water or not (Hu et al. Reference Hu, Yu, Yang, Wu, Wang and Boelt2010). Moreover, seeds within the pericarp washed in 1 L of de-ionized water for 0 h (nonwashed as the control) were compared with seeds within pericarp washed for 6, 12, 18, and 24 h and fresh isolated seeds (nonwashed) to show the effect of pericarp-mediated chemical inhibitors on germination. Furthermore, fresh and afterripened pericarps 300 mg were washed with 3 ml of distilled water using a shaker at 100 rpm for 6 h, and wash water from fresh pericarp, afterripened pericarp, or previously washed fresh pericarp (rewashed) was applied for germination tests.
Quantification of Endogenous ABA and GA Metabolites
The ratio between ABA (induces dormancy) and bioactive GA (induces germination) controls seed germination (Baskin and Baskin Reference Baskin and Baskin2014; Finch-Savage and Leubner-Metzger Reference Finch-Savage and Leubner-Metzger2006; Née et al. Reference Née, Xiang and Soppe2017). Fresh and afterripened isolated seeds, fresh and afterripened pericarp tissues, fresh pericarp tissues washed for 0 h (nonwashed as the control) were compared with fresh pericarp tissues washed 6, 12, 18, and 24 h to study the levels of endogenous ABA and GA metabolites. For ABA metabolite analysis, plant tissue (approximately 20 mg of each sample’s dry weight) were homogenized and extracted for 1 h in 1 ml ice-cold methanol/water/acetic acid (10/89/1, v/v/v). Internal standard mixtures, containing 20 pmol each of (−)-7′,7′,7′-[2H3]-phaseic acid; (−)-7′,7′,7′-[2H3]-dihydrophaseic acid; (−)-8′,8′,8′-[2H3]-neophaseic acid; (+)-4,5,8′,8′,8′-[2H5]-ABAGE; (−)-5,8′,8′,8′-[2H4]-7′-OH-ABA, and (+)-3′,5′,5′,7′,7′,7′-[2H6]-ABA were added to each of the samples. The homogenates were centrifuged (36,670 × g, 10 min, 4 C) after extraction, and the pellets were then re-extracted in 0.5 ml extraction solvent for 30 min. The combined extracts were purified by solid-phase extraction on Oasis® HLB cartridges (60 mg, 3 ml; Waters, Milford, MA, USA), then evaporated to dryness in a Speed-Vac (UniEquip, Planegg, Germany), and finally analyzed by UPLC-ESI (±)-MS/MS (ultra-performance liquid chromatography-electrospray ionization tandem mass spectrometry) (Turečková et al. Reference Turečková, Novák and Strnad2009). For GA metabolite analysis, sample preparation and analysis were performed according to the method described in Urbanová et al. (Reference Urbanová, Tarkowská, Novák, Hedden and Strnad2013) with some modifications. Briefly, 5-mg freeze-dried seed samples were ground to a fine consistency using 3-mm zirconium oxide beads (Retsch, Haan, Germany) and an MM 301 vibration mill at a frequency of 30 Hz for 3 min (Retsch) with 1 ml of ice-cold 80% acetonitrile containing 5% formic acid as extraction solution. The samples were then extracted overnight at 4 C using a benchtop laboratory rotator (Stuart SB3, Bibby Scientific, Staffordshire, UK) after adding 17 internal gibberellin standards ([2H2]GA1, [2H2]GA3, [2H2]GA4, [2H2]GA5, [2H2]GA6, [2H2]GA7, [2H2]GA8, [2H2]GA9, [2H2]GA15, [2H2]GA19, [2H2]GA20, [2H2]GA24, [2H2]GA29, [2H2]GA34, [2H2]GA44, [2H2]GA51, and [2H2]GA53; purchased from Lewis Mander, Canberra, Australia). The homogenates were centrifuged at 36 670 ×g (10 min, 4 C, using a Beckman Coulter Avanti™ 30, Indianapolis, IN, USA) and 4 C for 10 min and the corresponding supernatants were further purified using reversed-phase and mixed-mode SPE cartridges (Waters) and analyzed by ultra-high-performance chromatography–tandem mass spectrometry (Micromass, Manchester, UK). GA metabolites were detected using the multiple-reaction monitoring mode for the transition of the ion [M–H]− to the appropriate product ion. Masslynx v. 4.1 software (Waters) was used to analyze the data, and the standard isotope dilution method (Rittenberg and Foster Reference Rittenberg and Foster1940) was used to quantify the GA levels.
Data Analysis
One-way ANOVA was conducted to analyze the association between treatments for the water imbibition, the various germination assays, and the levels of ABA and GA metabolites. Data were subjected to one-way ANOVA, with post hoc comparisons made by a Tukey’s honest significant difference test. The rejection threshold for all analyses was P < 0.05. SigmaPlot v. 13 (Systat Software, San Jose, CA, USA) and PRISM v. 7.0a (GraphPad, San Diego, CA, USA) were used to generate graphs of the results.
Results and Discussion
Comparative Germination Analysis of Isolated Seeds and Indehiscent Fruits
The dispersal units of the related invasive, noxious, and weedy Brassicaceae species L. draba and L. appelianum are indehiscent fruits (Figure 1). The aim of the present study was to comparatively investigate the roles of the fruit coat (pericarp) in the dormancy mechanisms and germination biology of these species. To achieve this, the germination of isolated seeds (i.e., seeds manually removed from fruits by opening the pericarp; Figure 1A and B) was compared with germination of indehiscent fruits (Figure 1C and D). Visible emergence of the radicle through all covering layers (endosperm, testa, pericarp) was used as the criterion to score the completion of germination of seed or fruit populations. For isolated seeds, germination was accompanied by testa and endosperm rupture (Figure 1G and H) as described for the seeds of L. sativum (Müller et al. Reference Müller, Tintelnot and Leubner-Metzger2006). For the indehiscent fruits of L. draba, the tight encasement of the seeds by the pericarp did not allow the seeds to germinate within the fruits. Instead the fruit itself eventually completed germination with visible radicle emergence through all the covering layers of the seed (endosperm, testa) and fruit (pericarp) and visible pericarp rupture (Figure 1I). The pericarp rupture and visible radicle protrusion next to the tip of the L. draba fruit is therefore mechanically very similar to the fruit germination of L. didymum (Sperber et al. Reference Sperber, Steinbrecher, Graeber, Scherer, Clausing, Wiegand, Hourston, Kurre, Leubner-Metzger and Mummenhoff2017). For L. appelianum fruits, the seeds germinated within the fruits and subsequent embryo expansion eventually led to radicle emergence through the pericarp (Figure 1J).
