1. Introduction
Dementia is a leading cause of disability in older adults, with Alzheimer's disease (AD) accounting for roughly two-thirds of cases (World Health Organization, 2021). Development of AD typically involves neural atrophy in the medial temporal lobe (MTL), and follows a continuum of clinical severity with subjective cognitive decline (SCD) predating mild cognitive impairment (MCI), which predates AD (Scheltens et al., Reference Scheltens, De Strooper, Kivipelto, Holstege, Chételat, Teunissen, Cummings and van der Flier2021). However, some individuals appear better able than others to maintain cognitive functioning despite evident neuropathology (e.g., Snowdon, Reference Snowdon2003). The concepts of reserve and resilience are proposed to account for this often-observed discrepancy between amount of brain pathology and one's cognitive function. Certain lifestyle factors and experiences are hypothesized to contribute to structural and functional brain changes that underlie resilience in aging (Steffener & Stern, Reference Steffener and Stern2012; Stern, Reference Stern2002; Stern et al., Reference Stern, Arenaza-Urquijo, Bartrés-Faz, Belleville, Cantilon, Chetelat, Ewers, Franzmeier, Kempermann and Kremen2020, Reference Stern, Albert, Barnes, Cabeza, Pascual-Leone and Rapp2023). Bilingualism has been identified as one such factor that may contribute to greater resilience (e.g., Bialystok et al., Reference Bialystok, Craik and Freedman2007). The aim of this study was to use structural neuroimaging methods to explore bilingualism and resilience along the continuum of AD development.
Resilience is defined as a general term that encompasses any concept that relates to the brain's ability to maintain cognitive function in the context of aging and disease (Stern et al., Reference Stern, Albert, Barnes, Cabeza, Pascual-Leone and Rapp2023). Three mechanisms of resilience are commonly referred to in the literature: brain reserve, cognitive reserve and brain maintenance. Brain reserve is believed to be accrued across the lifespan through a variety of enriching and stimulating experiences and reflects the amount of neurobiological substrate available at a given time. With the assumption that cognitive deficits occur after a fixed threshold of brain pathology, an individual with greater brain reserve would be able to sustain a larger amount of pathology before reaching that threshold (Stern, Reference Stern2002; Stern et al., Reference Stern, Arenaza-Urquijo, Bartrés-Faz, Belleville, Cantilon, Chetelat, Ewers, Franzmeier, Kempermann and Kremen2020, Reference Stern, Albert, Barnes, Cabeza, Pascual-Leone and Rapp2023). Cognitive reserve, on the contrary, is believed to come into play when there is a need for it (i.e., in an attempt to overcome or compensate for a neurological insult or age-related neurodegeneration) and relates to functional brain changes that result in greater neural adaptability and flexibility in using existing neural resources. This is proposed to lead to outcomes such as increased neural efficiency or the use of alternate neural resources (i.e., neural compensation) to perform cognitive tasks in the context of brain aging or pathology (Stern et al., Reference Stern, Arenaza-Urquijo, Bartrés-Faz, Belleville, Cantilon, Chetelat, Ewers, Franzmeier, Kempermann and Kremen2020, Reference Stern, Albert, Barnes, Cabeza, Pascual-Leone and Rapp2023). Individuals with greater cognitive reserve are expected to have more brain atrophy compared to individuals who perform at the same cognitive level but have lower cognitive reserve (e.g., Stern, Reference Stern2002). Lastly, brain maintenance refers to the maintenance of brain structure, or a reduced development of age-related brain changes and brain pathology, over time due to genetics or lifestyle factors. Greater brain maintenance may then be considered a mechanism of sustaining greater brain reserve over time (Stern et al., Reference Stern, Arenaza-Urquijo, Bartrés-Faz, Belleville, Cantilon, Chetelat, Ewers, Franzmeier, Kempermann and Kremen2020, Reference Stern, Albert, Barnes, Cabeza, Pascual-Leone and Rapp2023). Thus, the concepts of resilience and reserve involve structural and/or functional brain changes across the lifespan and their relation to cognitive functioning.
