Wheat bran extract (WBE) is a food-grade preparation that is highly enriched in arabinoxylan oligosaccharides (AXOS) and that is produced by enzymic extraction from wheat bran. The AXOS in WBE consist of a backbone of β-1,4-linked d-xylopyranosyl residues (xylose), some of which are mono- or di-substituted at the C(O)2 and/or C(O)3 position with α-l-arabinofuranosyl residues (arabinose)( Reference Swennen, Courtin and Lindemans 1 – Reference Andersson and Aman 4 ). Some of the xylose units in the backbone of AXOS carry glucuronic acid at the C(O)2 position, whereas some of the arabinose units are ester-linked at the C(O)5 position with ferulic acid( Reference Swennen, Courtin and Lindemans 1 – Reference Andersson and Aman 4 ). The AXOS in WBE form a heterogeneous mixture of oligosaccharides differing in degree of polymerisation and degree of substitution of the xylan backbone. Besides AXOS, WBE also contains up to 15 % glucans (mainly β-d-(1,3)(1,4)-linked glucan oligomers) and low levels of proteins, minerals and monosaccharides( Reference Femia, Salvadori and Broekaert 5 ).
AXOS are non-digestible fermentable prebiotic oligosaccharides that selectively stimulate the growth of bifidobacteria in vitro ( Reference Yamada, Itoh and Morishita 6 ), in caeca of chickens( Reference Courtin, Swennen and Broekaert 7 , Reference Courtin, Swennen and Broekaert 8 ), in caeca of rats( Reference Van Craeyveld, Swennen and Dornez 9 ) and in the colon of healthy human volunteers( Reference Cloetens, Broekaert and Delaedt 10 ). The evidence for AXOS having prebiotic activity has been recently reviewed( Reference Broekaert, Courtin and Verbeke 11 ). Moreover, AXOS consumption decreases the excretion of urinary and faecal p-cresol, a marker of intestinal protein fermentation( Reference Cloetens, Broekaert and Delaedt 10 , Reference Hamer, De Preter and Cloetens 12 ). Colonic protein fermentation is often regarded as detrimental to host health, in particular with respect to colon toxicity, mutagenicity and carcinogenicity( Reference Hughes, Magee and Bingham 13 ). Fermentation of proteins by the microbiota produces a series of potentially toxic substances including ammonia, amines, sulfur-containing compounds and phenolic compounds such as p-cresol( Reference Bammens, Evenepoel and Keuleers 14 – Reference Meijers, De Preter and Verbeke 18 ). p-Cresol, a tyrosine metabolite produced in the colon by bacterial fermentation, is relevant to health status since it is considered the prototype of protein-bound uraemic toxins in chronic kidney disease( Reference De Preter, Falony and Windey 19 ).
In addition to causing a decrease in protein fermentation, fermentation of AXOS enhances the production of SCFA in vitro and in rats( Reference Van Craeyveld, Swennen and Dornez 9 , Reference Grootaert, Delcour and Courtin 20 , Reference Sanchez, Marzorati and Grootaert 21 ). SCFA are produced upon saccharolytic fermentation of carbohydrates and are considered to be beneficial to the host( Reference Topping and Clifton 22 ).
Several studies have indicated that SCFA improve the intestinal barrier function and reduce inflammation mainly by inhibition of NFκB activation( Reference Hamer, Jonkers and Venema 23 ). SCFA stimulate epithelial cell proliferation and differentiation in the large bowel in vivo ( Reference Frankel, Zhang and Singh 24 ), and SCFA, butyrate in particular, are the major energy source for the colonocytes( Reference Roediger 25 ). In addition, in vitro and animal studies have shown an anti-carcinogenic potential of increased SCFA production( Reference Wong, de Souza and Kendall 26 ). Butyrate, in particular, has received much attention as a potential chemopreventive agent by inhibiting promotion of neoplastic cells to cancer or by removing degraded cells from the tissue( Reference Young, Hu and Le Leu 27 ). However, SCFA are probably not solely responsible for the anti-carcinogenic potential of wheat dietary fibre as was suggested by Borowicki et al. ( Reference Borowicki, Stein and Scharlau 28 ). SCFA may provide further benefits beyond their contribution to gut health. For example, acetate and propionate can modify hepatic lipid metabolism. Propionate serves as a substrate for gluconeogenesis and may inhibit cholesterol synthesis, whereas acetate is utilised as a substrate for the synthesis of longer-chain fatty acids( Reference Wong, de Souza and Kendall 26 ). A possible role for increased SCFA production on glucose homeostasis is supported by the finding that patients lacking a colon after surgical resection exhibit a reduction in insulin sensitivity( Reference Robertson 29 ). These effects may be due to the fact that SCFA are ligands for G-protein-coupled receptors expressed on adipocytes, enteroendocrine L-cells and immune cells( Reference Tazoe, Otomo and Kaji 30 ). Other studies have reported that SCFA either directly or indirectly activate AMP-activated protein kinase (AMPK) in the liver( Reference Hu, Chen and Xu 31 ). AMPK is a key enzyme in the regulation of energy metabolism, and its activation increases skeletal muscle glucose uptake( Reference Winder and Hardie 32 ) and inhibits hepatic glucose production( Reference Zhang, Zhou and Li 33 ).
Intake of fermentable non-digestible carbohydrates has in some cases been noted to cause undesired gastrointestinal effects, including flatulence, abdominal discomfort and diarrhoea( Reference Grabitske and Slavin 34 ). Additionally, the consumption of fermentable non-digestible carbohydrates, such as inulin and galacto-oligosaccharides, has been associated in some studies, but not all, with a number of other gastrointestinal discomfort effects including acid reflux and heartburn, belching/burping, borborygmi, colic (spasmodic abdominal pain), laxation and nausea( Reference Livesey 35 ). Such responses, though transient, affect the perception of well-being by consumers and, hence, their acceptance of food products containing these fermentable carbohydrates.
