Introduction
Bopyrid isopods are obligate parasites that typically infest the gills or abdomens of decapod crustaceans. This infestation leads to stunted growth and a decrease in the reproductive capacity of the host (Beck, Reference Beck1980a; O'Brien and Van Wyk, Reference O'Brien, Van Wyk and Wenner1985; Calado et al., Reference Calado, Bartilotti, Goy and Dinis2008; Sherman and Curran, Reference Sherman and Curran2015; Corral et al., Reference Corral, Henmi, Shiozaki and Itani2019; Corral et al., Reference Corral, Henmi and Itani2021). Unlike other parasitic isopods, bopyrids have a complex life cycle that involves host changes from a small intermediate host (calanoid copepods) to a larger definitive host (decapod crustaceans) (Schädel et al., Reference Schädel, Perrichot and Haug2019). They develop through three distinct larval stages: epicaridium, microniscium, and cryptoniscium (Anderson and Dale, Reference Anderson and Dale1981; Williams and Boyko, Reference Williams and Boyko2012).
Bopyrid isopods exhibit extreme sexual dimorphism. Dwarf males resemble free-living isopods, while females, which are much larger in size, have a modified and asymmetrical body (Williams and Boyko, Reference Williams and Boyko2012). Females have a non-calcified exoskeleton, eyes that are reduced or absent, mouthparts adapted for piercing and sucking, an enlarged brood pouch that is either partially or fully covered by oostegites, and reduced pereiopods for attachment and limited movement (Cash and Bauer, Reference Cash and Bauer1993; Itani et al., Reference Itani, Kato and Shirayama2002; Lester, Reference Lester and Rohde2005).
It is evident that the morphology of female bopyrid isopods is adapted to parasitism, but understanding of the specialised functions is limited. This is due to their small size and cryptic lifestyle, which restricts their examination and observation. In particular, little is known about the functions of maxillipeds and the first oostegites, structures often used in the taxonomy and classification of the family Bopyridae (Markham, Reference Markham1985; An et al., Reference An, Boyko and Li2015). The few available studies have noted rapid movements of maxillipeds and first oostegites in ovigerous female parasites (Beck, Reference Beck1980b; Cash and Bauer, Reference Cash and Bauer1993) and suggested that these movements aid in brood ventilation (Beck, Reference Beck1980b; Cericola and Williams, Reference Cericola and Williams2015). However, this hypothesis remains untested, except for the behavioural observations made by Cericola and Williams (Reference Cericola and Williams2015) for the abdominal parasite Athelges takanoshimensis. To the best of our knowledge, no study has been conducted on bopyrid isopods infesting the host branchial chamber. Given the differences in parasite attachment sites, it is reasonable to assume that behavioural patterns may vary between species. Consequently, most of these studies reported movement of the first oostegites and maxillipeds in ovigerous female parasites, but observation of non-ovigerous females for comparison is lacking; thus, further studies are needed. Information on the functional morphology of bopyrid isopods can facilitate our understanding of their adaptive strategies for parasitism.
In the present study, the function of maxillipeds and oostegites of the branchial parasite Bopyrus crangorum (Fabricius, 1798) infesting the caridean shrimp Palaemon serrifer was investigated. Previously, this species was known as Bopyrus squillarum (Latreille, 1804), but revision of nomenclature has rendered this name invalid (Boyko and Williams, Reference Boyko and Williams2023; Boyko et al., Reference Boyko, Bruce, Hadfield, Merrin, Ota, Poore and Taiti2024). This species was used because its behaviour is easily visible through the transparent carapace of the host (Burgos and Itani, Reference Burgos and Itani2023). The aim of this study was to clarify the function of the first oostegites and maxilliped in bopyrid isopods infesting the branchial chamber of a caridean shrimp through behavioural observation and morphological examination. Specifically, (1) to describe the pattern and quantify the movement of maxillipeds and first oostegites, and (2) to determine if movement frequency differs between non-ovigerous and ovigerous females and across different embryonic stages.