Certain lifestyle factors and experiences are thought to contribute to the development of greater resilience. Bilinguals have been reported to show a 4–5 year delay in AD symptom onset compared to monolinguals (e.g., Alladi et al., Reference Alladi, Bak, Duggirala, Surampudi, Shailaja, Shukla, Chaudhuri and Kaul2013; Anderson et al., Reference Anderson, Hawrylewicz and Grundy2020; Bialystok et al., Reference Bialystok, Craik and Freedman2007), suggesting that bilingualism may be one such factor that protects against cognitive decline in dementia. Findings from structural neuroimaging studies have also demonstrated an association between bilingualism and resilience, suggesting greater brain and cognitive reserve for bilinguals compared to monolinguals.
For example, bilingualism has been associated with greater brain reserve in language-related brain regions. However, much of this evidence comes from studies of healthy younger and older adults. For example, greater gray matter has been observed in healthy younger and older bilinguals versus monolinguals in anterior cingulate cortex (ACC; e.g., Abutalebi et al., Reference Abutalebi, Della Rosa, Green, Hernandez, Scifo, Keim, Cappa and Costa2011, Reference Abutalebi, Guidi, Borsa, Canini, Della Rosa, Parris and Weekes2015b) and inferior parietal lobe (IPL; e.g., Abutalebi et al., Reference Abutalebi, Canini, Della Rosa, Green and Weekes2015a; Mechelli et al., Reference Mechelli, Crinion, Noppeney, O'Doherty, Ashburner, Frackowiak and Price2004). Similarly, bilingualism has been associated with greater cortical thickness in inferior frontal gyrus (IFG; e.g., Klein et al., Reference Klein, Mok, Chen and Watkins2014), expansions in the basal ganglia and thalamus (e.g., Burgaleta et al., Reference Burgaleta, Sanjuán, Ventura-Campos, Sebastian-Galles and Ávila2016) and greater gray matter volume (GMV) in the cerebellum (e.g., Pliatsikas et al., Reference Pliatsikas, Johnstone and Marinis2014) for healthy young adults, and greater hippocampal volume in healthy older adults (e.g., Voits et al., Reference Voits, Robson, Rothman and Pliatsikas2022). In addition, our group has previously reported greater cortical thickness in language control areas (e.g., IFG, ventromedial prefrontal cortex, cerebellum), and greater hippocampal volume, for multilinguals versus monolinguals with MCI and AD (Duncan et al., Reference Duncan, Nikelski, Pilon, Steffener, Chertkow and Phillips2018). Notably, there is little research examining bilingualism and brain structure longitudinally in the context of AD. However, Costumero et al. (Reference Costumero, Marin-Marin, Calabria, Belloch, Escudero, Baquero, Hernandez, Ruiz de Miras, Costa and Parcet2020) reported a slower rate of decline in parenchymal volume over a 7 month period for bilingual compared to monolingual participants with MCI. Thus, evidence suggests that bilingualism may be associated with greater brain reserve and, perhaps, the maintenance of brain structure over time (i.e., brain maintenance).
Bilingualism has also been associated with greater cognitive reserve in the context of MCI and AD. Schweizer et al. (Reference Schweizer, Ware, Fischer, Craik and Bialystok2012) observed greater atrophy in AD-related areas for bilinguals compared to monolinguals with AD, despite no statistically significant difference in age, education or cognitive function. Similarly, Duncan et al. (Reference Duncan, Nikelski, Pilon, Steffener, Chertkow and Phillips2018) observed less gray matter density in parahippocampal gyri for multilinguals versus monolinguals with AD, despite being matched on age, education, episodic memory and cognitive function. Additionally, Costumero et al. (Reference Costumero, Marin-Marin, Calabria, Belloch, Escudero, Baquero, Hernandez, Ruiz de Miras, Costa and Parcet2020) observed reduced brain parenchyma volume for bilingual compared to monolingual participants with MCI, despite no statistically significant difference in age, education or cognitive level. Thus, bilinguals may better maintain cognitive functioning, even when they present with greater neuropathology.
