Introduction
The intensification of land use to increase food production in the world has led to a reduction in its quality (Norris and Congreves, Reference Norris and Congreves2018), due to improper soil management, causing compaction, erosion, reduced fertility and contamination of the soil-water-plant environment (Bindraban et al., Reference Bindraban, Velde, Ye, Berg, Materechera, Kiba, Tamene, Ragnarsdóttir, Jongschaap, Hoogmoed, Hoogmoed, Beek and Lynden2012). In order to reverse this situation, combining the formation of dry matter for soil cover and food production, the intercropping between crops has been adopted. This system has been identified as a great alternative worldwide for intensifying food production in a sustainable way (Martin-Guay et al., Reference Martin-Guay, Paquette, Dupras and Rivest2018).
Among the various modalities, the intercropping between commercial grain-producing species with plants called green manures or cover crops has stood out as an advantageous production system. The species involved in the intercropping have roots with different morphology, increasing water absorption and avoiding losses through percolation and evaporation (Rahman et al., Reference Rahman, Liu, Hussain, Ahmed, Chen, Yang, Chen, Du, Liu and Yang2017). In addition, the roots of intercropped species grow so that the plants benefit each other by facilitating nutrient uptake (Li et al., Reference Li, Sum, Zhang, Guo, Bao, Smith and Smith2006). The species used as green manures are also able to fix atmospheric nitrogen and share part of this nutrient with the intercropped crop and in succession (Calegari and Carlos, Reference Calegari, Carlos, Lima Filho, Ambrosano, Rossi and Carlos2014).
Green manures promote improvements in the physical, chemical and biological attributes of the soil, increasing water infiltration rate and the formation of vegetation cover, as well as preventing erosion processes. Besides, they increase soil organic matter content, promote nutrient cycling and alter soil biota, providing conditions for the development of arbuscular mycorrhiza and microorganisms antagonistic to pathogens, promoting increases in grain yield (Amado et al., Reference Amado, Fiorin, Arns, Nicoloso, Ferreira, Lima Filho, Ambrosano, Rossi and Carlos2014; Calegari and Carlos, Reference Calegari, Carlos, Lima Filho, Ambrosano, Rossi and Carlos2014).
In Brazil, the intercropping of corn (Zea mays L.) with green manures (Urochloa ruziziensis and Crotalaria spectabilis) is used for grain production, as well as the formation of straw for the no-tillage systems (NTS). When used as a forage, it can also contribute to cattle breeding by supplying pasture, while when intercropped with the legume, aside from supplying N to the subsequent crop, it helps to reduce nematode populations (Arf et al., Reference Arf, Portugal, Buzetti, Rodrigues and Sá2018; Souza et al., Reference Souza, Couto Júnior, Flôres, Mingotte and Lemos2019).
In Brazil, rice (Oryza sativa L.) is also intercropped with essential crops for human consumption such as beans, cassava, corn and coffee, only as a subsistence crop, but with low frequency. Rice is also intercropped with forage, mainly Urochloa decumbens and U. brizantha, aiming to reduce costs, partially or totally, in the recovery/renewal of degraded pastures (Fornasieri Filho and Fornasieri, Reference Fornasieri Filho and Fornasieri2006). However, the inclusion of rice in this production system is extremely difficult, due to the lack of synchronism in growth between species, resulting in competition for water, light and nutrients, leading to losses in grain yield (Ramalho et al., Reference Ramalho, Utumi and Godinho2005; Saito et al., Reference Saito, Linquist, Keobualapha, Phanthaboon, Shiraiwa and Horie2006), which is the reason why in the world there is no technical recommendation to combine the cereal with green manures.
On the other hand, the dry biomass resulting from the intercropping of rice with green manures may increase the C/N ratio, promoting a longer period of soil cover compared to the sole cropping of green manures. In addition, the release of nutrients may be faster compared to the cultivation of rice as sole crop. This diversity of dry matter can cause the release of nutrients because its decomposition is synchronized with the demand required by the subsequent crop (Kaewpradit et al., Reference Kaewpradit, Toomsan, Cadisch, Vityakon, Limpinuntana, Saenjan, Jogloy and Patanothai2009), benefiting the grain yield of the crop in succession.
In Brazil, the succession of rice and common bean (Phaseolus vulgaris L.) makes it possible to bring benefits to the production system because they have morphologically different roots, are not susceptible to the same pests and diseases, and have different nutritional requirements. Rice is grown in the spring-summer season, in two large ecosystems, which are flooded and upland, either rainfed or with supplementary sprinkler irrigation (Fornasieri Filho and Fornasieri, Reference Fornasieri Filho and Fornasieri2006). Worldwide, rice cultivation ranks second in cultivated area, only after wheat (Triticum aestivum L.). Common bean has great flexibility in growing seasons, being the main grain-producing crop exploited in the autumn-winter season under irrigated conditions in the Central and Southeast regions of Brazil (Mingotte and Lemos, Reference Mingotte, Lemos, Paes and Andrade2018). Brazil is the country with the largest area cultivated with common bean crop in the world, with approximately 3.2 million ha (CONAB, 2020).