The purpose of the present study was to evaluate the effect of WBE on gastrointestinal health parameters in healthy adult volunteers. In the present study, WBE was administered at different dosages and in a larger study population as compared with previous studies( Reference Cloetens, Broekaert and Delaedt 10 , Reference Cloetens, De Preter and Swennen 36 ). Additionally, the effect of WBE administration on colonic carbohydrate fermentation was investigated through measurement of faecal levels of SCFA while previously breath H2 was used to analyse carbohydrate fermentation, and the effect on colonic protein fermentation was analysed through measurement of urinary and serum levels of phenolic compounds. Tolerance to WBE was assessed through self-reported scoring by the subjects on gastrointestinal symptoms. Safety was evaluated by assessing the occurrence of adverse events (AE) and by analysing the effect on haematological and clinical blood chemistry parameters.
Experimental methods
Composition of wheat bran extract
WBE (Brana Vita® 200) was produced from wheat bran by Fugeia NV, using a procedure based on that described by Swennen et al. ( Reference Swennen, Courtin and Lindemans 1 ). WBE was analysed for the content of AXOS, the AXOS average degree of polymerisation, its arabinose:xylose ratio, bound ferulic acid and glucuronic acid, glucose as part of poly/oligosaccharides, mannose as part of poly/oligosaccharides, galactose as part of poly/oligosaccharides, free monosaccharides, moisture, protein and ash by analytical procedures outlined previously( Reference Femia, Salvadori and Broekaert 5 ). Lipid content was measured by a Soxhlet method using petroleum ether extraction according to method ISO 1443 (International Organization for Standardization). The presence of mesophilic bacteria, yeasts, fungi and Salmonella was determined according to methods ISO 4833, ISO 7954, ISO 7954 and ISO 6579/cor1, respectively. The presence of the heavy metals As, Cd, Pb and Hg was determined according to a Eurofins method based on ISO 17 294-1/2.
Table 1 shows the composition of the WBE preparation used in the present study. It consisted of 79·0 % AXOS (on a DM basis), had an average degree of polymerisation of 5 and an arabinose:xylose ratio of 0·19.
Table 1 Characterisation of the wheat bran extract preparation

AXOS, arabinoxylan oligosaccharides; XOS, xylo-oligosaccharides; DP, degree of polymerisation; A:X ratio, arabinose:xylose ratio.
Subjects
Based on the dataset of an earlier human intervention trial with WBE( Reference Cloetens, Broekaert and Delaedt 10 ), an evaluable sample size of forty was expected to provide 80 % power (two-sided, α = 0·05) for detecting a statistically significant difference in faecal bifidobacteria levels (the primary outcome variable of the present study) between WBE treatment and placebo treatment. Based on this power analysis, it was decided to recruit approximately sixty volunteers for the present trial. A total of sixty-three healthy subjects (thirty women and thirty-three men, mean age 42 (sd 17) years, mean BMI 23·3 (sd 3·2) kg/m2, all of Caucasian ethnicity) participated in the study. The population encompassed healthy adult subjects of different age groups: twenty-five individuals were aged between 18 and 30 years, five were aged 31–40 years, eight were aged 41–50 years, fourteen were aged 51–60 years and eleven were aged 61–85 years. Exclusion criteria were using a low-energy diet or other extreme dietary habits in the 6 weeks before the start of the clinical trial, intake of antibiotics in the 3 months before the start of the clinical trial, intake of medication or dietary supplements influencing gastrointestinal tract processes in the 2 weeks before the start of the clinical trial, abdominal surgery in the past (with the exception of appendectomy), serious illness (defined as more than 2 weeks unable to work) in the 3 months before the start of the clinical trial, complete anaesthetics in the month before the start of the clinical trial, history of chronic gastrointestinal conditions such as inflammatory bowel disease and irritable bowel syndrome, allergy to wheat products, coeliac disease, alcohol abuse, or smoking of more than five cigarettes per d. Female volunteers were excluded if pregnant or lactating. During the study, the subjects ate their usual diet, but were asked to have a regular eating pattern (three meals per d). The intake of food substances containing probiotics and/or prebiotics was forbidden. At the time of inclusion, all subjects were informed about pro- and prebiotics and the food products containing them. The subjects were asked to read food labels carefully to check the content for the addition of pro- and/or prebiotics. The present study was conducted according to the guidelines laid down in the Declaration of Helsinki and all procedures involving human subjects were approved by the Ethics Committee of the University Hospital UZ Leuven, Belgium (approval no. ML5282). Written informed consent was obtained from all subjects. Compliance was assessed by inquiry and by counting the returned bottles at the end of each intervention. Non-compliance was defined as not taking 100 % of the required drinks during at least 16 of the 21 d treatment period and/or not taking 100 % of the required drinks during at least 2 of the 3 last days before stool collection.