Despite these findings, there is still debate regarding the benefits of bilingualism on cognition and aging (e.g., Bak & Alladi, Reference Bak and Alladi2016; Del Maschio et al., Reference Del Maschio, Fedeli and Abutalebi2021; Fuller-Thomson, Reference Fuller-Thomson2015; Lawton et al., Reference Lawton, Gasquoine and Weimer2015; Mukadam et al., Reference Mukadam, Sommerlad and Livingston2017). However, few studies have examined bilingualism and reserve in older adults with MCI or AD, and none in older adults with SCD (i.e., self-reported cognitive complaints but no objective cognitive impairments). Therefore, we examined structural brain differences in language-related and AD-related areas in bilinguals and monolinguals at varying stages of AD development (i.e., from healthy older adults to older adults with SCD, MCI, and AD). Given the literature presented above (e.g., Duncan et al., Reference Duncan, Nikelski, Pilon, Steffener, Chertkow and Phillips2018), we predicted greater brain reserve (i.e., greater cortical thickness and volume) in language-related areas and greater cognitive reserve (i.e., greater atrophy in AD-related brain regions) for bilinguals compared to monolinguals.
2. Methods
2.1 Participants
We used data from The Comprehensive Assessment of Neurodegeneration and Dementia Study (COMPASS-ND; N = 356) of the Canadian Consortium on Neurodegeneration in Aging (CCNA) and the Consortium for the Early Identification of Alzheimer's disease-Quebec (CIMA-Q; N = 175). The COMPASS-ND study is CCNA's signature clinical study of Canadians with, or at risk for, various types of dementia. The COMPASS-ND study aims to learn about neurodegenerative diseases in aging, with a focus on early detection and diagnosis, prevention and improving quality of life (Chertkow et al., Reference Chertkow, Borrie, Whitehead, Black, Feldman, Gauthier, Hogan, Masellis, McGilton and Rockwood2019). CIMA-Q is a longitudinal study of more than 350 older adults that aims to facilitate earlier AD diagnosis, make a well-characterized observational cohort available to the scientific community, and identify and support the study of new therapeutic targets to slow or prevent cognitive decline and AD (Belleville et al., Reference Belleville, LeBlanc, Kergoat, Calon, Gaudreau, Hébert, Hudon, Leclerc, Mechawar and Duchesne2019).
Our sample was restricted to right-handed participants who were cognitively unimpaired (CU), had SCD, MCI or mild AD (as defined in Belleville et al., Reference Belleville, LeBlanc, Kergoat, Calon, Gaudreau, Hébert, Hudon, Leclerc, Mechawar and Duchesne2019; Chertkow et al., Reference Chertkow, Borrie, Whitehead, Black, Feldman, Gauthier, Hogan, Masellis, McGilton and Rockwood2019), and had complete data for our variables of interest. Sixty participants were excluded due to outlier values greater than 3 standard deviations from the group mean on our dependent variables. An additional 10 participants were excluded due to magnetic resonance imaging (MRI) data preprocessing errors. Within diagnosis groups, language groups were strictly matched on participant age, biological sex, years of education, memory performance (i.e., delayed-recall score on the Logical Memory subtest of the Wechsler Memory Scale-III) and cognitive function (i.e., total score on the Montreal Cognitive Assessment) using 1:1 matching by the ‘MatchIt’ package in R (Ho et al., Reference Ho, Imai, King and Stuart2011). Exact matching was used for biological sex and optimal matching was used for all other matching variables. This resulted in a final sample of 364 participants (109 from CIMA-Q and 255 from COMPASS-ND). See Table 1 for participants’ demographic information.
Table 1. Participant demographics

Note: Standard deviations are reported in parentheses.
CU, cognitively unimpaired; SCD, subjective cognitive decline; MCI, mild cognitive impairment; AD, Alzheimer's disease; MoCA, Montreal Cognitive Assessment.
The data for this study are stored on the Longitudinal Online Research Information System (Das et al., Reference Das, Zijdenbos, Harlap, Vins and Evans2012; Mohaddes et al., Reference Mohaddes, Das, Abou-Haidar, Safi-Harab, Blader, Callegaro, Henri-Bellemare, Tunteng, Evans and Campbell2018). This study was approved by all relevant ethics committees and all participants provided written informed consent.