The objectives of the first set of experiments were to identify the seed dormancy class (Baskin and Baskin Reference Baskin and Baskin2004; Finch-Savage and Leubner-Metzger Reference Finch-Savage and Leubner-Metzger2006; Willis et al. Reference Willis, Baskin, Baskin, Auld, Venable, Cavender-Bares, Donohue and Rubio de Casas2014) and to reveal the role of the pericarp. Therefore, the germination of freshly harvested mature fruit and isolated seed populations of L. draba and L. appelianum were compared with their germination responses in the afterripened state (Figure 2; Supplementary Figure S2) and their responses to cold stratification (Figure 2) and to GA treatment (Figure 3). Fresh isolated seed populations germinated at a high percentage (ca. 90%) in both species. The high percentage of germination of isolated seeds was not appreciatively affected by dry afterripening storage (Figure 2A; F(4, 20) = 0.834, P = 0.433), cold stratification (Figure 2B; F(4, 20) = 1.371, P = 0.274), and treatment of isolated seeds with GA3 (Figure 3A; F(4, 10) = 0.06, P = 0.992). It is well established that these treatments release physiological dormancy (Baskin and Baskin Reference Baskin and Baskin2014; Finch-Savage and Leubner-Metzger Reference Finch-Savage and Leubner-Metzger2006; Willis et al. Reference Willis, Baskin, Baskin, Auld, Venable, Cavender-Bares, Donohue and Rubio de Casas2014). The isolated seeds of both the U.S. (KM 1269) and the Austrian (KM 1568) L. draba accessions germinated to a high percentage (ca. 90%) independent of the harvest year (2014 to 2015, 2015 to 2016, or 2016 to 2017) and state (fresh vs. afterripened) (Supplementary Figure S2 and corresponding statistics in Supplementary Table S1). Because both freshly harvested and afterripened isolated seed populations of L. draba andL. appelianum germinated at a high percentage and were not affected by these treatments, we conclude that these seeds are physiologically ND at maturity.
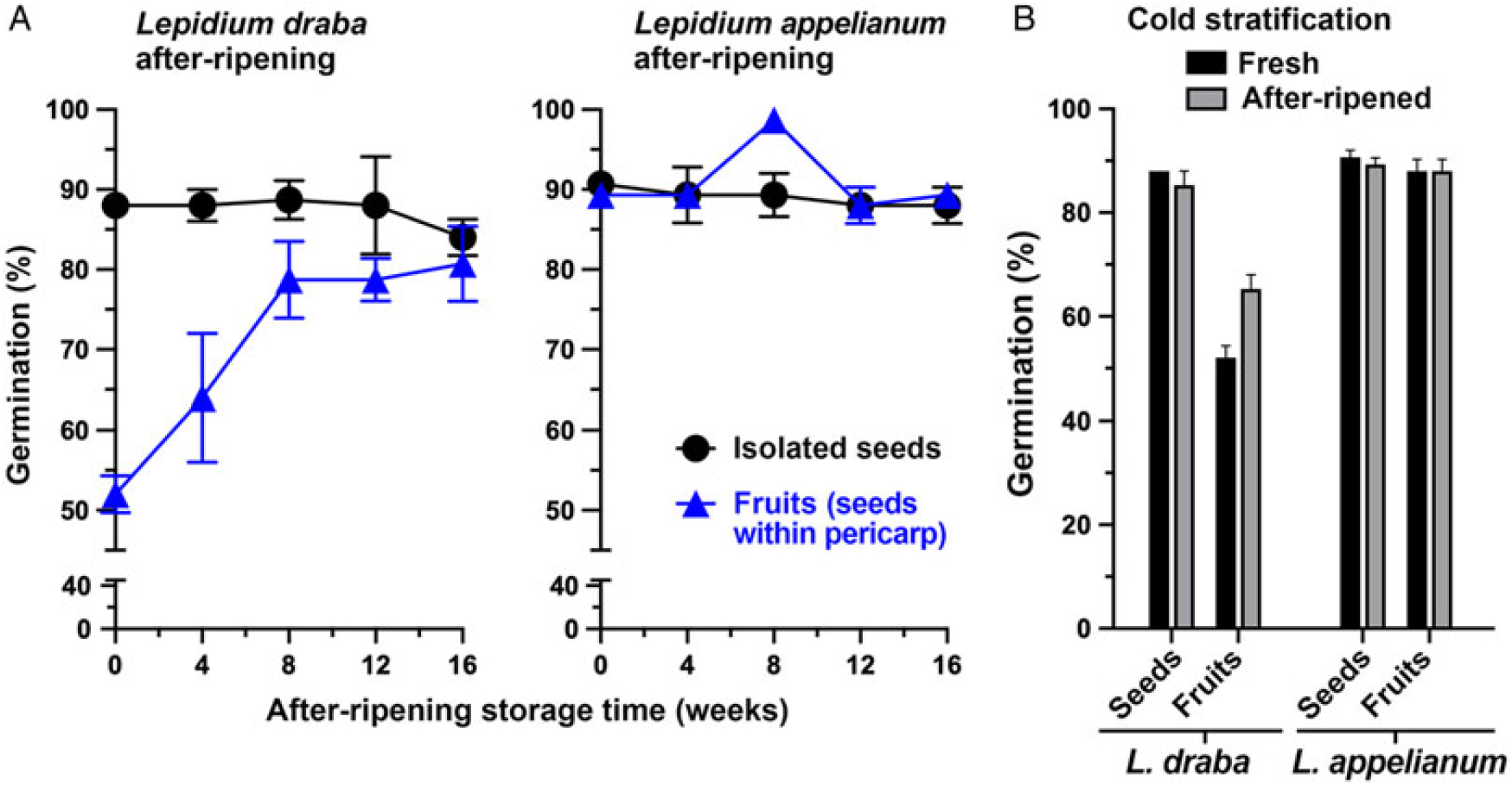
Figure 2. The effect of afterripening and cold stratification on the germination of Lepidium draba and Lepidium appelianum isolated seeds and indehiscent fruits (seeds within pericarp). (A) The effect of afterripening (dry) storage at room temperature and humidity. (B) The effect of cold stratification in the imbibed state under dark conditions in a refrigerator (4 C). Mean values ± SE (N = 3 × 25) of accessions KM 1296 and KM 1754 (2014 to 2015 harvest) at optimal germination assay conditions (12/12-h light regime at 25/15 C day/night for 28 d) are presented.