Study design
Figure 1 presents a schematic overview of the randomised, placebo-controlled, double-blind, cross-over study. The study started with a 1-week run-in period, followed by three 3-week treatment periods with 2-week washout periods in between two consecutive treatment periods. Each subject underwent three treatment periods. During the treatment periods, the following treatments were applied, yet not necessarily in this order: placebo treatment; treatment with WBE at 3 g/d (low WBE dose, which equals 2·4 g AXOS/d); and treatment with 10 g WBE/d (high WBE dose, which equals 8 g AXOS/d). Clinic visits took place at the end of the run-in period and at the end of each treatment period. WBE and placebo were administered as non-carbonated soft drinks, of which the volunteer had to drink twice daily 70 ml, once after breakfast and once after dinner. Consumption of the soft drinks took place at the volunteers' home. The WBE-containing soft drinks contained sucrose, colorant, flavour, citric acid and potassium sorbate. The placebo soft drink had the same composition as the WBE-containing soft drink, except that WBE was omitted and that 0·25 g tricalcium phosphate per litre was added to mimic the turbidity of the WBE-containing soft drinks. Subjects were randomly assigned to one of six randomisation groups, each randomisation group differing in the treatment sequence by which the three types of drinks were to be consumed. A list of unique three-digit numbers (volunteer numbers) was generated in a random way. Each volunteer number was linked with the number of a randomisation group. At the screening visit, an eligible subject who gave informed consent was randomly assigned to a volunteer number. Subjects started the study at any of three different inclusion periods, with the starting date of the first inclusion period and the starting date of the third inclusion period being 21 d apart. The study was a double-blind study. The investigators that had direct contact with the subjects were blinded to the treatment since they were unaware of the randomisation groups to which the subjects were assigned. Moreover, the appearance and the taste of the different soft drinks were near identical.

Fig. 1 Schematic representation of the study design. The study started with a 1-week run-in (RI) period, followed by three 3-week treatment periods in which wheat bran extract (WBE) was taken in by the subjects at a dose of 0 g/d (placebo), 3 g/d and 10 g/d (not necessarily in this order). Blood, urine and faecal samples were taken at different, indicated, time points. The subjects completed weekly a questionnaire assessing the occurrence frequency and distress severity of eighteen gastrointestinal symptoms. Additionally, subjects recorded in the bowel habits diary the number of bowel movements and stool consistency during the 1-week RI period and during the last week of each 3-week treatment period. WOP, washout period; GI, gastrointestinal.
Sample collection
Blood samples
At the end of the run-in period and at the end of each 3-week treatment period, fasting serum and plasma samples were collected from those thirty-nine volunteers who had voluntarily provided consent for drawing blood during the study. EDTA, lithium heparin and fluoride/oxalate were used as anti-coagulants. Immediately after blood collection, plasma and serum were transferred to the central laboratory facility of the University Hospitals UZ Leuven for standard analysis of haematology and clinical chemistry parameters. Samples of EDTA-plasma were stored at − 20°C for the analysis of lipid peroxides. Samples of serum were stored at − 80°C for the analysis of p-cresylsulfate.
Urine samples
From the morning of day 5 until the morning of day 7 of the run-in period and from the morning of day 19 until the morning of day 21 of each 3-week treatment period, urine was collected in receptacles containing 1·0 g neomycin. The volume of all urine fractions was measured and the samples were stored at − 20°C until the analysis of p-cresol and phenol. The completeness of each urine collection was estimated from the calculation of the observed:expected creatinine ratio, as proposed by Knuiman et al. ( Reference Knuiman, Hautvast and Van Der Heyden 37 ). The ratio of the observed:expected creatinine excretion was calculated using the formula ((24 h creatinine (mmol) × 113 g/mol)/(24 mg/kg × body weight (kg))) for males and ((24 h creatinine (mmol) × 113 g/mol)/(21 mg/kg × body weight (kg))) for females( Reference Knuiman, Hautvast and Van Der Heyden 37 ). When the ratio was lower than 0·7, the urine collection was considered as incomplete( Reference Knuiman, Hautvast and Van Der Heyden 37 , Reference Murakami, Sasaki and Takahashi 38 ), and the corresponding data were omitted for analysis.
Faecal samples
On the evening of day 5 or during day 6 of the run-in period as well as from the evening of day 19 or during day 20 of each 3-week treatment period, one bowel movement was collected and immediately frozen by the subjects. The faecal sample was kept frozen until delivery at the clinic. After 6 d storage at − 20°C at the clinic, the faecal samples were thawed. Subsequently, 2·5 g were immediately fixed in paraformaldehyde using the procedure described by Franks et al. ( Reference Franks, Harmsen and Raangs 39 ) for microbial analysis by fluorescent in situ hybridisation (FISH). Samples of the faecal samples were stored at − 20°C until the analysis of SCFA, pH and ammonia.
Recording of adverse events
Subjects were asked to record whether they had suffered from a medical condition (differing from the baseline recordings), had to take new medication or had to stop taking previously reported medication. Additionally, at each clinic visit, the subjects were asked these questions. This information was recorded in the appropriate section of the case report form.
Biochemical analyses of blood samples
Haematological parameters, clinical blood chemistry parameters (including liver enzymes and parameters of kidney function), blood lipids, blood vitamins and blood minerals were analysed using standard laboratory techniques for blood analysis. Serum p-cresylsulfate content was determined as described by Meijers et al. ( Reference Meijers, De Preter and Verbeke 18 ).
Microbiological analyses of faecal samples
Faecal samples were analysed for composition of the faecal microbiota. FISH was used to count the number of different bacterial groups in paraformaldehyde-fixed faecal samples. Processing of fixed samples and FISH analysis were performed as described by Franks et al. ( Reference Franks, Harmsen and Raangs 39 ). For total bacterial cell counts, 4′-6-diamidino-2-phenylindole (DAPI) was used. The probes used for group-specific FISH analysis were Bif164 for the genus Bifidobacterium ( Reference Langendijk, Schut and Jansen 40 ), Lab158 for the Lactobacillus–Enterococcus group( Reference Harmsen, Elfferich and Schut 41 ), an equimolar mixture of Chis150 and Clit135 for the Clostridium histolyticum–lituseburense group( Reference Franks, Harmsen and Raangs 39 ), Fprau645 for the Faecalibacterium prausnitzii group( Reference Suau, Bonnet and Stutren 42 ) and Rint623 for the Roseburia–Eubacterium rectale group( Reference Aminov, Walker and Duncan 43 ). Fluorescent cells were counted as described earlier( Reference Harmsen, Raangs and He 44 ). Percentage of bifidobacteria was calculated as the ratio of the absolute amounts of bifidobacteria to the total bacterial cell count.