However, to make a production system feasible and sustainable, it is not enough to evaluate the increase in food production and the conservation of the environment. It is also necessary to assess the quality of the food produced. Rice is one of the main staple foods worldwide, with demand expected to increase by 8.5% by 2025 (OECD and FAO, 2019). This cereal provides 20% of the world's dietary energy, in addition to protein and vitamins. Common bean is an important source of protein, especially in developing countries, besides being a source of carbohydrates, fibres, vitamins and micronutrients (Castro-Guerrero et al., Reference Castro-Guerrero, Isidra-Arellano, Mendoza-Cozatl and Valdés-López2016).
The combination of rice and common bean in human food is essential for adequate nutrition and widely used by the Brazilian population. The common bean has a high protein value, being rich in lysine, but it contains a low content of sulphur amino acids. Rice, in turn, in its protein composition, is rich in sulphur amino acids and has a low lysine content. Thus, when ingesting the combination of rice and common bean, the biological value obtained is close to that of the protein of animal origin (Lajolo et al., Reference Lajolo, Genovese, Menezes, Araújo, Rava, Stone and Zimmermann1996), being an extremely important strategy in the context of food security.
The objective of this study was to verify the possibility of intercropping rice with green manure, as well as the impact of the dry biomass yield of these intercropping systems on common bean in succession, evaluating the agronomic and qualitative performance of grains from both crops.
Materials and methods
Experimental area location and characterization
The experiment was conducted in the 2018 and 2019 growing seasons using the succession of upland rice cultivated as a sole crop and intercropped with green manure in the spring/summer and common bean in the autumn/winter under NTS. The experiment was carried out in the city of Jaboticabal, São Paulo, Brazil, located at 21°14′33″S latitude and 48°17′10″W longitude, at an elevation of 565 m above sea level, under Aw climate type (humid tropical with rainy season in the summer and dry season in the winter), in a Latossolo Vermelho eutrófico (Oxisol), with clay texture (Santos et al., Reference Santos, Jacomine, Oliveira, Lumbreras, Coelho, Almeida, Cunha and Oliveira2013).
In the experimental area, millet (Pennisetum glaucum), cultivar ADR 300, was grown from December 2017 to March 2018. After its desiccation, the experimental area was under fallow until the implementation of rice cropping systems.
Prior to the installation of the experiment, soil collection was carried out in the 0.00–0.20 m layer for chemical and granulometric characterization, obtaining the following results: OM (organic matter) = 20 g dm−3; P(resin) = 65 mg dm−3; pH (CaCl2) = 5.6; K+ = 5.9 mmolc dm−3, Ca2+ = 39 mmolc dm−3, Mg2+ = 19 mmolc dm−3, H + Al = 29 mmolc dm−3, Al3+ = 0 mmolc dm−3 and CEC (cation exchange capacity) = 93 mmolc dm−3, respectively; base saturation = 69% (w/v); clay = 540 g kg−1; silt = 230 g kg−1 and sand = 230 g kg−1.
Experimental design and treatments
The experimental design was a randomized block with four replications. The treatments consisted of eight rice cropping systems, one with rice as sole crop and seven with rice intercropped with green manures, preceding the common bean crop. The cropping systems were: sole-crop rice, rice + Crotalaria breviflora; rice + C. spectabilis; rice + jack bean; rice + dwarf pigeon pea; rice + calopo; rice + forage peanut; rice + stylo. All green manures were sown simultaneously with rice. After rice harvest, the common bean was cultivated in the same location as the plots defined for rice.
Upland rice conduction
Each plot consisted of five 5 m-long rows of rice, with a spacing of 0.45 m between rows. The useful area was composed of three central rows, considering the lateral rows as a border. The green manures were sown between the rice rows, 0.225 m away from these.
Rice was sown on 13 November 2018, using 70 kg ha−1 of seeds of the cultivar BRS Esmeralda, which has long/fine grains, yield potential of 7525 kg ha−1, plant height ranging from 95 to 108 cm, cycle of about 77 days until flowering and from 105 to 110 days until maturation. This cultivar has initial vigour, with a fast canopy closure between rows, resistance to lodging, persistence of green colour of the leaves at the stage of grain maturation (stay green), as well as drought tolerance and moderate resistance to blast (Castro et al., Reference Castro, Morais, Breseghello, Lobo, Guimarães, Bassinello, Colombari Filho, Santiago, Furtini, Torga and Utumi2014).
The sowing density of green manures was the recommended amount according to Calegari and Carlos (Reference Calegari, Carlos, Lima Filho, Ambrosano, Rossi and Carlos2014) for C. breviflora D.C. (12 kg ha−1), C. spectabilis Roth (12 kg ha−1), jack bean – Canavalia ensiformis L. (80 kg ha−1), dwarf pigeon pea – Cajanus cajan L. (35 kg ha−1), calopo – Calopogonium mucunoides Desv. (8 kg ha−1), forage peanut – Arachis pintoi Krap. and Greg. (8 kg ha−1), stylo – Stylosanthes guianensis Aubl. Sw. (4 kg ha−1). Rice and green manure seeds were treated with pyraclostrobin (5 ml 100 kg seed−1) + thiophanate-methyl (45 ml 100 kg seed−1) + fipronil (50 ml 100 kg seed−1), aiming to control termites and Elasmopalpus lignosellus.