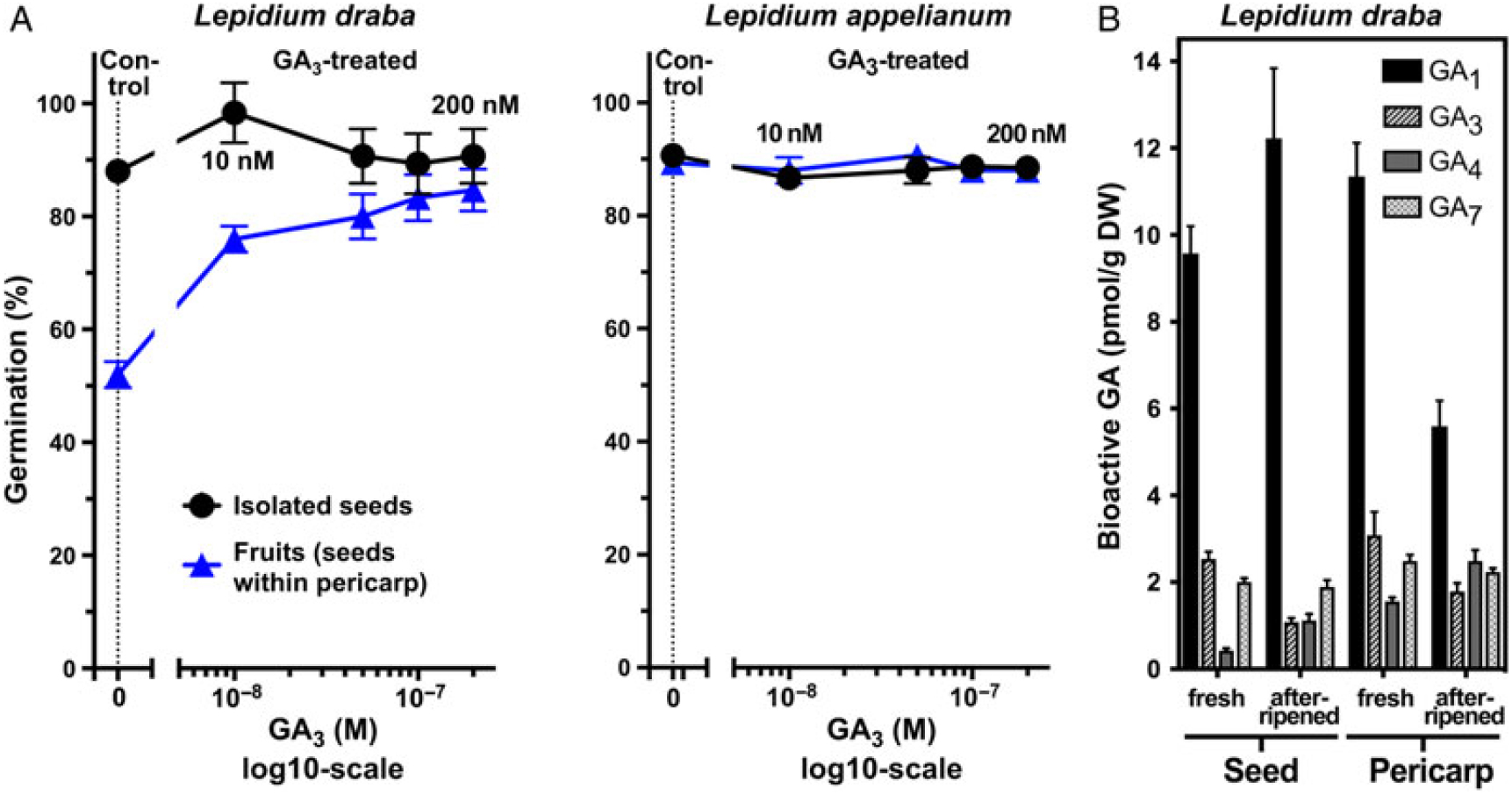
Figure 3. The effect of gibberellic acid (GA3) treatment on the germination of Lepidium draba and Lepidium appelianum fresh and afterripened seeds and fruits and the levels of endogenous bioactive gibberellins (GA). (A) Dose response for the effects of exogenous GA3 on germination responses of fresh isolated seeds and fruits (seeds within pericarp). Mean values ± SE (N = 3 × 25) of accessions KM 1296 and KM 1754 (2014 to 2015 harvest) at optimal germination assay conditions (12/12-h light regime at 25/15 C day/night for 28 d) are presented. (B) Endogenous levels of bioactive gibberellins (GA1, GA3, GA4, and GA7) in fresh and afterripened seeds and pericarps of L. draba. N = 4 × 20 mg (dry weight, DW) of seed/pericarp are presented.
Analysis of endogenous GA metabolite levels in fresh and afterripened seed and pericarp tissues in L. draba revealed the presence of the bioactive gibberellins GA1, GA3, GA4, and GA7 (Figure 3B), as well as their precursors and inactive metabolites (unpublished data). The dry seed and pericarp contained nanogram quantities of these GA metabolites, with GA1 being the dominant bioactive GA. No striking differences were evident between fresh and afterripened seeds (Figure 3B). The finding that fresh and afterripened seeds do not appreciably differ in the levels of bioactive GAs further supports the conclusion that L. draba seeds are ND.
Our study species, L. draba and L. appelianum, are highly invasive weeds throughout the Middle East, Asia, Australia, New Zealand, Canada, and the western United States (Chipping and Bossard Reference Chipping, Bossard, Bossard, Randall and Hoshovsky2000; Gaskin Reference Gaskin2006; Gaskin et al. Reference Gaskin, Zhang and Bon2005; McInnis et al. Reference McInnis, Kiemnec, Larson, Carr and Sharratt2003) and are dispersed in disturbed areas, including roadsides. Analysis of their global distribution using the Global Biodiversity Information Facility (https://www.gbif.org/) database suggests that their main occurrence in the western part of North America is expanding toward the eastern regions for both species (Supplementary Figure S1). Bani-Aameur and Sipple-Michmerhuizen (Reference Bani-Aameur and Sipple-Michmerhuizen2001) and Presotto et al. (Reference Presotto, Poverene and Catamutto2014) reported that the lack of dormancy in weedy species increases the survival ability of the species, because germination and early seedling growth are the most critical factors for species establishment. Therefore, higher germination and seedling recruitment have been recognized as being among the major factors promoting naturalization success of invasive species (Fernández-Pascual et al. Reference Fernández-Pascual, Jiménez-Alfaro, Caujapé-Castells, Jaén-Molina and Díaz2013; Mandák Reference Mandák2003; Udo et al. Reference Udo, Tarayre and Atlan2016; Walck et al. Reference Walck, Hidayati, Dixon, Thompson and Poschlod2011). Rezvani and Zaefarian (Reference Rezvani and Zaefarian2016) found that isolated seeds of L. draba are light requiring and that GA3 treatment can replace the light requirement to trigger germination. Similar to L. draba and L. appelianum (Figures 1–3), other Brassicaceae species, including Noccaea papillosa (Boiss.) F. K. Mey (Kirmizi Reference Kirmizi2017), L. sativum (Graeber et al. Reference Graeber, Linkies, Steinbrecher, Mummenhoff, Tarkowská, Turečková, Ignatz, Sperber, Voegele, de Jong, Urbanová, Strnad and Leubner-Metzger2014), and L. didymum (Sperber et al. Reference Sperber, Steinbrecher, Graeber, Scherer, Clausing, Wiegand, Hourston, Kurre, Leubner-Metzger and Mummenhoff2017), produce ND seeds. In contrast to this, other Brassicaceae weedy species, for example, Coincya rupestris subsp. leptocarpa (Gonz. Albo) Leadlay, Coincya rupestris subsp. rupestris Porta & Rigo ex Rouy (Copete et al. Reference Copete, Herranz and Ferrandis2005), clasping pepperweed (Lepidium perfoliatum L.) (Tang et al. Reference Tang, Tian and Long2010), L. papillosum (Graeber et al. Reference Graeber, Voegele, Büttner-Mainik, Sperber, Mummenhoff and Leubner-Metzger2013), A. thaliana (Baskin and Baskin Reference Baskin and Baskin2014), Chorispora sibirica (L.) de Candolle, Syrian mustard [Euclidium syriacum (L.) W. T. Alton], Goldbachia laevigata (Marschall von Bieberstein) de Candole, Spirorhynchus sabulosus Karelin & Kirilov,Sterigmostemum fuhaiense H. L. Yang, Tauscheria lasiocarpa Fischer ex de Candolle (Lu et al. Reference Lu, Zhou, Tan, Baskin and Baskin2015), and Isatis violascens Bunge (Zhou et al. Reference Zhou, Lu, Tan, Baskin and Baskin2015) produce PD seeds (Baskin and Baskin Reference Baskin and Baskin2014).