Biochemical analyses of faecal samples
Concentrations of the SCFA acetate, propionate and butyrate were determined as described by Van de Wiele et al. ( Reference Van de Wiele, Boon and Possemiers 45 ), using 2-methylhexanoic acid as an internal standard. Total SCFA was defined as the sum of acetate, propionate and butyrate.
To determine the faecal pH, a sample of approximately 1 g faeces was homogenised by mixing into 10 % (w/w) demineralised water( Reference Yap, Mohamed and Jamal 46 ). The pH was immediately measured upon homogenisation. Ammonia levels were measured on the same faecal slurries as used for pH determination, following the procedure described by Bremner & Keeney( Reference Bremner and Keeney 47 ).
Biochemical analyses of urine samples
Total p-cresol and phenol contents in urinary samples were determined by GC-MS( Reference De Loor, Bammens and Evenepoel 48 ). Creatinine content of urine was measured using a standard laboratory urinology protocol. p-Cresol and phenol levels were corrected for creatinine content (mg p-cresol/g creatinine; mg phenol/g creatinine).
Recording of gastrointestinal symptoms
A questionnaire assessing gastrointestinal symptoms was established following the guidelines of the US Food and Drug Administration for the development of patient-reported outcomes. The following eighteen gastrointestinal symptoms were scored: diarrhoea, constipation, painful bowel movement, blood in stool, abdominal pain, bloating, abdominal cramps, abdominal stretching, borborygmy, flatulence, burping, acid regurgitation, retrosternal burning, nausea, vomiting, indigestion, difficulty with swallowing and hoarseness/sore throat. Occurrence frequency of the symptoms was graded on a five-step scale ranging from never (0), occasionally (1), frequently (2), nearly always (3) to always (4). Distress severity of the symptoms was graded on a five-step scale ranging from no (0), minimal (1), mild (2), moderate (3) to severe (4) distress, as described by van Munster et al. ( Reference van Munster, de Boer and Jansen 49 ). Subjects were asked to grade these gastrointestinal symptoms weekly during the trial.
Recording of stool parameters
During the run-in period and during the last week of every 3-week treatment period, defecation frequency as well as stool consistency (according to the Bristol Stool Form Scale( Reference Riegler and Esposito 50 )) were recorded daily using appropriate questionnaires. Average defecation frequency was calculated as the number of stools divided by the numbers of days of diary recording. Average stool consistency was calculated as the sum of Bristol Stool Form Scales divided by the number of stools. The composite parameter of defecation frequency and stool consistency (also called the Bristol Composite Measure) was calculated as the sum of Bristol Stool Form Scales divided by the number of days of diary recording( Reference Maki, Carson and Miller 51 ).
Dietary composition
Subjects were asked to record all food and beverage intake of days 3–5 of the run-in period and of days 17–19 of each 3-week treatment period. These data were used to calculate the average daily energy intake (kJ), the average percentage of energy from carbohydrates, the average percentage of energy from lipids and the average percentage of energy from proteins. These calculations were made using the Nubel Food Planner (www.nubel.be). During treatment with 10 g WBE/d, an additional 218 kJ/d (52 kcal/d) and 9·5 g carbohydrates/d were added to the reported data; during treatment with 3 g WBE/d an additional 147·3 kJ/d (35·2 kcal/d) and an additional 7·8 g carbohydrates/d were added to the reported data; during the placebo treatment period an additional 117 kJ/d (28 kcal/d) and 7 g carbohydrates/d were added to the reported data.
Statistical analysis of efficacy variables
All tests of significance were performed at α = 0·05 and two-sided, unless otherwise stated. Assumptions of normality of residuals were investigated for each variable using the Shapiro–Wilk test( Reference Shapiro and Wilk 52 ). When the data were normally distributed, linear mixed models were applied to the raw data as such, except for the microbiota data which were log-transformed before analysis, as is customary for microbiota counts. When the distribution was not approximated by a normal curve, values were ranked before analysis and the linear mixed model was performed on the rank-transformed data( Reference Conover and Iman 53 ). Ties occurring during the rank-transformation were replaced by their average rank. The data to estimate the fixed-effect parameter for the run-in of the response remained unranked.
Evaluations of the effects of treatment on the efficacy variables were completed on an efficacy evaluable (EE) population, defined as all randomised subjects who received placebo and at least one serving of at least one WBE-containing soft drink and who provided at least one post-randomisation outcome data point during each of the two treatment phases. Subjects who took antibiotics during or before the placebo treatment period or subjects who took antibiotics during or before an AXOS treatment period that was not the last treatment period were excluded from the EE population. Additionally, evaluations of the treatment effects on the efficacy variables were also completed on a per protocol (PP) population, a subset of EE subjects who completed the study, were compliant as defined above, who did not take antibiotics or other forbidden medications or products and had no major protocol violations.
Treatment effects as well as treatment × treatment sequence and treatment × inclusion period interaction effects were tested with linear mixed models using conditional F and t tests( Reference Pinheiro and Bates 54 ) (significance at α = 0·1). The single-step Tukey post hoc multiple-comparison procedure was used for the pairwise comparisons of the treatments, using R's multcomp package( Reference Hothorn, Bretz and Westfall 55 ). When no significant interactions were found, treatment differences were evaluated based on the main effect model. In the case of significant interactions, treatment differences were evaluated within each treatment sequence group or within each inclusion period group. Next to that, the overall differences were also analysed by aggregating over the interaction effects in the model. Aggregation over the interaction effects was done by setting up a linear combination of the treatment differences for each treatment sequence group and/or inclusion period group, giving equal weights to either treatment sequence group and/or inclusion period group. Efficacy results are presented for the PP population since no differences between the EE and PP results were observed.