Fertilization at sowing of the rice crop was carried out with 210 kg ha−1 of the formulation 08-28-16, equivalent to 16.8 kg ha−1 of N, 58.8 kg ha−1 of P2O5 and 33.6 kg ha−1 of K2O, according to the recommendations of Cantarella and Raij (Reference Cantarella, Raij, Raij, Cantarella, Quaggio and Furlani1997). Top-dressing nitrogen (N) fertilization was performed at stage V3 (formation of the 3rd leaf collar on the main stem and beginning of tillering), at 22 DAE (days after emergence) at a dose of 60 kg ha−1 of N, using as source ammonium sulphate (Cantarella and Raij, Reference Cantarella, Raij, Raij, Cantarella, Quaggio and Furlani1997).
Phytosanitary management was performed according to the recommendation of Fornasieri Filho and Fornasieri (Reference Fornasieri Filho and Fornasieri2006). Rice was harvested on 19 March 2019. After rice harvest, the area was desiccated and, subsequently, the dry biomass was managed with a mechanical disintegrator.
Common bean conduction
The common bean crop was planted on 07 May 2019, spaced by 0.45 m between rows, with 12 seeds m−1, using the cultivar BRS Estilo. This cultivar belongs to the commercial group of ‘Carioca’ grains, with indeterminate growth habit, type II, of cycle between 85 and 95 days, has erect plant architecture and is resistant to lodging (Melo et al., Reference Melo, Peloso, Pereira, Faria, Costa, Díaz, Rava, Wendland, Carvalho, Costa, Almeida, Melo, Vieira Júnior, Faria, Souza, Marangon, Cargnin, Abreu, Moreira, Pereira Filho, Guimarães, Bassinello, Brondani and Magaldi2009). Each plot consisted of five 5 m-long rows of common bean, with a spacing of 0.45 m between rows. The useful area was composed of three central rows, considering the lateral rows as a border.
Fertilization at sowing was carried out with 200 kg ha−1 of the formulation 04-20-20, equivalent to 8 kg ha−1 of N, 40 kg ha−1 of P2O5 and 40 kg ha−1 of K2O, according to the recommendations of Ambrosano et al. (Reference Ambrosano, Wutke, Bulisani, Cantarella, Raij, Cantarella, Quaggio and Furlani1997). Top-dressing N fertilization was carried out at the stage V4−3 (3rd trifoliate leaf fully open) with 100 kg ha−1 of N using urea as a source (Ambrosano et al., Reference Ambrosano, Wutke, Bulisani, Cantarella, Raij, Cantarella, Quaggio and Furlani1997). Common beans were harvested on 21 August 2019. Phytosanitary management was performed according to the recommendation of Fornasieri Filho and Fornasieri (Reference Fornasieri Filho and Fornasieri2006) and Arf et al. (Reference Arf, Lemos, Soratto and Ferrari2015) for each crop.
Climatic characterization of the experimental period
Weather data of rainfall and maximum and minimum temperatures along the experiment are presented in Fig. 1. In order to meet the water requirements of the plants, a conventional sprinkler irrigation system was installed in the experimental area.

Fig. 1. Daily total rainfall and maximum and minimum temperature during the cultivation of rice as a sole crop and intercropped with green manures (a), and for common bean (b). Jaboticabal (SP), Brazil. 2018/19. S 0 = rice sowing, R 4 = rice flowering, R 9 = complete maturity of panicle, V 0 = common bean sowing, R 6 = common bean flowering, R 9 = physiological maturity of common bean.
Upland rice evaluation
In order to determine the agronomic performance of the rice crop, the number of panicles m−2 was evaluated by counting the panicles in 2.0 m of row of plants and subsequently calculating the number for m−2. The total number of spikelets per panicle was determined by the average number of grains from 20 panicles collected at the time of harvest. After separation of the grains by airflow, the numbers of filled and unfilled spikelets panicle−1 were determined. The mass of 100 grains was evaluated by weighing four samples of 100 grains in each plot (13% wet basis). The grains from the useful area of the plots were weighed, their moisture contents were corrected to 13% and the values were converted to kg ha−1, obtaining grain yield.
The quality of rice grains was determined based on the crude protein content, obtained by multiplying the N content in the grains (Sarruge and Haag, Reference Sarruge and Haag1974) by 6.25. The milling yield was obtained using a 100 g sample of rice paddy from each plot after processing in a mill, for 1 min. Afterwards, the polished grains were weighed, and the value found was considered as milling yield. After this process, burnished grains were placed in the ‘trieur’ n° 0 for 30 s, to separate the grains. The grains that remained in the ‘trieur’ were weighed to determine the head rice yield (whole milled grains) and the others were used to determine the broken-grain yield.