2.2 Language groups
Participants self-reported their native language (L1) and number of languages, which was used to define our language groups (monolingual = one language; bilingual = two or more languages). Monolinguals reported English (71%) or French as their L1. Bilinguals reported English (37.9%), French (39.0%) or a variety of other languages as their L1. Within the bilingual group, 67.6% reported knowing two languages, 22.0% reported knowing three languages and the remaining participants reported knowing between four and seven languages. Notably, 11% of monolinguals and 32% of bilinguals were immigrants. Additional language experience data were available for a subset of the COMPASS-ND bilingual participants (n = 119), including age of acquisition (AoA), self-reported language ability and amount of language use (see Table 2). The majority of our subsample reported English or French as their L1 (English: 50.4%; French: 18.5%) or second language (L2) (English: 37.8%; French: 41.2%), with the remaining participants reporting a variety of other languages. They reported a range of L2 AoA and L2 ability. However, our subsample may be characterized as mostly late bilinguals (i.e., learning an L2 after age 5), having moderate self-reported L2 ability, and relatively few participants reporting daily L2 use (33 out of 119).
Table 2. Additional language information for a subsample of bilingual participants
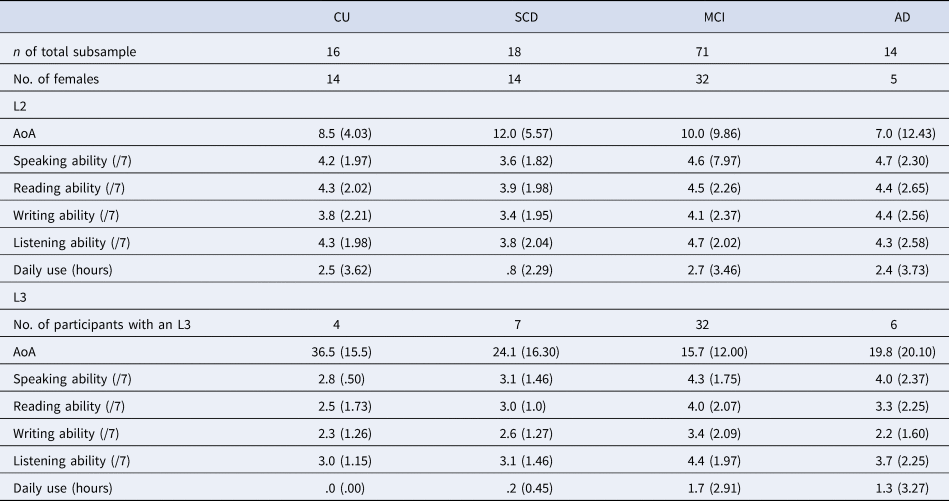
Note: We do not report additional language data for participants’ native language (L1), given that additional L1 language data were not provided by all participants in the subsample. In addition, we do not report additional language data for participants' languages past their third language, given that too few participants in the subsample reported knowing more than three languages. Participants self-reported their language ability in speaking, reading, writing and listening on a scale from 1 (very poor) to 7 (native-like). Daily use reflects participants' self-reported number of hours spent speaking the given language per day. Standard deviations are reported in parentheses.
CU, cognitively unimpaired; SCD, subjective cognitive decline; MCI, mild cognitive impairment; AD, Alzheimer's disease; AoA, age of acquisition; L2, second language; L3, third language.