Materials and methods
Sample collection and behavioural observations
Infested host shrimp, P. serrifer, were collected from the tidal zone of Uranouchi Inlet, Tosa Bay, Kochi, Japan (33°26′0.46″N, 133°26′21.75″E) using a handheld dip net with a 1 mm mesh. Infested shrimps were identified based on the presence of distinct bulges in the branchial chamber and the presence of male and female B. crangorum (Figure 1). The female B. crangorum was positioned diagonally inside the branchial chamber of the host P. serrifer, with its head directed posteriorly in relation to the host. The dorsal side of the female parasite faced the gills, whereas the ventral side was pressed against the host's branchiostegite. In relation to the host, the dorsolateral side of the female parasite was shorter than the ventrolateral side. The dwarf male of B. crangorum was attached between the pleopods of the female parasite's abdomen, with its head oriented towards the anterior end of the female. The shrimp were kept in individual jars covered with fine mesh nets and placed in an 18-l aquarium filled with filtered and aerated seawater. The water was maintained at a salinity of 28–30 and 24–26°C, and the shrimp were fed commercial flakes (TetraFin®; Tetra, Blacksburg, VA, USA) daily.
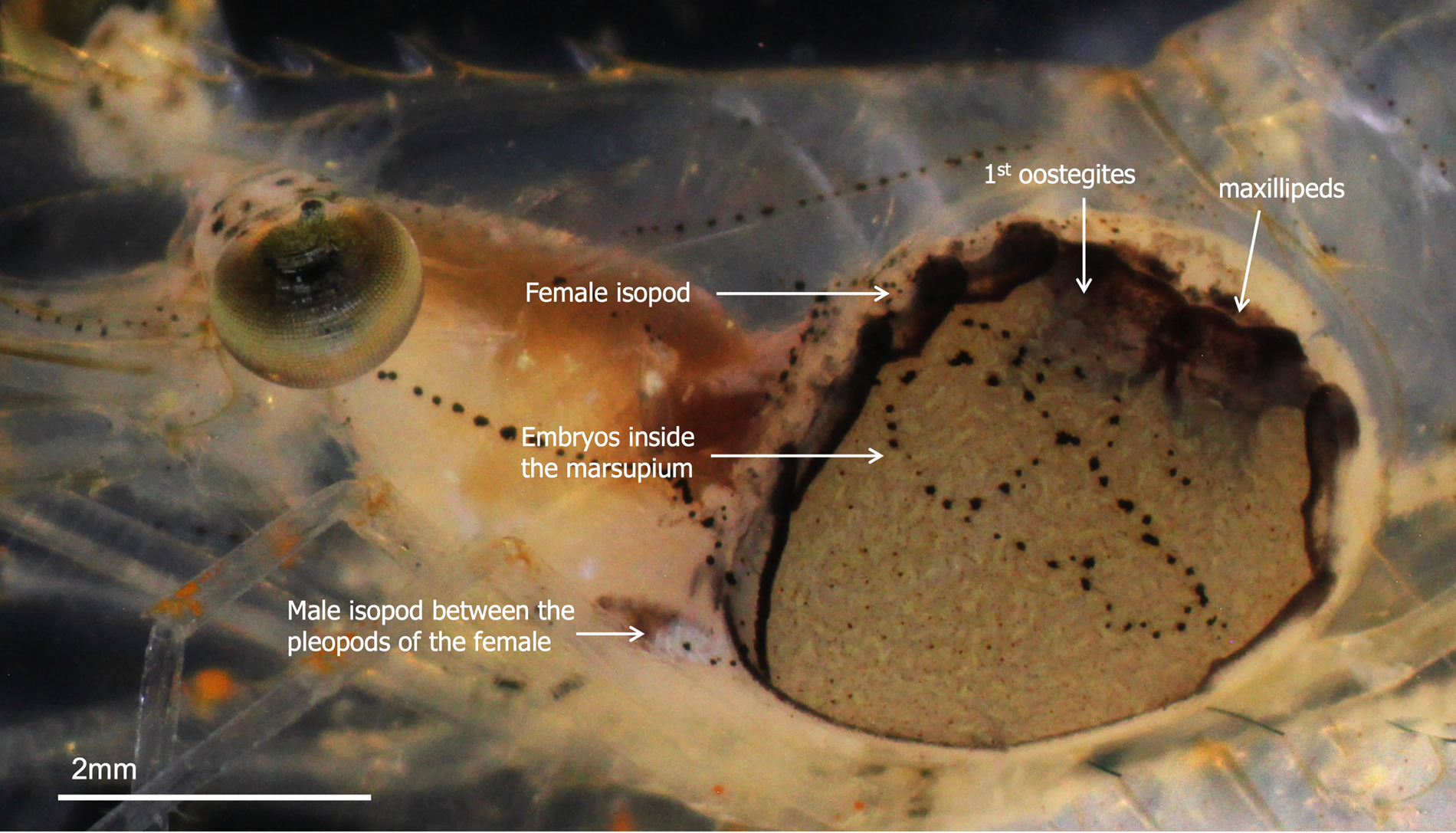
Figure 1. Positions of male and female Bopyrus crangorum inside the branchial chamber of Palaemon serrifer.