The residual dry biomass and consequently its decomposition, originating from cropping systems of rice with green manures, were evaluated by collecting the plant material with a 0.25 m2 frame (0.45 × 0.55 m) at two points in each plot. The material was dried in a forced-ventilation oven at an average temperature of 60–70°C until it reached equilibrium mass, and the values were converted into kg ha−1. The dry material was placed in nylon bags (‘litter bags’), with a 2 mm opening mesh, with dimensions of 0.25 × 0.25 m (0.06 m2) in a proportional amount to that obtained in kg ha−1. Three litter bags were placed per plot on 07 May 2019, just after sowing the common bean, and later the collection was made at 30, 60, 90 days after the installation of the litter bags, coinciding with the stages V3 (1st trifoliate leaf fully open), R 6 (end of flowering) and R 9 (beginning of pod maturation) of common bean, respectively. Each litter bag was dried in a forced-ventilation oven at an average temperature of 60–70°C until it reached an equilibrium mass, after which weighing was carried out.
Common bean evaluation
In the common bean crop, the agronomic performance was evaluated by the aerial dry biomass after the collection of ten plants in the useful area of the plot, during the full flowering of the plants (R 6), which were packed in paper bags, submitted to drying in a forced-ventilation oven at an average temperature of 60–70°C until reaching constant mass. After weighing, the values were converted into kg ha−1.
Common bean yield components were evaluated at harvest with the collection of ten consecutive plants, in the central row of the useful area of the plots. The number of pods plant−1 was evaluated as the relationship between the total number of pods and the number of plants. The average number of grains pod−1 was determined by the ratio of the total number of grains to the total number of pods. The mass of 100 grains was obtained by weighing four samples of 100 grains per plot. Grain yield was determined by manual harvesting of three rows; after drying in full sun, the grains were submitted to the mechanical threshing. The grains were weighed and the data were transformed into kg ha−1, corrected to 13% moisture. The percentage of Fusarium wilt (Fusarium oxysporum f. sp. phaseoli) incidence was obtained by the ratio between plants affected by the disease in the useful area of the plot and the total of plants.
The quality of the common bean grains was evaluated based on sieve yield ≥12, which was obtained by classifying the grains with the aid of sieves with oblong holes 12/64″ × 3/4 (4.76 × 19.05 mm) in agitation for 1 min. The percentage of grains retained was obtained by the relationship between the mass of grains on the sieve and the total mass of grains. The crude protein content was determined after obtaining the N content in the grains (Sarruge and Haag, Reference Sarruge and Haag1974) and multiplying it by 6.25.
Cooking time was determined with a Mattson cooker, after 16 h of hydration of the grains (Farinelli and Lemos, Reference Farinelli and Lemos2010). The level of resistance of the grains to cooking was verified according to the scale of Proctor and Watts (Reference Proctor and Watts1987). To evaluate the maximum hydration time, 50 g of common bean were weighed and 200 ml of deionized water were added. The volume was checked every 2 h with a test tube for 16 h. The data obtained were submitted to polynomial regression analysis, finding the value of the time needed for maximum hydration of the grains. The hydration ratio was determined by the difference between the mass of the grains after 16 h of hydration and the initial mass of the grains.
Statistical analyses
The data were submitted to analysis of variance (ANOVA) and, when significance was found for the F test (<0.05), the means were compared using the Scott–Knott test (0.05). The data presented in percentage were transformed to meet the criteria of the ANOVA. The analysis of the dry biomass decomposition of the intercropping plants was performed by the regression test according to the collection times, following the methodology of Wider and Lang (Reference Wider and Lang1982), adopting an equation with a higher coefficient of determination (R 2) and significant (P < 0.05).
Results
The intercropping of rice and green manures affected the agronomic performance of the cereal, with a statistical difference between the cropping systems for the variables number of panicles m−2, mass of 100 grains and grain yield (Table 1).
Table 1. Number of panicles m−2, number of total spikelets, filled and unfilled spikelets panicle−1, mass of 100 grains and grain yield of rice as sole crop or intercropped with green manures

Jaboticabal (SP), Brazil. 2018/19.
¹F test: **, * and ns – significant at 1% and 5% probability levels and not significant, respectively. Averages followed by the same letter in a column do not differ by Scott–Knott's test (P < 0.05). CV, coefficient of variation. 2Average: composed of treatments with values greater than zero.
The use of C. breviflora enabled the development and production of rice, but reduced the amount of panicles m−2, mass of 100 grains and grain yield in comparison to the cultivation of rice as sole crop and intercropped with forage peanut and stylo, indicating that there was also competition between these species (Table 1). The rice intercropped with C. breviflora reduced the grain yield of the cereal by 61% compared to the sole-crop cultivation.
The quality of rice grains was influenced by the intercropping systems with green manures, with statistical differences for all variables, except for the percentage of broken-grain yield (Table 2).