Statistical analysis of safety parameters
The safety population was defined as all randomised subjects who received at least one serving of WBE. Safety was analysed using the emergent AE and the changes in clinical blood parameters in the safety population. An AE was attributed to the treatment period during which the AE started. An AE that started during a washout period was attributed to the treatment preceding the specific washout period. In a first analysis of the changes in clinical blood parameters, the occurrence of adverse shifts in clinical blood parameters was determined. For a given subject, a shift in a blood parameter was considered to be adverse when the blood parameter value after treatment was either (i) outside the normal range while the corresponding baseline value of that subject was within the normal range, (ii) below the normal range while the corresponding baseline value was above the normal range or (iii) above the normal range while the corresponding baseline value was below the normal range. McNemar's test was used to compare differences in AE frequencies and differences in blood parameter adverse shifts among the three treatments (α = 0·017, Sidak correction for three comparisons)( Reference Sidak 56 ). A second analysis of the clinical blood parameters was performed as defined for the efficacy analysis, but applied to the safety population.
Results
Participant characteristics
The disposition of all study participants is presented in Fig. 2. A total of eighty-eight volunteers were screened and sixty-six were randomised to any of the six different randomisation groups. Of these, three volunteers terminated the study prematurely during the run-in period: two volunteers decided to terminate the study for personal non-medical reasons; the third volunteer was asked to terminate the study because of antibiotic intake during the run-in period. Since these volunteers did not receive any WBE serving, they were excluded from the safety population. Hence, sixty-three volunteers were included in the safety population. Of these, fifty-eight were included in the EE population and fifty-seven in the PP population.

Fig. 2 Volunteer disposition. Rand., randomisation; AB, antibiotics; safety pop., safety population; EE pop., efficacy evaluable population; PP pop., per protocol population. * Reason for withdrawal: personal, non-medical. † Reason for withdrawal: intake of AB during run-in period.
Baseline demographics and anthropometric characteristics for the PP population are presented for the six randomisation groups in Table 2. No significant differences could be observed at baseline between the six randomisation groups with respect to sex, age, BMI, faecal bifidobacteria level, defecation frequency, cholesterol content, LDL-cholesterol and TAG content. A significant difference at baseline was observed for HDL-cholesterol content, indicating that randomisation with respect to HDL-cholesterol was suboptimal.
Table 2 Baseline characteristics for the six randomisation groups (Mean values with their standard errors)

* Fisher's exact test for count data test.
† Kruskal–Wallis rank sum test.
‡ One-way ANOVA.
§ n 5.
∥ n 7.
¶ n 34.
Analysis of safety variables
Analysis of emergent adverse events
AE were categorised in eleven categories according to the National Cancer Institute Common Terminology Criteria for Adverse Events v3·0 before the unblinding of the study. Statistical analysis of the AE in the safety population revealed no difference between the three treatments in frequency of any of the different AE categories (P>0·1).
Analysis of haematological and clinical chemistry parameters
In a first analysis, the occurrence of adverse shifts in clinical blood parameters was determined in the safety population. Statistical analysis indicated no significant differences in occurrence frequency of adverse shifts between the three treatments (P>0·1).
A second analysis of the clinical blood parameters was performed as defined for the efficacy analysis, but applied to the safety population (Table 3).
Table 3 Haematological and clinical blood chemistry parameters during a human intervention study following intake of placebo, wheat bran extract (WBE) at 3 g/d or WBE at 10 g/d (Mean values and standard deviations)

MCH, mean corpuscular Hb; MCHC, mean corpuscular Hb concentration; MCV, mean corpuscular volume; MPV, mean platelet volume.
* P1 is the P value of the conditional F test for overall WBE-related significant treatment effects; P2, P3 and P4 are the P values for the comparison between WBE at 10 g/d and placebo, WBE at 10 g/d and WBE at 3 g/d, and WBE at 3 g/d and placebo, respectively.
Conditional F tests showed overall WBE-related significant treatment effects for four parameters: platelet count, lymphocyte percentage, mean corpuscular volume and bicarbonate (P < 0·1). Subsequent pairwise comparisons demonstrated that the only parameter that was affected by WBE treatment as compared with placebo treatment was the lymphocyte percentage in plasma. Lymphocyte percentage was significantly lower after treatment with WBA at 3 g/d (34·3 %) than after the placebo treatment period (36·2 %) (P = 0·045). However, the lymphocyte percentage in plasma after treatment with WBA at 10 g/d (33·8 %) was not significantly different from the lymphocyte percentage in plasma after the placebo treatment period (P>0·1).
Analysis of efficacy variables
Conditional F tests showed overall WBE-related significant treatment effects for ten parameters (Table 4): level of bifidobacteria in faeces, percentage of bifidobacteria in faeces, level of Roseburia–E. rectale in faeces, faecal levels of acetic acid, propionic acid, butyric acid and total SCFA, stool pH, percentage stool moisture and urinary p-cresol levels (P < 0·1). The main results of the subsequent pairwise comparisons of these parameters will be discussed below.
Table 4 Efficacy variables during a human intervention study following intake of placebo, wheat bran extract (WBE) at 3 g/d or WBE at 10 g/d (Mean values and standard deviations)
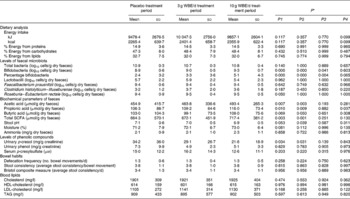
* P1 is the P value of the conditional F test for overall WBE-related significant treatment effects; P2, P3 and P4 are the P values for the comparison between WBE at 10 g/d and placebo, WBE at 10 g/d and WBE at 3 g/d, and WBE at 3 g/d and placebo, respectively.