2.3 MRI data acquisition and preprocessing
T1-weighted images were obtained using 3T scanners following the Canadian Dementia Imaging Protocol (Duchesne et al., Reference Duchesne, Chouinard, Potvin, Fonov, Khademi, Bartha, Bellec, Collins, Descoteaux and Hoge2019; www.cdip-pcid.ca). Cortical reconstruction and volumetric segmentation were performed using FreeSurfer (version 6.0; http://surfer.nmr.mgh.harvard.edu). The FreeSurfer pipeline performs surface-based morphometry, which involves multiple processing steps that have been described in detail in previous publications (e.g., Dale et al., Reference Dale, Fischl and Sereno1999; Fischl & Dale, Reference Fischl and Dale2000). The fully automated pipeline was run on CBRAIN (Sherif et al., Reference Sherif, Rioux, Rousseau, Kassis, Beck, Adalat, Das, Glatard and Evans2014). All brain scans were manually checked for segmentation precision. Automatic segmentation of subcortical structures and hippocampal subregions was performed using the FreeSurfer subcortical and hippocampal atlases (Fischl et al., Reference Fischl, Salat, Busa, Albert, Dieterich, Haselgrove, Van Der Kouwe, Killiany, Kennedy and Klaveness2002; Iglesias et al., Reference Iglesias, Augustinack, Nguyen, Player, Player, Wright, Roy, Frosch, McKee and Wald2015). Cortical parcellation was performed using the Desikan–Killiany–Tourville atlas (Klein & Tourville, Reference Klein and Tourville2012). As mentioned, 10 participants were excluded due to errors in the automatic segmentation of gray and white matter.
2.4 Regions of interest
Language-related regions of interest (ROIs) were regions commonly identified as being involved in bilingual language control (e.g., Abutalebi & Green, Reference Abutalebi and Green2016; Calabria et al., Reference Calabria, Costa, Green and Abutalebi2018; Gallo et al., Reference Gallo, DeLuca, Prystauka, Voits, Rothman and Abutalebi2022; Green & Abutalebi, Reference Green and Abutalebi2013) and included, bilaterally, the IFG pars opercularis, IFG pars orbitalis, IFG pars triangularis, caudal ACC, IPL (which includes inferior parietal and angular gyrus), supramarginal gyrus, caudate, thalamus, putamen and cerebellum.
AD-related ROIs included, bilaterally, the hippocampus, entorhinal cortex, parahippocampal gyrus, cornu Ammonis 1 (CA1) and subiculum, given that they typically show atrophy in AD, with the CA1 and subiculum subfields of the hippocampus appearing to be most prominently affected (e.g., Hari et al., Reference Hari, Kurt, Bayram, Kizilates-Evin, Acar, Demiralp and Gurvit2022; La Joie et al., Reference La Joie, Perrotin, de La Sayette, Egret, Doeuvre, Belliard, Eustache, Desgranges and Chételat2013; Mueller et al., Reference Mueller, Schuff, Yaffe, Madison, Miller and Weiner2010; Saad et al., Reference Saad, Alashwah, Alsafa and Dawoud2020). ROIs are shown in Figure 1.

Figure 1. ROIs from the Desikan–Killiany–Tourville cortical atlas (A) and the FreeSurfer subcortical atlas (B). ROIs also include the subiculum and CA1 subfields (not depicted here but located within the hippocampus). Language-related regions are shown in light gray and AD-related regions are shown in dark gray.
2.5 Statistical analysis
Statistical analyses were run in R (version 4.1.0; R Core Team, 2021). Step-wise and hierarchical multiple linear regression models were used to analyze the effects of diagnosis and language group on GMV of subcortical ROIs and cortical thickness of cortical ROIs. First, a base model (model 1) was run whereby GMV or cortical thickness was predicted by dataset (CIMA-Q vs COMPASS-ND), age, sex (male vs female) and immigration status (immigrant vs non-immigrant). Estimated total intracranial volume (eTIV) was also included for GMV analyses. Age and eTIV were standardized using the scale() function. Immigration status was not a significant predictor for any ROI and was excluded from subsequent regression models. Our predictors of interest were then included in a hierarchical fashion in the following order: (1) main effect of diagnosis (model 2), (2) main effect of language group (model 3) and (3) interaction effect of diagnosis and language group (model 4). See Table 3 for a summary of these statistical models. The Benjamini–Hochberg False Discovery Rate (FDR) correction was applied to correct for multiple models run per ROI. For each ROI, the anova() function was used to identify the model with the best fit. Pairwise comparisons were run using the emmeans() function.
Table 3. Summary of statistical models

Note: eTIV was also included in the models for the statistical analysis of regional GMV.