Table 2. Crude protein content, milling yield, head rice yield and broken-grain yield of rice as sole crop or intercropped with green fertilizers

Jaboticabal (SP), Brazil. 2018/19.
¹ F test: ** and ns – significant at 1% probability level and not significant, respectively. Averages followed by the same letter in a column do not differ by Scott–Knott's test (P < 0.05). CV, coefficient of variation.
2 Average: composed of treatments with values greater than zero.
The dry matter decomposition for all cropping systems, except for the rice intercropped with dwarf pigeon pea, followed the logarithmic mathematical model, with rapid decomposition in the first 30 days of common bean development. Rice intercropped with dwarf pigeon pea showed exponential decomposition, that is, gradual decomposition over time (Fig. 2).

Fig. 2. Residual dry biomass of rice as a sole crop and intercropped with green manures remaining in the soil during common bean cultivation. Jaboticabal (SP), Brazil. 2019. ¹F test: **significant at 1% probability level.
Prior to the cultivation of common beans, rice intercropped with stylo, calopo and forage peanut and sole-crop rice obtained dry biomass yields close to 6000 kg ha−1. For the intercropping of rice with jack bean, C. spectabilis and C. breviflora, the values were close to 8000 kg ha−1, while the intercropping with dwarf pigeon pea produced a dry biomass yield of around 11 000 kg ha−1.
The dry biomass yield of rice intercropped with C. breviflora favoured the dry biomass production of common bean plants over the other cropping systems, followed by rice intercropping with dwarf pigeon pea and C. spectabilis, despite making rice grain yield unfeasible (Table 3).
Table 3. Aerial dry biomass, number of pods plant−1, grains pod−1, mass of 100 grains, grain yield and incidence of Fusarium wilt in common bean in succession to rice as sole crop or intercropped with green manures
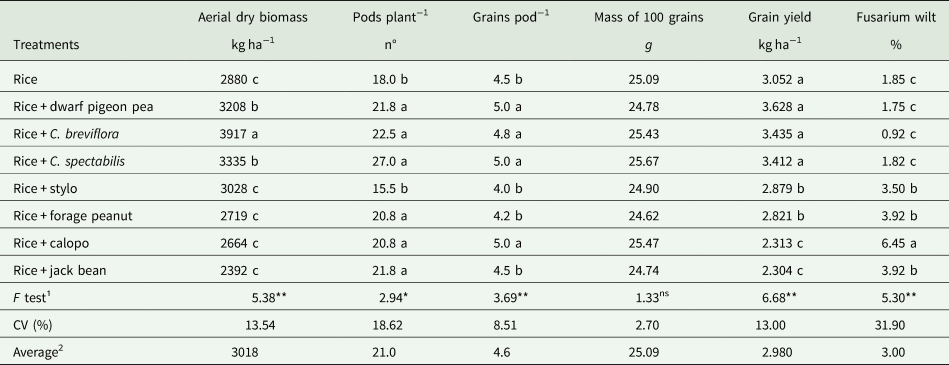
Jaboticabal (SP), Brazil. 2019.
¹F test: **, * and ns – significant at 1 and 5% probability levels and not significant, respectively. Averages followed by the same letter in a column do not differ by Scott–Knott's test (P < 0.05). CV, coefficient of variation. 2Average: composed of treatments with values greater than zero.
The number of pods per plant and the number of grains per pod of common bean showed statistical differences due to rice cropping systems (Table 3). The cultivation of rice as a sole crop and intercropped with stylo resulted in fewer pods per plant when compared to the other treatments. For the number of grains per pod, treatments of rice intercropped with dwarf pigeon pea, C. breviflora, C. spectabilis and calopo stood out. The mass of 100 grains of common bean was not influenced by rice cropping systems (Table 3).
The intercropping of rice with dwarf pigeon pea, C. breviflora and C. spectabilis provided greater dry biomass over the common bean cycle (Fig. 2), with positive effects on grain yield (Table 3). These cropping systems of intercropped rice, together with the sole-crop one, allowed grain yields higher than the average in the state of São Paulo, Brazil, for irrigated autumn-winter beans, 2713 kg ha−1 (IEA, 2020). These plants accumulate more N in the aerial part than stylo, forage peanut and calopo (Mendonça et al., Reference Mendonça, Lima, Guimarães, Moura and Andrade2017), making this nutrient available to the common bean cultivated in succession. N is the most exported nutrient by common bean, being a constituent of proteins and molecules such as chlorophyll, besides being related to the increase in photosynthetic rate, leaf growth, development of vegetative and reproductive organs and biomass production (Taiz et al., Reference Taiz, Zeiger, Møller and Murphy2017).
Despite the lower dry biomass yield, the cultivation of sole-crop rice also stood out in terms of grain yield of common bean in succession, due to the lower incidence of Fusarium wilt, when compared to the intercropping of rice with stylo, forage peanut and calopo, which showed higher incidence of the disease despite having dry biomass production and decomposition similar to those of sole-crop rice (Table 3). The higher residual biomass and decomposition of the rice intercropped with jack bean did not contribute to the grain yield of common bean, because of the incidence of Fusarium wilt disease in this succession of crops.