Levels of faecal microbiota
In the PP population, WBE intake selectively increased bifidobacteria levels in the faeces (Table 4). Intake of WBE at 10 g/d increased the average levels of bifidobacteria in the faeces relative to placebo intake by 0·36 log units (P < 0·001). The average percentage of bifidobacteria relative to the total bacterial content in faeces upon intake of 10 g WBE/d increased by over 2-fold relative to placebo intake (P < 0·001), while intake of WBE at 3 g/d tended to increase the average percentage of bifidobacteria by 1·3-fold (P = 0·065). The faecal levels of Roseburia–E. rectale group remained unchanged after WBE intake, as were the faecal levels of total bacteria, lactobacilli, F. prausnitzii and the C. histolyticum–lituseburense group.
Biochemical parameters in faeces
Intake of WBE at 10 g/d increased the total level of faecal SCFA and the levels of acetic acid, propionic acid and butyric acid by about 8 % relative to placebo intake (P ≤ 0·05) (Table 4). Additionally, at the dose of 3 g/d, WBE significantly increased faecal propionic acid levels (P = 0·037). Consistently with the increased faecal SCFA levels, stool pH after intake of WBE at 10 g/d (pH = 6·93) was significantly lower as compared with the stool pH after placebo intake (pH = 7·10) (P = 0·039).
WBE intake did not affect the percentage moisture in faeces, nor did it influence faecal ammonia levels (P>0·1).
Urinary and serum levels of phenolic compounds
The completeness of each urine collection was estimated from the calculation of the observed:expected creatinine ratio as proposed by Knuiman et al. ( Reference Grabitske and Slavin 34 ). Of the 188 urine collections, thirty-one were classified as incomplete and were not taken into account for the statistical analysis of p-cresol and phenol excretion. Table 4 shows the effects of WBE and placebo intake on the urinary levels of phenolic compounds. In general, p-cresol levels were about four times higher than phenol levels. WBE intake at the dose of 10 g/d decreased urinary p-cresol levels by 37 % (P = 0·031). WBE intake did not influence urinary phenol excretion (P>0·1).
Average serum levels of p-cresylsulfate varied between 12·2 and 15·8 μm. WBE intake did not influence the serum p-cresylsulfate levels (P>0·1).
Energy intake
In the PP population, WBE intake did not modulate the average daily energy intake, or the average percentage of energy from carbohydrates, lipids or proteins (P ≥ 0·1).
Blood lipids
In the PP population, WBE intake did not affect the average blood levels of either total cholesterol, LDL-cholesterol, HDL-cholesterol or TAG (P>0·1).
Bowel habits: defecation frequency and stool consistency
WBE intake did not influence the number of bowel movements per d, nor did it modulate stool consistency as measured using the Bristol Stool Form Scale (P>0·1).
Analysis of tolerability
Tolerability was assessed through self-reported scoring by the volunteers of the occurrence frequency and distress severity of eighteen different gastrointestinal symptoms. During treatment with WBA at 10 g/d, both the occurrence frequency and distress severity of flatulence increased compared with the placebo treatment (P = 0·02, binary mixed model; P < 0·05, Bayesian ordinal mixed model) (Fig. 3(a) and (b)). With respect to occurrence frequency, the largest increase was seen in the scores ranging between occasionally and regularly, while no increases were seen in the more severe flatulence occurrence frequency scores. With respect to distress severity, the largest increase was seen in the scores ranging between minimally disturbing and mildly disturbing. A slight reduction in occurrence frequency of constipation was observed upon intake of WBE at 10 g/d compared with placebo intake (P = 0·095; binary mixed model) (Fig. 4). The subjects that never experienced constipation increased from 81 % during the placebo treatment period to 91 % during treatment with WBA at 10 g/d (P = 0·095; binary mixed model). None of the other assessed gastrointestinal symptoms was affected by WBE intake.
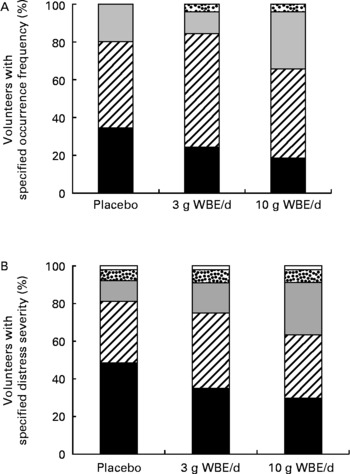
Fig. 3 (a) Distribution of flatulence occurrence frequency scores. (■), Frequency 0 (never); (
), frequency > 0–1; (
), frequency > 1–2; (
), frequency > 2–3. Frequency 1, occasionally; frequency 2, regularly; frequency 3, nearly always; WBE, wheat bran extract. (b) Distribution of flatulence distress severity scores. (■), Score 0 (not disturbing); (
), score > 0–1; (
), score > 1–2; (
), score > 2–3; (□), score > 3–4. Score 1, minimally disturbing; score 2, mildly disturbing; score 3, moderately disturbing; score 4, very disturbing.