Distinct Roles of the Pericarp in Lepidium draba and Lepidium appelianum Fruit Germination
Figure 2 shows that while seeds of both species are physiologically ND and germinate readily, the two species differ in the germination responses of their indehiscent fruits. In populations of L. appelianum fruits, the seeds germinated within the fruits and subsequent radicle expansion led to emergence through the pericarp (Figure 1J) of ca. 90% already in the fresh mature state (Figure 2A). In contrast to this, populations of L. draba fresh fruits exhibited pericarp rupture and visible radicle emergence (Figure 1I), with only ca. 50% of the fruits completing germination (Figure 2A). Interestingly, afterripening for 16 wk resulted in ca. 90% fruits germinating in L. draba with visible pericarp rupture (Figure 2A). This finding for L. draba was evident for both the U.S. (KM 1269) and the Austrian (KM 1568) accessions and consistent over the harvest years (2014 to 2015, 2015 to 2016, 2016 to 2017) (Supple-mentary Figure S2 and corresponding statistics in Supplementary Table S1). As with afterripening, treatment with GA3 also increased the maximum fruit germination percentage of L. draba from ca. 50% to ca. 90% (Figure 3A). In contrast to this, cold stratification had no appreciable effect on the maximum germination percentage of L. draba (Figure 2B). When the germination responses of fruits and isolated seeds (Figures 2 and 3; Supplementary Figure S2) are compared, it is clear that in L. appelianum the pericarp has no effect on the maximum germination percentage of the population, but in L. draba it inhibited the germination of about half of the population. We conclude that while both species have ND seeds, the roles of the pericarps differ. In L. appelianum, the pericarp does not affect the germination capacity, while the indehiscent fruits of L. draba have pericarp-imposed dormancy. Interestingly, this pericarp-imposed dormancy of the L. draba indehiscent fruits can be released by afterripening and by GA3 treatment (Figures 2 and 3).
To further investigate the finding that the pericarp confers coat dormancy in L. draba, while it does not affect the germination in L. appelianum, we compared the patterns of water uptake of fruits and seeds. Figure 4 shows that the water uptake patterns of isolated seeds of both species were very similar, with three typical phases: imbibition (phase 1), plateau (phase 2), and completion of germination by radicle emergence and subsequent growth (phase 3) (Finch-Savage and Leubner-Metzger Reference Finch-Savage and Leubner-Metzger2006). In Figure 4A a comparison of fresh mature fruits of L. draba with fresh mature isolated seeds shows that the pericarp slowed down the water uptake during imbibition (phase 2) and delayed the onset and rate of phase 3 water uptake, which in seeds is associated with radicle emergence and embryo postemergence growth. While all L. draba seeds completed the germination process within ca. 6 d, even after 28 d, the L. draba fruit population had only reached ca. 50%. The results in Figure 4A demonstrate that the L. draba pericarp is water permeable, that is, the fruits do not have physical dormancy, but the pericarp confers coat dormancy in association with slowing down the water uptake and the transition to phase 3 and the completion of fruit germination. In contrast to this, Figure 4B shows that when fruits and isolated seeds of L. appelianum were compared, the pericarp did not appreciably affect the water uptake patterns and permitted maximum germination. We conclude that the pericarp-imposed dormancy of L. draba fruits is caused by some mechanism that decreases the water uptake and inhibits the transition from phase 2 to phase 3 required for the completion of germination.
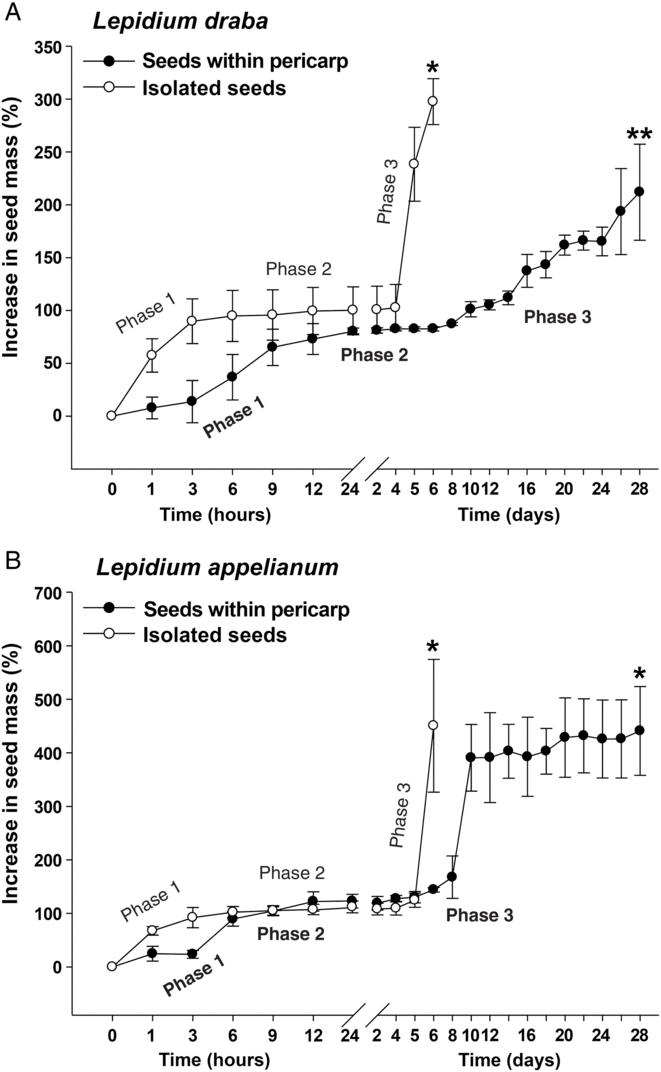
Figure 4. The effects of the pericarp (fruit coat) on the water uptake of (A) Lepidium draba and (B) Lepidium appelianum seeds. A single asterisk refers to the time of full (>90%) completion of germination of fresh isolated seeds or fruits (seeds within pericarp), whereas a double asterisk refers to the maximum germination (52%) due to the pericarp-mediated dormancy of L. draba (see Figure 2A). Isolated seeds and fruits exhibit a typical three-phase pattern of water uptake by seeds: phase 1 (imbibition) is followed by the plateau phase 2 (metabolic activation), and upon endosperm rupture, the radicle emergence is associated with phase 3 (water uptake indicative for the completion of germination). N = 3 × 20 (fresh seeds) of accessions KM 1296 and KM 1754 (2014 to 2015 harvest); N = 3 × 10 for each time point measured (fresh seeds within pericarp).