As for the quality of common bean, the percentage of grains retained on the sieve ≥12 (sieve yield), crude protein content, cooking time, time for maximum hydration and the hydration ratio of the grains were not affected by previous cropping systems (Tables 4 and 5). Sieve yield ≥12 of the common bean was close to 70% considered as ideal by Carbonell et al. (Reference Carbonell, Chiorato, Gonçalves, Perina and Carvalho2010) in all treatments. The crude protein content in common bean grains, in all rice cropping systems, was below that indicated for the cultivar BRS Estilo, which is 23% (Melo et al., Reference Melo, Peloso, Pereira, Faria, Costa, Díaz, Rava, Wendland, Carvalho, Costa, Almeida, Melo, Vieira Júnior, Faria, Souza, Marangon, Cargnin, Abreu, Moreira, Pereira Filho, Guimarães, Bassinello, Brondani and Magaldi2009), corroborating Vanier et al. (Reference Vanier, Ferreira, Lindemann, Santos, Bassinello and Elias2019). This may be due to the variation of this component according to the place of cultivation and climatic conditions (Lajolo et al., Reference Lajolo, Genovese, Menezes, Araújo, Rava, Stone and Zimmermann1996).
Table 4. Grains retained on the sieve ≥12, crude protein content, cooking time and level of resistance to cooking of common bean in succession to rice as sole crop or intercropped with green manures

Jaboticabal (SP), Brazil. 2019.
¹ F test: ns – significant at 1 and 5% probability levels and not significant, respectively. Averages followed by the same letter in a column do not differ by Scott–Knott's test (P ≤ 0.05). CV, coefficient of variation.
2 Average: composed of treatments with values greater than zero.
Table 5. Regression equation between the time (T – h) for grain hydration and the amount of water absorbed (WA – ml) by common bean, determination coefficient (R 2) and time for maximum grain hydration (TMH) and the hydration ratio (HR) of the common bean in succession to rice as sole crop or intercropped with green manures

Jaboticabal (SP), Brazil. 2019.
¹ F test: ** and ns – significant at 1% probability level and not significant, respectively. CV, coefficient of variation.
2 x = time for hydration (min) and y = amount of water absorbed (ml).
The average value of the cooking time was 21 min and 50 s, considered adequate for being below 30 min (Ramalho and Abreu, Reference Ramalho, Abreu, Vieira, Paula Junior and Borém2006). In this context, for the cultivar BRS Estilo, used in this work, Melo et al. (Reference Melo, Peloso, Pereira, Faria, Costa, Díaz, Rava, Wendland, Carvalho, Costa, Almeida, Melo, Vieira Júnior, Faria, Souza, Marangon, Cargnin, Abreu, Moreira, Pereira Filho, Guimarães, Bassinello, Brondani and Magaldi2009) obtained a cooking time of 26 min. In addition, the values obtained were classified as normal cooking resistance, according to Proctor and Watts (Reference Proctor and Watts1987), which promotes energy savings and practicality in preparing meals (Perina et al., Reference Perina, Carvalho, Chiorato, Lopes, Gonçalves and Carbonell2014).
From the regression equations between the hydration time and the amount of water absorbed by the grains (Table 5), the time necessary for maximum hydration of the grains was obtained. The average value was 11 h and 24 min, which is a favourable result, since common beans are usually soaked for 12 h before cooking in Brazilian cuisine (Souza et al., Reference Souza, Couto Júnior, Flôres, Mingotte and Lemos2019). In all treatments, the common bean grains showed a hydration ratio >2, indicating that the water absorption was equivalent to their initial weight, that is, there was an adequate performance of the grains in relation to this characteristic (Farinelli and Lemos, Reference Farinelli and Lemos2010).
Discussion
It is noteworthy that the yield of rice when intercropped with forage peanut and stylo was statistically similar to that obtained with its sole cropping (Table 1). This was possible due to the slow development and reduced size of forage peanut and stylo, resulting in low competition for water, light and nutrients. Shampazuraini et al. (Reference Shampazuraini, Husein, Sitiaminah and Nurhasanah2016) found that forage peanuts intercropped with rice did not affect grain yield, but increased the aerial dry biomass compared to the cultivation of rice as sole crop.