3. Results
Model 1 (demographic predictors only) provided the best fit for left and right ACC, left and right IFG pars orbitalis and pars triangularis, left and right caudate and putamen and left thalamus. In other words, we did not observe any effects of language group or diagnosis group for these regions. Model 2, indicating a main effect of diagnosis group, provided the best fit for left hippocampus, left subiculum, right thalamus, left and right parahippocampal gyri, left and right entorhinal cortex, left and right IFG pars opercularis, left and right supramarginal gyri, left and right IPL and left and right cerebellum (see Supplementary Tables 1–4). Model 3 did not explain significantly more variance compared to the other models for any ROIs, indicating no main effect of language group for any of the brain regions examined. Model 4 provided the best fit, indicating a language group by diagnosis group interaction, for the right hippocampus, right subiculum and the left and right CA1 (see Table 4). The observed effects are described next.
Table 4. Regression models indicating a language group by diagnosis group interaction effect

Cognitively unimpaired women monolinguals were the reference group for all regressions.
B, unstandardized beta coefficient; SE, standard error; eTIV, estimated total intracranial volume; SCD, subjective cognitive decline; MCI, mild cognitive impairment; AD, Alzheimer's disease.
*p < .05, **p < .01, ***p < .001.
3.1 Diagnosis group effects (model 2)
Generally, reduced GMV or cortical thickness was observed in older adults with AD. Pair-wise comparisons revealed that GMV or cortical thickness decreased from healthy older adults and those with SCD to MCI, and then to AD for left hippocampus, left subiculum, left entorhinal cortex and right IPL. In addition, GMV or cortical thickness was reduced for older adults with AD compared to the other diagnosis groups for left and right parahippocampal gyri, right entorhinal cortex, left supramarginal gyrus, left IPL and right thalamus.
Although reduced GMV or cortical thickness for the AD group was still observed, the pattern of effects for the remaining language-related areas was slightly more varied. Pair-wise comparisons revealed reduced cortical thickness in left and right IFG pars opercularis for the AD group compared to the SCD group. Reduced cortical thickness was observed in right supramarginal gyrus for the MCI and AD groups compared to the SCD group. Lastly, pair-wise comparisons revealed that volume of the left and right cerebellum was greater for healthy older adults compared to those with SCD and AD. Overall, the observed diagnosis group effects are consistent with the expected progression of atrophy in AD development.
3.2 Interaction effects (model 4)
Pair-wise comparisons examining language group differences within diagnosis groups revealed greater GMV in right hippocampus, right subiculum and left and right CA1 for bilinguals compared to monolinguals in the AD group only (p-values <.008; see Figure 2).Footnote 1 Pair-wise comparisons were also run examining differences between diagnosis groups within each language group. For all four ROIs (right hippocampus, right subiculum, left and right CA1), monolinguals showed reduced GMV for the AD group compared to the other three groups. In contrast, bilinguals showed reduced GMV in right hippocampus and left CA1 for the AD group compared to the CU and SCD groups, with no difference between the MCI and AD groups. Similarly, bilinguals with AD showed less GMV in right subiculum compared to CU bilinguals, with no differences between the SCD, MCI and AD groups. Furthermore, no diagnosis group differences were observed in right CA1 for bilinguals. Thus, although monolinguals showed reduced hippocampal volumes in AD compared to the other diagnosis groups, no difference in hippocampal volume was observed between bilinguals with MCI and AD (Figure 2).

Figure 2. GMV of left CA1 (A), right CA1 (B), right subiculum (C) and right hippocampus (D). Greater volumes are observed for bilinguals versus monolinguals with AD.
4. Discussion
The purpose of this study was to use structural neuroimaging to examine bilingualism-related brain and cognitive reserve in older adults with, or at risk for, AD. We did not observe any evidence of brain reserve in language-related brain regions or cognitive reserve in AD-related brain regions. However, we observed greater hippocampal volumes, suggesting a reserve in the form of brain maintenance, for bilinguals compared to monolinguals with AD. We discuss these findings in greater detail next.