ABA-mediated Inhibition as Mechanism for the Pericarp-imposed Dormancy in Lepidium draba
To investigate the mechanisms through which the pericarp confers coat dormancy to seeds in L. draba fruits, we conducted scarification and leaching experiments. Figure 5A shows that neither pericarp scarification (i.e., removing a possible mechanical constraint by manually removing the part of the pericarp covering the seed’s radicle end with razor blade) nor pericarp surface sterilization to eliminate possible microbial activity removed the pericarp-imposed dormancy. The germination percentages of mechanically scarified fruits (58%) and surface-sterilized fruits (54%) did not differ significantly from nonsterilized fruits (control, 52%) (F(2, 120) = 3.8, P = 0.086) of L. draba. The treatments did not affect the ca. 90% germination of subsequently isolated L. draba seeds, and the comparison with L. appelianum shows that neither the scarification nor the sterilization treatment had negative effects (Figure 5A). The finding that neither pericarp scarification nor surface sterilization affected the pericarp-mediated dormancy of L. draba strongly suggests that lack of germination is not mechanical in nature and that microbial activity is not involved in its release. It is therefore different from the recent finding in L. didymum that the pericarp-imposed dormancy is caused by a mechanical constraint and the microbial activity of common fungi is involved in the release of the pericarp-imposed dormancy (Sperber et al. Reference Sperber, Steinbrecher, Graeber, Scherer, Clausing, Wiegand, Hourston, Kurre, Leubner-Metzger and Mummenhoff2017). Many other Brassicaceae species, including wild radish (Raphanus raphanistrum L.) (Cousens et al. Reference Cousens, Young and Tadayyon2010), Diptychocarpus strictus (Fischer ex Marschall von Bieberstein) Trautvetter (Lu et al. Reference Lu, Tan, Baskin and Baskin2010), turnipweed [Rapistrum rugosum (L.) All.] (Ohadi et al. Reference Ohadi, Mashhadi and Tavakol-Afshari2011), Lachnoloma lehmannii Bunge (Mamut et al. Reference Mamut, Tan, Baskin and Baskin2014), C. sibirica, E. syriacum, G. laevigata, S. sabulosus, S. fuhaiense, T. lasiocarpa (Lu et al. Reference Lu, Zhou, Tan, Baskin and Baskin2015), and I. violascens (Zhou et al. Reference Zhou, Lu, Tan, Baskin and Baskin2015) seem to have a coat dormancy with a mechanical component similar, at least in part, to the pericarp-imposed mechanical dormancy of L. didymum (Sperber et al. Reference Sperber, Steinbrecher, Graeber, Scherer, Clausing, Wiegand, Hourston, Kurre, Leubner-Metzger and Mummenhoff2017). In contrast to this, in L. appelianum, the pericarp does not confer a coat dormancy at all.
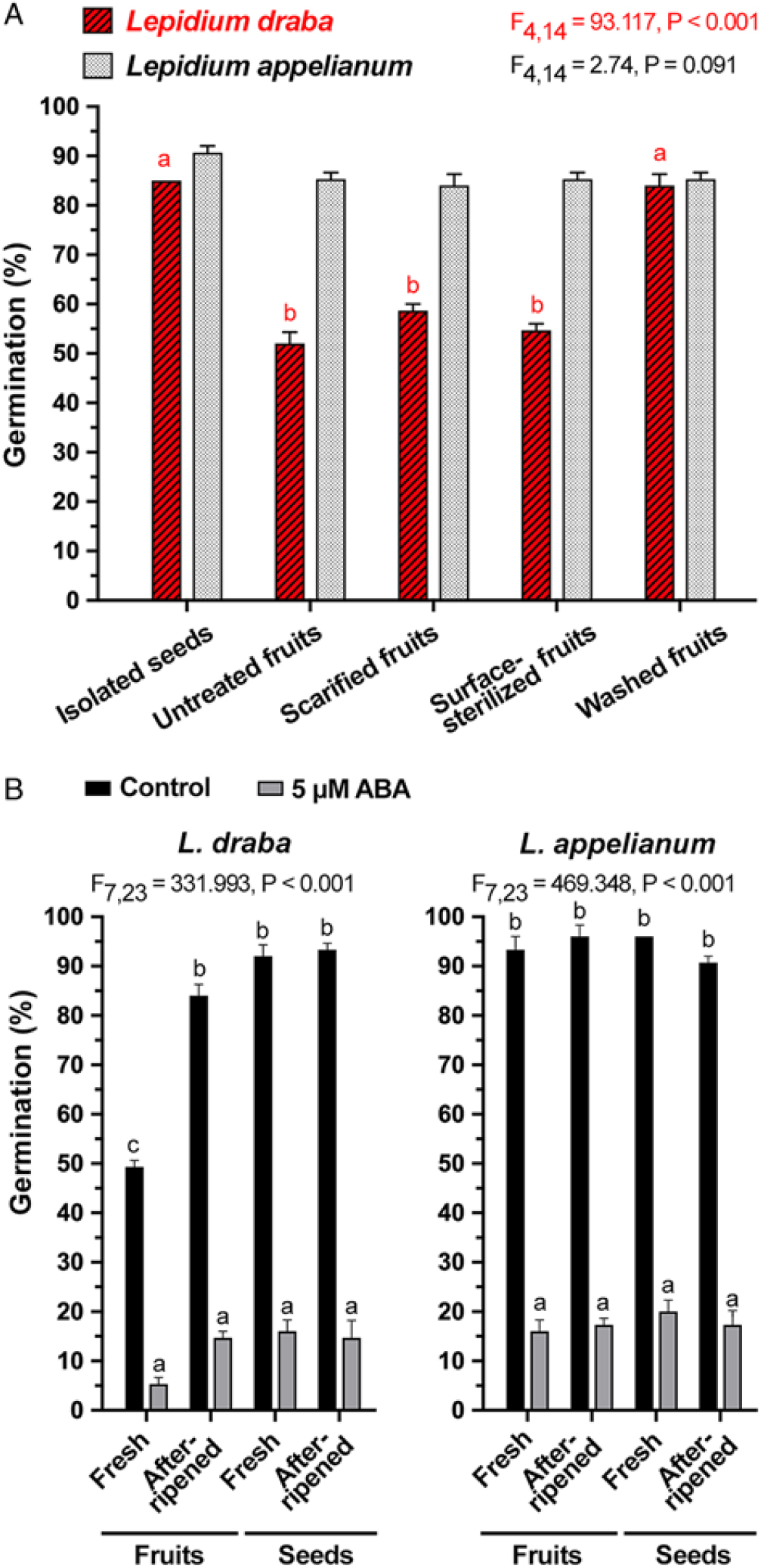
Figure 5. The effects of pericarp scarification, sterilization, washing, and abscisic acid (ABA) treatment on the germination of Lepidium draba and Lepidium appelianum freshly harvested mature fruits. (A) Germination of fresh isolated seeds, untreated fresh fruits (seeds enclosed within untreated pericarp), scarified fresh fruits (seeds enclosed within scarified pericarp, that is, mechanical constraint of pericarp removed by scarification with razor blade), surface-sterilized fresh fruits (seeds enclosed within surface-sterilized pericarp to eliminate microbial activity), and washed fresh fruits (fruits washed for 24 h to remove water-soluble chemical inhibitors) of L. draba and L. appelianum. (B) Germination of fresh and afterripened indehiscent fruits and isolated seeds without (control) and with addition of 5 µM ABA. Mean values ± SE (N = 3 × 25) of accessions KM 1296 and KM 1754 (2014 to 2015 harvest) at optimal germination assay conditions (12/12-h light regime at 25/15 C day/night for 28 d) are presented. Different letters (a, b) designate significantly different mean values as determined by Tukey’s pairwise multiple-comparison test (P < 0.05).