All cropping systems (sole-crop rice and intercropping systems) showed grain yield above the world average (4100 kg ha−1) and close to the Brazilian average, which is 6020 kg ha−1 (IRRI, 2020). These results are also due to the adequate crop management, with emphasis on the choice of the cultivar BRS Esmeralda, which has great stability and adaptability in several regions of Brazil (Castro et al., Reference Castro, Morais, Breseghello, Lobo, Guimarães, Bassinello, Colombari Filho, Santiago, Furtini, Torga and Utumi2014). Regarding the weather conditions that occurred during rice cultivation (Fig. 1a), three drought periods were observed: 03–12 December 2018, 15–24 January 2019, and 28 January 2019 to 05 February 2019. According to Guimarães et al. (Reference Guimarães, Stone and Silva2016), 10 days of drought can cause 31 and 51% reductions in rice grain yield when it occurs at the stages of panicle appearance (R 3) and grain length expansion (R 5), respectively. The water supply was carried out in the short-term drought periods by supplemental irrigation, and the water depth supplied was 90 mm, added to 830 mm from the rain in the rest of the rice cycle. It is also noteworthy that the cultivar BRS Esmeralda has drought tolerance (Castro et al., Reference Castro, Morais, Breseghello, Lobo, Guimarães, Bassinello, Colombari Filho, Santiago, Furtini, Torga and Utumi2014), and the physical and chemical attributes of the soil such as clayey texture, having greater capacity to store water, low acidity, as well as high levels of phosphorus, potassium, calcium and magnesium, contributed to root development and plant nutrition, positively affecting grain yield.
The intercropping of rice with dwarf pigeon pea, C. spectabilis, calopo and jack bean, made the development and grain yield of rice unfeasible due to the rapid growth and competitiveness of these green manures in comparison to the cereal. These plants suppressed the rice plants, due to shading, reducing the development of cells in young and old leaves, damaging the leaf area (Wu et al., Reference Wu, Gong and Yang2017), besides decreasing the absorption and utilization of nutrients (Taiz et al., Reference Taiz, Zeiger, Møller and Murphy2017). Wang et al. (Reference Wang, Deng, Ren and Yang2013) observed that shading, especially at more advanced phenological stages, change the composition of rice starch, increasing the amount of broken and poorly developed grains. Chen et al. (Reference Chen, Li, Zeng, Deng and Ren2019) observed that shading reduces spikelet filling and rice grain mass, directly interfering with crop yield. The authors found that shading interferes with the availability and quality of light for rice, a factor that affects the content and ratio of chlorophylls a and b and, consequently, reduces photosynthetic efficiency and increases heat energy dissipation. Pan et al. (Reference Pan, Liu, Mo, Patterson, Duan, Tian, Hu and Tang2016) also found that shading reduced photosynthetic rate, total root length, grain mass and, consequently, rice yield.
Another aspect that may have contributed is that rice plants have slow initial development until 30–40 days after emergence (Ramalho et al., Reference Ramalho, Utumi and Godinho2005), in addition to being a species with C3 metabolism, having lower efficiency of energy conversion into biomass compared to plants with C4 metabolism (Taiz et al., Reference Taiz, Zeiger, Møller and Murphy2017). The intercropping of rice with C. breviflora promoted a higher protein content in the grains, probably due to the concentration effect (Jarrel and Beverly, Reference Jarrel and Beverly1981). However, this intercropping system hampered the quality of the grains, reducing the percentage of milling yield and head rice yield, when compared to rice as sole crop or intercropped with stylo and forage peanut (Table 2). It should be noted that the protein values found in rice cropping systems are within the recommended range for the cereal, which can vary from 5.1 to 11.3% (Champagne et al., Reference Champagne, Bett-Garber, Vinyard, McClung, Barton, Moldenhauer, Linscombe and McKenzie1999). Crusciol et al. (Reference Crusciol, Arf, Soratto and Mateus2008) found protein values in rice grains ranging from 7.5 to 8.4%.
In Brazil, the recommended milling yield is above 68% (Fornasieri Filho and Fornasieri, Reference Fornasieri Filho and Fornasieri2006), and only the intercropping of rice with C. breviflora led to milling yield below this value. The values of milling yield and head rice yield were similar to those found by Portugal et al. (Reference Portugal, Arf, Buzetti, Portugal, Garcia, Meirelles, Garé, Abrantes and Rodrigues2020) in rice cultivation under NTS, for the cultivar BRS Esmeralda. Rice quality is also assessed by the percentage of broken-grain yield and rice grits. The classification varies in category 1–5, in which 1 tolerates up to 7.5% of broken-grain and category 5, up to 45% of broken-grain yield and rice grits (Brazil, 2010). In the present study, in all rice cropping systems, the percentage was <3%, indicating that they fall into type 1. This characteristic gives greater value to the product in commercialization, since the consumer has a preference for rice with uniform and head rice grains.
We highlight that the dry biomass yield obtained in the intercropping is important for the viability of the NTS, and consequently for conducting a conservational and sustainable agriculture, since it enables the covering of the soil, contributing to the reduction of erosive processes, through the lower impact of raindrops on the soil, allowing greater water infiltration in the soil and less runoff. In order to have adequate soil cover in this production system, it is recommended that 7000–8000 kg ha−1 of dry biomass be produced in tropical and subtropical regions in the Brazilian Cerrado (Aidar et al., Reference Aidar, Kluthcouski, Cobucci and Paula Júnior2007). Thus, the intercropping systems of rice with jack bean, C. spectabilis, C. breviflora and dwarf pigeon pea showed dry biomass yield values above 8000 kg ha−1, proving to be advantageous for the quality of NTS.