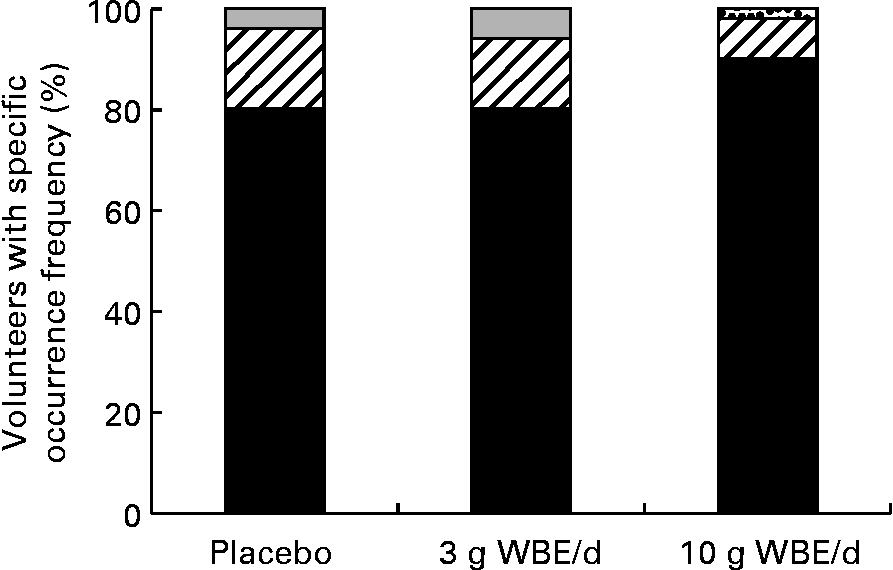
Fig. 4 Distribution of constipation occurrence frequency scores. (■), Frequency 0 (never); (
), frequency > 0–1; (
), frequency > 1–2; (
), frequency > 2–3. Frequency 1, occasionally; frequency 2, regularly; frequency 3, nearly always; WBE, wheat bran extract.
Discussion
WBE consumption during 3 weeks at a daily dosage of 10 g led to an increase in bifidobacteria counts relative to placebo intake. Cloetens et al. ( Reference Cloetens, Broekaert and Delaedt 10 ) previously showed that the stimulation of bifidobacteria after WBE intake was most pronounced in subjects with the lowest baseline levels. Since bifidobacteria levels have been shown to decrease upon ageing( Reference Hopkins, Sharp and Macfarlane 57 , Reference Woodmansey 58 ), we determined the effect of age on faecal bifidobacteria levels in two age subgroups: 18- to 50-year subgroup and ≥ 51-year subgroup. This analysis showed that intake of WBE at 10 g/d significantly (P < 0·05) increased faecal bifidobacteria in both age subgroups (data not shown). In addition, a statistically significant increase (P < 0·05) in bifidobacteria was found regardless of sex (data not shown).
It is well known that the prebiotic effect of a compound is strongly affected by the baseline levels of targeted beneficial bacteria in faeces, in the sense that relatively lower relative changes are observed upon intake of a prebiotic compound when the baseline levels are high( Reference Roberfroid 59 , Reference Roberfroid 60 ). Since the baseline level of faecal bifidobacteria in the present study was already high (log10 8·6 cells/g dry faeces), it is not surprising that the relative increase in faecal levels of bifidobacteria is relatively small. In addition, the change in absolute numbers of beneficial intestinal bacteria is considered to be more important for the prebiotic effect than the relative change( Reference Roberfroid 60 , Reference Meyer and Stasse-Wolthuis 61 ). The 0·4 log increase in faecal bifidobacteria levels after intake of WBE observed in the present study amounts to an increase by an absolute number of about 2 × 109 cells/g dry faeces, which is in the same order of magnitude observed in several other studies reviewed by Roberfroid et al. ( Reference Roberfroid 60 ).
We demonstrated that the total levels of faecal SCFA and of the individual SCFA, acetic acid, propionic acid and butyric acid, were increased upon intake of WBE at 10 g/d relative to placebo intake, while an increase of faecal propionic acid levels already occurred upon intake of WBE at 3 g/d. WBE appears to increase the faecal level of SCFA at lower doses than reported for other prebiotic compounds such as inulin and oligofructose. A number of studies in human volunteers who consumed oligofructose at high dosages varying from 13 to 20 g/d revealed an increase in faecal concentrations of acetic acid( Reference Van Dokkum, Wezendonk and Srikumar 62 ) or total SCFA, acetic acid and propionic acid( Reference Grasten, Liukkonen and Chrevatidis 63 ), but not in butyric acid( Reference Van Dokkum, Wezendonk and Srikumar 62 , Reference Grasten, Liukkonen and Chrevatidis 63 ). However, consumption of 20–40 g inulin/d was reported not to modulate faecal levels of SCFA in a statistically significant way( Reference Causey, Feirtag and Gallaher 64 – Reference Kleessen, Sykura and Zunft 66 ). Additionally, Gibson et al. could not demonstrate an increase in faecal SCFA levels after consumption of 15 g oligofructose/d( Reference Gibson, Beatty and Wang 67 ). The increased levels of bifidobacteria in the faeces could explain the increased faecal acetic acid levels after WBE intake as compared with placebo intake. In fact, in vitro studies have demonstrated that different Bifidobacterium sp. can degrade AXOS, thereby generating SCFA, mainly acetate and the organic acid lactate( Reference Yamada, Itoh and Morishita 6 , Reference Van Laere, Hartemink and Bosveld 68 ). Bifidobacteria do not produce butyric acid and a mechanism of cross-feeding with acetate- or lactate-converting bacteria may be involved in increased colonic butyric acid production( Reference Falony, Vlachou and Verbrugghe 69 , Reference Morrison, Mackay and Edwards 70 ). The levels of bacteria of the Roseburia–E. rectale group and of the F. prausnitzii group were determined given their butyric acid-producing capacity( Reference Louis and Flint 71 ). WBE intake did not increase faecal levels of the Roseburia–E. rectale group or of the Faecalibacterium group. However, the metabolic activity of the Roseburia–E. rectale group or the Faecalibacterium group could be changed upon WBE administration, leading to an increase in butyric acid-forming capacity, which cannot be detected using FISH. In addition, using a new molecular approach, based on analysis of the butytyl-CoA:acetate CoA transferase gene, Louis et al. identified twenty novel operational taxonomic units probably capable of producing butyrate( Reference Louis, Young and Holtrop 72 ). Hence, the observed increase in butyrate production upon WBE intake could be explained by an increase in the levels of novel, still to be identified, butyrate-producing strains. The ability of the different members of the gut community to compete successfully depends on many traits besides their ability to utilise certain substrates, such as their intrinsic growth rate, tolerance to colonic pH fluctuations and efficient binding to substrate particles and gut surfaces( Reference Louis, Young and Holtrop 72 ).