In contrast to pericarp scarification, washing of fresh L. draba fruits removed the pericarp-imposed dormancy and caused ca. 90% germination, as is the case for the isolated ND seeds of L. draba (Figure 5A). For the ND L. appelianum fruits, germination occurs without the washing treatment (Figure 5A). This finding strongly suggests that chemical inhibitors present in the pericarp of fresh L. draba fruits cause the pericarp-imposed dormancy, while such inhibitors are lacking in L. appelianum. Washing may have caused leaching out of the chemical inhibitors from L. draba pericarps, thereby releasing the dormancy. To further quantify the effect of the inhibitors, we added wash water of L. draba pericarps to the germination media of fresh (Figure 6A) and afterripened (Figure 6C) L. draba seeds. The wash water from fresh L. draba pericarps caused a significant delay in the onset of seed germination and in the maximum germination percentages of fresh (Figure 6A) and afterripened (Figure 6C) seeds. In contrast to this, no inhibition of seed germination was obtained with wash water from afterripened or wash water from previously washed fresh pericarps (Figure 6A and C). It is therefore clear that only the fresh L. draba pericarp contains water-soluble germination inhibitors that leach out and thereby inhibit germination.
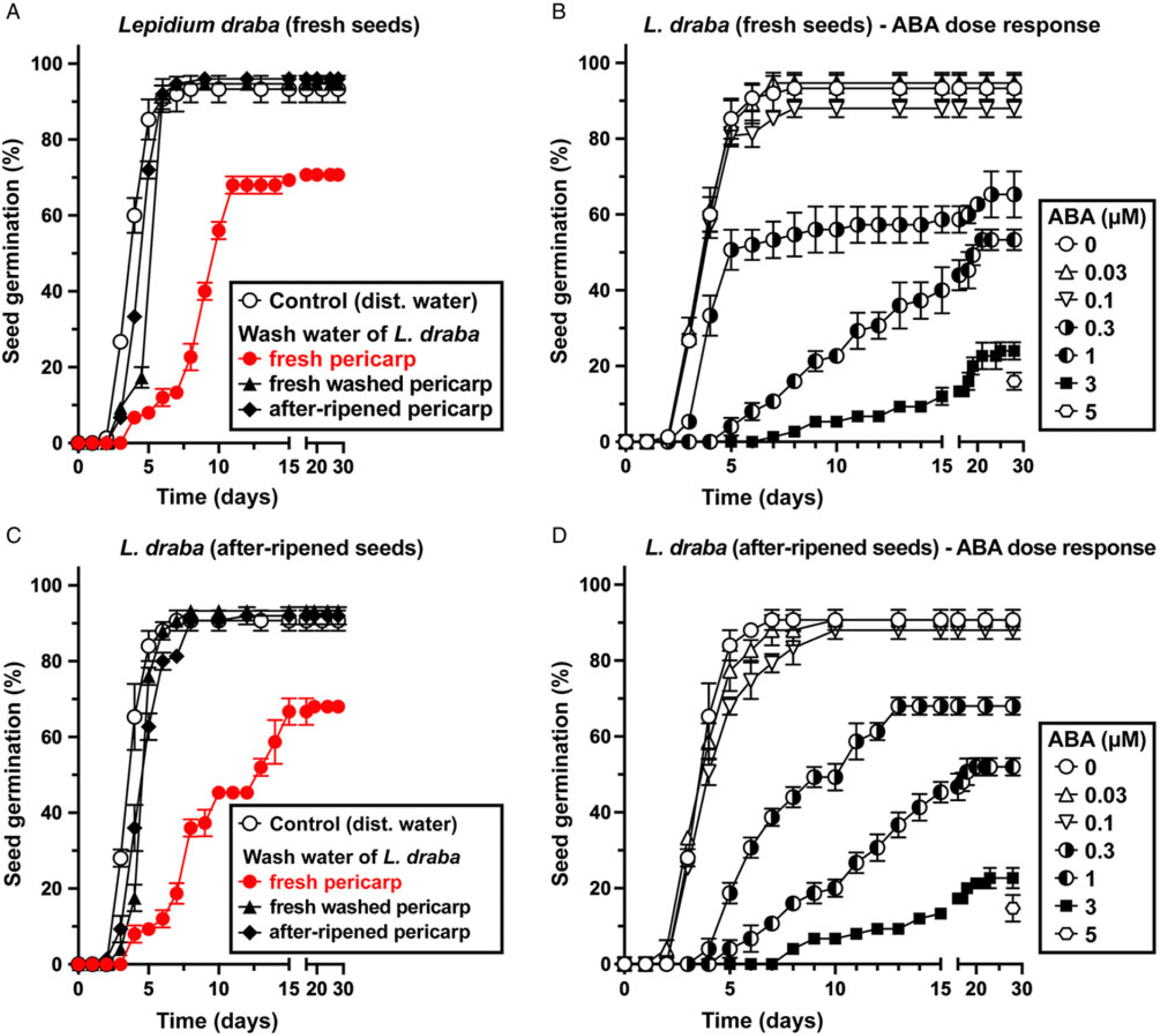
Figure 6. The effect of treatment with abscisic acid (ABA), wash water from fresh pericarp, wash water of washed fresh pericarp, and wash water of afterripened pericarp on the germination kinetics of Lepidium draba isolated seeds. (A) The effect of wash water from L. draba pericarp on the germination of L. draba fresh seeds. (B) Germination dose response of L. draba fresh seeds incubated with different ABA concentrations applied. (C) The effect of wash water from L. draba pericarp on the germination of L. draba afterripened seeds. (D) Germination dose response of L. draba afterripened seeds incubated with different ABA concentrations applied. Mean values ± SE (N = 3 × 25) of accessions KM 1296 and KM 1754 (2014 to 2015 harvest) at optimal germination assay conditions (12/12-h light regime at 25/15 C day/night for 28 d) are presented. Pericarp tissues weighing 300 mg were washed with 3 ml of distilled water using a shaker at 100 rpm for 6 h to obtain the pericarp wash water applied in the germination assays.
To investigate whether pericarp-released ABA is involved in mediating the pericarp-imposed dormancy of L. draba, we initially compared the germination responses to treatment with ABA of fresh and afterripened fruits and isolated seeds of both species. Treatment with 5 µM ABA inhibited the germination of fruits and seeds of both species in both physiological stages (Figure 5B). Fresh and afterripened seeds of both species seem to be equally sensitive to inhibition by ABA. Interestingly, seeds in fresh L. draba fruits were more sensitive to the inhibition compared with those in fresh L. appelianum fruits (Figure 5B). To precisely quantify the seed sensitivities, we conducted dose–response experiments for the ABA treatment with fresh (Figure 6B) and afterripened (Figure 6D) L. draba seeds. These results demonstrated that increasing ABA concentrations caused a similar delay in the onset of the completion of seed germination of fresh and afterripened L. draba seeds (Figure 6). Increasing ABA concentrations also caused a decrease in the maximum germination percentages reached after ca. 1 mo of incubation of the seed populations. This quantification demonstrated that the fresh and afterripened L. draba seeds had the same ABA sensitivity (Figure 7A). A comparison of these ABA sensitivity values with the results obtained with the wash water of fresh pericarp (Figure 7B) revealed that its inhibitory effect corresponds to a ca. 0.3 µM ABA concentration in the incubation medium (Figure 7).