Another important aspect refers to the results of dry biomass yield being similar to that found by Arf et al. (Reference Arf, Portugal, Buzetti, Rodrigues and Sá2018) in an intercropping of corn with green manures (jack bean, dwarf pigeon pea and C. spectabilis), noting that the amount of N present in the dry biomass of the intercropped cultivation was 48% higher than that of sole-crop corn. Common bean crop shows higher macronutrient absorption in the leaves between 28 and 31 DAE (Pegoraro et al., Reference Pegoraro, Oliveira, Moreira, Kondo, Maia and Vieira2013). Thus, the greater initial decomposition of the previous crop may have favoured the development of common bean in the first 30 days, which was at stage V 3, with this decomposition being higher in the cropping systems of rice intercropped with C. breviflora, jack bean, dwarf pigeon pea and C. spectabilis (2328, 2276, 2055 and 1977 kg ha−1, respectively).
In intercropped systems, as in the present work, where different species are cultivated in the same period, there are improvements in the diversification of microbial species in the soil, favouring microorganisms that have the function of biocontrol against certain pathogens (Vukicevich et al., Reference Vukicevich, Lowery, Bowen, Úrbez-Torres and Har2016). However, some plant exudates may also favour an increase in the population of pathogenic microorganisms (Nicol et al., Reference Nicol, Yousef, Traquair and Bernards2003). Plants grown before common bean can be hosts of the pathogen, such as jack beans, and other bad hosts such as rice (Dhingra and Coelho-Neto, Reference Dhingra and Coelho-Neto2001).
In this sense, we verified that the cultivation of stylo, forage peanut, calopo and jack bean with rice increases Fusarium wilt incidence in common bean cultivated in succession. According to Calegari and Carlos (Reference Calegari, Carlos, Lima Filho, Ambrosano, Rossi and Carlos2014), some species of green manures can multiply diseases and pests that attack crops of economic interest, such as common bean. Thus, green manure species that multiply pathogens should be avoided when economic interest crops are also susceptible to them. Gridi-Papp et al. (Reference Gridi-Papp, Fuzatto, Ferraz and Cia1970) observed that green manures can multiply Fusarium wilt and increase the inoculum source and disease incidence in crops grown in succession.
Common bean grain yield ranged from 2304 to 3628 kg ha−1, obtained after the cultivation of rice intercropped with jack bean and dwarf pigeon pea, respectively (Table 3). Average grain yield was 2980 kg ha−1, which is higher than the Brazilian average for autumn-winter cultivation, which is 1348 kg ha−1 (CONAB, 2020). In addition to the influence of rice cropping systems, as sole crop and intercropped with green manures, the common bean grain yield may be linked to cultural management, with emphasis on water supply, totalling 423 mm of total water depth, being 48 mm from rain and the rest supplied by irrigation. Regarding the climatic conditions, it was found that in the period from 06 to 07 November 2019, there were minimum temperatures below 10°C (Fig. 1b), which paralyze plant development (Wutke et al., Reference Wutke, Brunini, Barbano, Castro, Gallo, Kanthack, Martins, Pereira, Bortoleto, Paulo, Sakai, Saes, Ambrosano, Carbonell and Silveira2000). However, this cold period was short so that it did not influence the cycle and the yield performance of the common bean.
We found that the cultivation of rice (sole crop) and common bean was the crop succession that promoted the largest total amount of grains, 9927 kg ha−1, with 6875 kg ha−1 coming from rice and 3052 kg ha−1 from beans (Fig. 3). The cropping systems of rice intercropped with stylo and forage peanut also stood out for the total grain yield, which was 9010 kg ha−1 (6131 kg ha−1 of rice grains and 2879 of common bean) and 8911 kg ha−1 (6091 kg ha−1 of rice grains and 2821 kg ha−1 of common bean), respectively. Grain yield close to 10 000 kg ha−1 year−1 has been observed in the succession of grain crops under NTS, such as for soybean/corn (Nóia Júnior and Sentelhas, Reference Nóia Júnior and Sentelhas2019) and corn/common bean (Souza et al., Reference Souza, Couto Júnior, Flôres, Mingotte and Lemos2019).

Fig. 3. Total grain yield of rice (as sole crop and intercropped with green manures) and common bean. Jaboticabal (SP), Brazil. 2018/19. F test = 128.32** – significant at 1% probability level. Means followed by the same letter on the bars do not differ by the Scott–Knott (P ≤ 0.05). Coefficient of variation = 9.92%.
Conclusion
The results from this study show that intercropping of upland rice is viable depending on the green manure species. The intercropping of rice with stylo and forage peanut made it possible to obtain grain yield and quality similar to those obtained with the cultivation of sole-crop rice. The cropping systems of rice intercropped with dwarf pigeon pea, C. breviflora and C. spectabilis, although unfeasible in terms of cereal grain yield, provided greater dry biomass yield that can help long-term soil conservation and increase the system's yield.
Financial support
Coordenação de Aperfeiçoamento de Pessoal de Nivel Superior (CAPES), finance code 001.
Conflict of interest
None.
Ethical standards
Not applicable.