First, we did not observe bilingualism-associated brain reserve in language-related regions. This contrasts with findings from Duncan et al.'s (Reference Duncan, Nikelski, Pilon, Steffener, Chertkow and Phillips2018) study of older adults with MCI and AD. Notably, Duncan et al. performed vertex-wise analyses, compared to our ROI-wise analyses. Thus, bilingualism-related brain reserve may be evident in smaller, more specific areas within language-related brain regions, but not in these regions as a whole. With such few studies on brain reserve in aging bilinguals, more research is needed to examine the association between bilingualism and brain reserve in healthy and pathological aging.
Second, in contrast to previous research (e.g., Costumero et al., Reference Costumero, Marin-Marin, Calabria, Belloch, Escudero, Baquero, Hernandez, Ruiz de Miras, Costa and Parcet2020; Duncan et al., Reference Duncan, Nikelski, Pilon, Steffener, Chertkow and Phillips2018; Schweizer et al., Reference Schweizer, Ware, Fischer, Craik and Bialystok2012), we did not find evidence of greater cognitive reserve (i.e., greater MTL atrophy despite group matching) for bilinguals compared to monolinguals. Instead, we observed greater hippocampal volume for bilinguals versus monolinguals with AD, which is consistent with research on healthy older adults and those with MCI and AD (Duncan et al., Reference Duncan, Nikelski, Pilon, Steffener, Chertkow and Phillips2018; Voits et al., Reference Voits, Robson, Rothman and Pliatsikas2022). Importantly, cognitive reserve refers to increased neural efficiency and/or flexibility. Although structural brain measures can provide evidence of cognitive reserve, measures of brain function would provide even stronger evidence. Thus, our future research will use functional neuroimaging methods to explore bilingualism and cognitive reserve.
The degree of bilingualism in the current sample may provide an explanation as to why we do not see evidence of brain and cognitive reserve in the current study. Research by Calabria et al. (Reference Calabria, Hernández, Cattaneo, Suades, Serra, Juncadella, Reñé, Sala, Lleó and Ortiz-Gil2020) suggests that active, but not passive, bilingualism may contribute to resilience (i.e., delayed onset of MCI symptoms and diagnosis). While we did not have detailed language information for all participants, a subset of participants in the current study (119 out of 364 participants) may be characterized as late L2 learners who do not regularly use their L2. Thus, the heterogeneity of bilingual language experience included in our bilingual sample may have contributed to the lack of brain and cognitive reserve observed in this study.
Our findings thus do not support the hypothesis that bilingualism contributes to cognitive reserve. However, the pattern of effects across diagnosis groups suggests brain maintenance associated with bilingualism. Brain maintenance refers to a reduced development of age-related brain changes and neuropathology over time, associated with preserved cognitive function (Stern et al., Reference Stern, Arenaza-Urquijo, Bartrés-Faz, Belleville, Cantilon, Chetelat, Ewers, Franzmeier, Kempermann and Kremen2020, Reference Stern, Albert, Barnes, Cabeza, Pascual-Leone and Rapp2023). Although cross-sectional, our data suggest that hippocampal atrophy increases in monolingual groups across the AD spectrum (i.e., from MCI to AD), but not in bilinguals. This is consistent with reports of better maintained brain structure over time for bilinguals compared to monolinguals with MCI (Costumero et al., Reference Costumero, Marin-Marin, Calabria, Belloch, Escudero, Baquero, Hernandez, Ruiz de Miras, Costa and Parcet2020). Longitudinal research is needed to further explore this association between bilingualism and maintained brain structure in AD, and how this might relate to change in cognitive function over time.