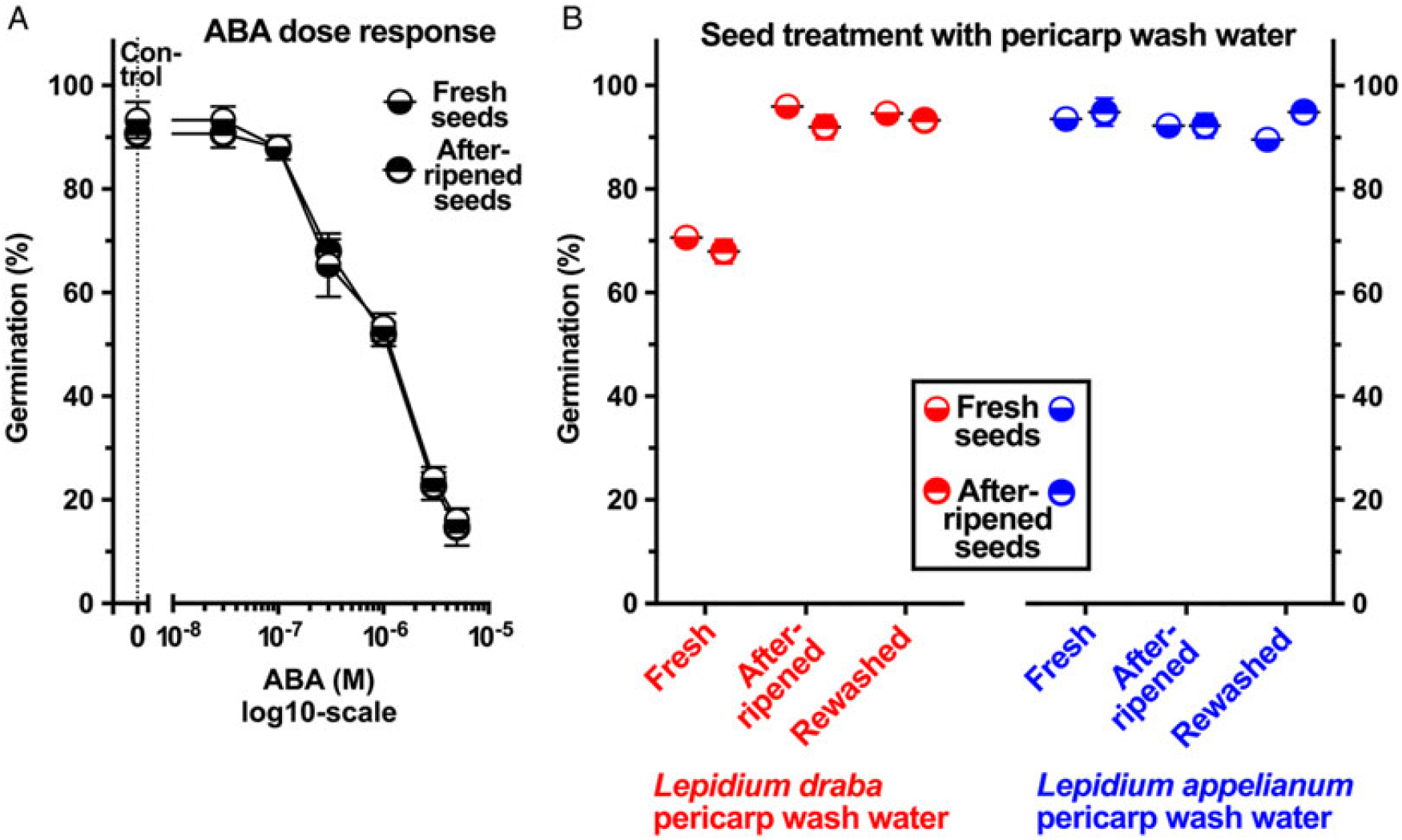
Figure 7. The effect of exogenous abscisic acid (ABA), wash water from Lepidium draba pericarp (fresh, fresh-washed, and afterripened) on the germination of L. draba fresh and afterripened isolated seeds. (A) Germination dose-response of L. draba seeds incubated with different ABA concentrations. (B) The effect of wash water from pericarp on the germination of L. draba seeds. Wash water of fresh L. draba pericarp inhibits at a level similar to 0.3 µM ABA. Lepidium appelianum pericarp does not contain ABA or other water-soluble compounds that may inhibit germination. Mean values ± SE (N = 3 × 25) of accessions KM 1296 and KM 1754 (2014 to 2015 harvest) at optimal germination assay conditions (12/12-h light regime at 25/15 C day/night for 28 d) are presented. Pericarp tissues of 300 mg were washed with 3 ml of distilled water using a shaker at 100 rpm for 6 h to obtain the pericarp wash water applied in the germination assays.
Germination and dormancy are controlled by the hormonal balance between promoting GAs and inhibiting ABA (Finch-Savage and Leubner-Metzger Reference Finch-Savage and Leubner-Metzger2006). To investigate whether ABA is involved in the pericarp-mediated dormancy of L. draba, we analyzed the endogenous hormone levels of fresh and afterripened L. draba seeds, pericarps, and fruits (Figure 8). In contrast to the low GA levels, fresh and afterripened dry seeds of L. draba contained equally high levels of ABA. Interestingly, the ABA content of L. draba fresh mature pericarp was more than 20-fold higher compared with the afterripened pericarp (Figure 8A). Remarkably, when we calculated this, the pericarp ABA content will upon pericarp washing lead to a ca. 0.1 µM ABA concentration in the wash water of fresh L. draba pericarps and to a ca. 0.005 µM ABA concentration in the wash water from afterripened pericarp. Compared with the ABA dose responses (Figure 7A), only the concentration from the fresh pericarp would inhibit germination, and this is exactly what we observed (Figure 7B). In agreement with the ABA in the pericarp playing a role in the chemical dormancy of L. draba fruits, the increased germination percentages of washed fruits were associated with a decrease of the ABA content during the washing process (Figure 8B). Analysis of the ABA catabolites demonstrates that these also decrease during the washing process (Supplementary Table S2) and that the classical 8′-hydroxylation pathway via phaseic acid and dihydrophaseic acid plays a major role in ABA degradation (Turečková et al. Reference Turečková, Novák and Strnad2009). Further, the high germination proportion of afterripened fruits was associated with a low ABA content (Figure 8B), and afterripened fruits did not germinate when imbibed in the presence of ABA (Figure 8B). We therefore conclude that fruit washing and afterripening released chemical dormancy by eliminating ABA from the pericarp by leaching and/or degradation.

Figure 8. The effect of afterripening and washing on the abscisic acid (ABA) and gibberellin (GA) levels of Lepidium draba fruits. (A) Endogenous levels of ABA and bioactive GAs in fresh and afterripened dry seeds and pericarps. (B) ABA and bioactive GA levels during washing of fresh L. draba fruits, as compared with afterripened fruits, and with the resultant maximum germination responses presented. Mean values ± SE (N = 3 × 25) of accessions KM 1296 and KM 1754 (2014 to 2015 harvest) at optimal germination assay conditions (12/12-h light regime at 25/15 C day/night for 28 d) are presented. N = 4 × 20 mg (dry weight, DW) of seed/pericarp for ABA and bioactive GA analysis. For a detailed statistical analysis of the ABA contents and their catabolites, see Supplementary Table S2.