Concomitant with the increased SCFA production, intake of WBE at 10 g/d significantly decreased stool pH by about 0·2 units as compared with placebo intake. A more acidic gut environment is reported to enhance the colonisation resistance against pathogens( Reference Topping, Fukushima and Bird 73 ), to reduce the formation of secondary bile acids( Reference Zampa, Silvi and Fabiani 74 ) and to impair the activity of specific enzymes such as proteases( Reference Macfarlane, Allison and Gibson 75 ).
Intake of WBE at 10 g/d resulted in a marked reduction of urinary p-cresol levels by 37 % as compared with the p-cresol levels after placebo intake, which is indicative for a reduction in colonic protein fermentation. The reduction observed in the present study confirms previous results( Reference Cloetens, Broekaert and Delaedt 10 ) and is in agreement with foregoing studies in which a beneficial modulation of the colonic ammonia metabolism (another protein fermentation metabolite) was observed after AXOS intake( Reference Cloetens, De Preter and Swennen 36 ). Colonic fermentation of proteins results in the formation of ammonia, nitrosamines, thiols and phenolic compounds, which are generally believed to be potentially harmful. Hence, reduction of colonic protein fermentation is believed to be beneficial to human health( Reference De Preter, Falony and Windey 19 ).
Besides a small difference for lymphocyte percentage, we found no difference in any of forty-four safety-related blood parameters that were tested following WBE intake. The difference in lymphocytes was not considered toxicologically relevant since the observed difference was within the normal range and not dose-dependent, and since WBE intake did not affect the occurrence of adverse blood shifts in this or any other blood parameter. Absence of adverse effects on blood parameters was previously observed in a smaller-scale intervention trial on healthy subjects with WBE-like material( Reference Cloetens, Broekaert and Delaedt 10 ).
Intake of WBE at doses up to 10 g/d did not affect bowel habits. This is in agreement with a study in which intake of inulin at 20 g/d by healthy volunteers did not modulate stool frequency and consistency( Reference Slavin and Feirtag 65 ). In addition, intake of WBE at doses up to 10 g/d had no effect on most of the eighteen gastrointestinal symptoms surveyed. A mild increase of flatulence was observed at intake of WBE at 10 g/d. Mild to moderate flatulence was observed in some studies with other prebiotic compounds such as inulin and fructo-oligosaccharides, which is caused by the production of gases upon fermentation of the prebiotic compound( Reference Grabitske and Slavin 34 , Reference Slavin and Feirtag 65 , Reference Bouhnik, Vahedi and Achour 76 – Reference Bonnema, Kolberg and Thomas 79 ). On the other hand, a trend of decreased occurrence frequency of constipation was noted at the high WBE dose. Improvement of constipation symptoms has been observed for other prebiotic compounds, such as xylo-oligosaccharides( Reference Tateyama, Hashii and Johno 80 ) and inulin( Reference Kleessen, Sykura and Zunft 66 , Reference Den Hond, Geypens and Ghoos 81 , Reference Marteau, Jacobs and Cazaubiel 82 ), yet these studies were generally performed on constipated subjects.
The low incidence of gastrointestinal complaints and the absence of adverse changes in haematology and clinical blood chemistry parameters provide evidence for the excellent tolerability and safety of WBE, complementing the results of the in vitro and in vivo safety assessment of WBE( Reference François, Lescroart and Veraverbeke 83 ).
We recommend that WBE should be taken in at the dose of 10 g/d, considering that consumption of WBE at this dosage exerts the following beneficial effects on gut health parameters: increased production of SCFA, reduction of protein fermentation and increase of faecal bifidobacteria levels. Moreover, WBE is well tolerated and does not cause adverse effects at up to 10 g/d in healthy adult volunteers.
Acknowledgements
The authors thank Jan Wijffels (BNOSAC – the Belgium Network of Open Source Analytical Consultants) for the statistical analysis. The authors are also grateful to Erwin Raangs for technical assistance in analysing the faecal microbiota. The authors' responsibilities were as follows: I. E. J. A. F., O. L., J. A. D., C. M. C., K. V. and W. F. B. conceived of and designed the study; W. S. V. coordinated the production of the study's soft drinks; M. M. and S. P. were responsible for the analysis of ammonia content in stool samples; P. E. was responsible for the analysis of p-cresylsulfate content in serum; H. H., E. H. and K. W. were responsible for the analysis of the percentage moisture in the faecal samples and for the analysis of p-cresol content in urine; G. W. W. was responsible for the analysis of microbiota levels in the faecal samples; I. E. J. A. F. and W. F. B. were responsible for interaction with Jan Wijffels for analysis of the data; I. E. J. A. F. and W. F. B. had full access to all of the data in the study and jointly take responsibility for its integrity; I. E. J. A. F., O. L. and W. F. B. wrote the paper. I. E. J. A. F., O. L., W. S. V. and W. F. B. are presently employed by Fugeia NV, which manufactures the wheat bran extract product and is the sole sponsor source of funding for the studies described in the present paper.