4.1 Limitations
We note several differences between this study and previous research that used structural neuroimaging to examine reserve in aging bilinguals. First, we used surface-based morphometry methods in FreeSurfer, instead of the gray matter volumetry of voxel-based morphometry methods. This allowed estimation of regional cortical thickness and volumes, as well as hippocampal subfield volumes. The current study has relatively large sample sizes, and language groups were strictly matched within diagnosis groups. We note that our participants were highly educated older adults (i.e., approximately 15 years of education on average compared to previous studies reporting 12 or 13 years of education: Abutalebi et al., Reference Abutalebi, Canini, Della Rosa, Green and Weekes2015a, Reference Abutalebi, Guidi, Borsa, Canini, Della Rosa, Parris and Weekes2015b; Duncan et al., Reference Duncan, Nikelski, Pilon, Steffener, Chertkow and Phillips2018; or 8 years of education: Costumero et al., Reference Costumero, Marin-Marin, Calabria, Belloch, Escudero, Baquero, Hernandez, Ruiz de Miras, Costa and Parcet2020), which may contribute to differences in our findings relative to these other studies (e.g., Gollan et al., Reference Gollan, Salmon, Montoya and Galasko2011). Moreover, the number of men and women was not balanced within our groups. Our sample consisted of a larger proportion of women in the CU and SCD diagnosis groups, with the reverse pattern in the AD groups. Thus, the current study differs from previous research on bilingualism and reserve in the type of imaging analysis, the measures used and the demographic characteristics of our sample.
A main limitation of this study is that bilingualism was used as a dichotomous variable. Given the available language data from COMPASS-ND and CIMA-Q, we were limited in our definition of bilingualism and could not analyze brain structure in relation to other language experience variables (e.g., AoA, proficiency or use). However, we provide information on such language experience factors for a subset of participants to facilitate comparisons with future research. Lastly, given the uneven number of men and women within each group, we were unable to examine sex differences in the current sample.
5. Conclusion
To our knowledge, this study is the first to use strict group matching and surface-based morphometry methods to examine bilingualism and reserve in both language-related and AD-related brain regions across a continuum of groups at risk for AD, including those with SCD. Although bilingualism was not associated with brain reserve in language-related areas, nor with cognitive reserve in AD-related areas, bilingualism appears to confer reserve in the form of brain maintenance in AD. Future research should explore the association between bilingualism and brain maintenance with longitudinal research methods.
Supplementary material
The supplementary material for this article can be found at https://doi.org/10.1017/S1366728924000221.
Data availability statement
The data used in this study come from the Quebec Consortium for the Early Identification of Alzheimer's disease (CIMA-Q)Footnote 2 and The Comprehensive Assessment of Neurodegeneration and Dementia Study (COMPASS-ND)Footnote 3 of the Canadian Consortium on Neurodegeneration in Aging (CCNA). The CIMA-Q data are available to the research community to address research questions related to dementia and aging, upon approval by the User Access Committee. Access is limited to CIMA-Q members. However, membership is open to all scientists interested in dementia research. Detailed information on the procedure for submitted a data access request can be found on the CIMA-Q website (http://www.cima-q.ca/). The baseline COMPASS-ND data are available to CCNA researchers upon application to the Publications and Data Access Committee (PDAC). As of March 2025, non-CCNA researchers may be granted access to the data upon submission of a data access request to the PDAC. Information on COMPASS-ND data access can be found on the CCNA website (https://ccna-ccnv.ca/compass-nd-study/).
Acknowledgements
Portions of this work were supported by a grant awarded to NAP from the Natural Sciences and Engineering Research Council Discovery Grant Program [RGPIN-2018-04393]. NAP is a member of Team 17 which is a part of the Canadian Consortium on Neurodegeneration in Aging (CCNA). The CCNA is supported by a grant from the Canadian Institutes of Health Research (CIHR) with funding from several partners. Parts of the data used in this article were obtained from the Québec Consortium for the Early Identification of Alzheimer's disease (CIMA-Q; cima-q.ca). The CIMA-Q investigators contributed to the design, protocols and implementation of the study, as well as the collection of clinical, cognitive and neuroimaging data and biological samples. A complete list of the CIMA-Q investigators can be found at www.cima-q.ca. The CIMA-Q is supported by the Fonds de recherche du Québec–Santé (FRQS) – Pfizer Innovation Program, FRQ cohort funds, the Quebec Network for Research on aging, the Fondation Courtois (NeuroMod project), the Consortium for the Neurodegeneration associated with Aging, CIHR and the Fondation Famille Lemaire. We also acknowledge the Cognition, Aging and Psychophysiology Lab, with special thanks to Sana Rehan, for their contributions to data organization and quality control of MRI data.
Competing interests
None.