As for L. draba, but not L. appelianum, pericarp-mediated chemical dormancy is suggested to occur in crop and weed species of Brassicaceae, including R. raphanistrum (Cheam Reference Cheam1986; Mekenian and Willemsen Reference Mekenian and Willemsen1975), L. lehmannii (Mamut et al. Reference Mamut, Tan, Baskin and Baskin2014), and Leptaleum filifolium (Willd.) DC. (Lu et al. Reference Lu, Tan, Baskin and Baskin2017). Chemical inhibitors that leach out from the pericarp are thought to cause seed dormancy and can be removed by washing intact fruits with a large volume of water (Baskin and Baskin Reference Baskin and Baskin2014; Hu et al. Reference Hu, Yu, Yang, Wu, Wang and Boelt2010; Liu et al. Reference Liu, Liu, Ji, Chen and Xu2015; Mamut et al. Reference Mamut, Tan, Baskin and Baskin2014). In general, the pericarp can inhibit germination by (1) physical means, that is, inhibition of water imbibition (Cousens et al. Reference Cousens, Young and Tadayyon2010) and reduction of gas exchange (Adkins et al. Reference Adkins, Bellairs and Loch2002; Hu et al. Reference Hu, Wang and Wu2009); (2) mechanical means, that is, mechanical resistance on the radicle protrusion is imposed by the pericarp (Baskin and Baskin Reference Baskin and Baskin2014; Hu et al. Reference Hu, Yu, Yang, Wu, Wang and Boelt2010; Sperber et al. Reference Sperber, Steinbrecher, Graeber, Scherer, Clausing, Wiegand, Hourston, Kurre, Leubner-Metzger and Mummenhoff2017); and/or (3) chemical inhibitors residing inside the fruit coat inhibit germination (this study; Mamut et al. Reference Mamut, Tan, Baskin and Baskin2014; Sari et al. Reference Sari, Oguz and Bilgic2006). In the Brassicaceae, physical dormancy has not been recorded to date (Baskin and Baskin Reference Baskin and Baskin2014), and the possible dormancy types for fruits as dispersal units in this family are either nondormancy and/or pericarp-mediated mechanical (Cousens et al. Reference Cousens, Young and Tadayyon2010; Ohadi et al. Reference Ohadi, Mashhadi and Tavakol-Afshari2011; Sperber et al. Reference Sperber, Steinbrecher, Graeber, Scherer, Clausing, Wiegand, Hourston, Kurre, Leubner-Metzger and Mummenhoff2017) and chemical dormancy (this study; Cheam Reference Cheam1986; Mamut et al. Reference Mamut, Tan, Baskin and Baskin2014; Mekenian and Willemsen Reference Mekenian and Willemsen1975).
Conclusions and Future Research
Weeds compete with crops for water and nutrients, causing significant yield reduction (Bijanzadeh et al. Reference Bijanzadeh, Naderi and Behpoori2010; Olorunmaiye and Olorunmaiye Reference Olorunmaiye and Olorunmaiye2009). Sequestration of these resources by weeds results in vigorous growth, increased seed production, and ease of weed population establishment, all of which have direct impact on crop agriculture and crop yield (Korres Reference Korres2005). This is particularly true for L. draba, an invasive and agronomically problematic weed in western United States and Canada (Al-Shehbaz and O’Kane Reference Al-Shehbaz and ÓKane2002; Gaskin Reference Gaskin2006). Pericarp-mediated chemical dormancy in L. draba plays a critical role in the weediness of this species, because the germination biology is controlled by the dormancy mechanism. Dormancy has a crucial role in the survival of the species in determining the germination timing. Dormancy in weed seedbanks cycles throughout the season, with soil temperatures and moisture providing the key environmental factors to regulate dormancy mechanisms to time germination in variable field environments (Finch-Savage and Footitt Reference Finch-Savage and Footitt2017; Walck et al. Reference Walck, Hidayati, Dixon, Thompson and Poschlod2011). Dormant weed seedbanks could be greatly depleted by stimulating early-season germination and then killing the young seedlings (Westwood et al. Reference Westwood, Charudattan, Duke, Fennimore, Marrone, Slaughter, Swanton and Zollinger2018). Based on our findings, 2 to 3 mo of afterripening or 1 d of fruit washing eliminated the ABA in the pericarp and thereby released the pericarp-mediated dormancy of L. draba. In the soil, this dormancy release would therefore occur slowly in dry conditions and quickly in very wet conditions. Because the pericarp is dead tissue, the removal of this ABA- and pericarp-mediated primary dormancy mechanism would be irreversible. Ultimately, corresponding weather conditions would affect the timing of weed germination and subsequent seedling emergence. The timing of these events constitutes major traits that determine the success of a weed in agricultural ecosystems (Cousens and Mortimer Reference Cousens and Mortimer1995), and understanding seed germination timing under natural conditions is therefore crucial to weed management (Karimmojeni et al. Reference Karimmojeni, Taab, Rashidi and Bazrafshan2014). This point becomes particularly important in the case of our study species L. draba, which is listed as an “invasive species” (Al-Shehbaz and O’Kane Reference Al-Shehbaz and ÓKane2002; Gaskin Reference Gaskin2006). The success of future weed management strategies targeting this weed will depend on knowledge of its biological, phenological, and reproductive characteristics and population dynamics. An understanding of the weed establishment time within a particular cropping system enables timely implementation of control strategies (Karimmojeni et al. Reference Karimmojeni, Taab, Rashidi and Bazrafshan2014).
Author ORCIDs
Said Mohammed https://orcid.org/0000-0002-6717-3796; Veronika Turečková https://orcid.org/0000-0001-8519-805X; Danuše Tarkowská https://orcid.org/0000-0003-1478-1904; Miroslav Strnad https://orcid.org/0000-0002-2806-794X; Klaus Mummenhoff https://orcid.org/0000-0002-8449-1593; Gerhard Leubner-Metzger https://orcid.org/0000-0002-6045-8713.
Acknowledgments
This study was supported by the Deutsche Forschungsgemeinschaft, DFG (MU 1137/8-2) to K.M.; the European Regional Development Fund project Centre for Experimental Plant Biology (no. CZ.02.1.01/0.0/0.0/16_019/0000738) and the Grant Agency of the Czech Republic (18-10349S) to D.T.; and the Biotechnology and Biological Sciences Research Council (BBSRC) to G.L.-M. (BB/M000583/1 and BB/M02203X/1). We thank John Gaskin (USDA) for providing the L. appelianum accession (KM 1754), Ulrike Coja for technical assistance, and Samik Bhattacharya for critical discussion. No conflicts of interest have been declared.
Author contributions
S.M., K.M., and G.L.-M. planned and designed the research; S.M., D.T., and V.T. performed experiments; D.T., V.T. and M.S. provided analytical tools and conducted the hormone quantification; S.M., K.M., and G.L.-M., analyzed and interpreted the data; S.M., K.M., and G.L.-M. wrote the manuscript; all authors revised and approved the final article.
Data availability statement
All data presented or analyzed are included in this published article or are available from the corresponding authors.
Supplementary material
To view supplementary material for this article, please visit https://doi.org/10.1017/wsc.2019.33