The morphology of B. crangorum was studied under a Nikon SMZ1000 dissecting microscope (Nikon, Tokyo, Japan). Digital photographs were taken using a Canon EOS KissF digital camera (Canon, Tokyo, Japan), mounted on the microscope. The stacks of several frames of different focal planes were merged using Affinity Photo (Serif (Europe) Ltd., 2019). The carapace length of the host and body length of male female parasites were measured using ImageJ software (Schindelin et al., Reference Schindelin, Rueden, Hiner and Eliceiri2015). Prior to each observation, the developmental stages of the isopod embryo were noted according to the embryonic coloration and morphology described by Beck (Reference Beck1980c), Cash and Bauer (Reference Cash and Bauer1993), and Sherman and Curran (Reference Sherman and Curran2015), as follows: egg; translucent or white; embryo I: yellowish, embryo reniform, and tissue around the yolk segmented; embryo II: tan with brown pigmentation, body segmentation well-developed but appendages not free, with little or no yolk; epicaridium larva: dark grey or black, embryo with free appendages, and well-developed eyes.
The movement of the maxillipeds and first oostegites was recorded by transferring the infested shrimp into a petri dish with a sponge that had a rectangular cut in the middle, following the method described in Burgos and Itani (Reference Burgos and Itani2023). Recordings were made using an Olympus Tough TG-6 digital camera (Olympus, Tokyo, Japan) mounted on a Nikon SMZ 1000 dissecting microscope. In the first set of recordings, the movement sequence of the maxillipeds for each female parasite was recorded three times at 480 frames per second (fps) for 20 s. In the second set, the movement of the first oostegites was recorded three times at 25 fps for 5 min. To observe the water movement created by the maxillipeds and the first oostegites, infested shrimp were transferred to a Petri dish filled with methylene blue water solution (Otanishiki Koi Co., Ltd., Suminoe City, Osaka, Japan). The infested shrimp were incubated in the solution until the dye residue was observed inside the brood chamber of the parasite. The movement of the methylene blue residue inside the brood chamber of the female was recorded at 25 fps.
Statistical analyses
The frequency of maxilliped beating and oostegite flapping between the ovigerous and non-ovigerous female parasites was compared using the Wilcoxon Signed Rank test because the data had unequal sample sizes and were not normally distributed. The significant differences in the frequency of maxillipeds beating and oostegite flapping between developmental stages were compared using the nonparametric Kruskal Wallis tests with Steal Dwass multiple comparisons. All analyses were performed using JMP version 14 (SAS Institute Inc., 2018).
Results
The female B. crangorum had five pairs of oostegites that partially covered the marsupium (Figure 2A). The first oostegites were symmetrical and articulated; the anterior lobe was ovate, whereas the posterior lobe was subtriangular, with a slightly curved inward posterolateral point (Figure 2A, B). The internal surface of the first oostegites had an internal ridge that bears simple ventral projections, whereas on the dorsal face, the joints between the anterior lobes were folded inward (Figure 2B–E). Underneath the first oostegites was the pair of maxillipeds, which were trapezoidal in shape with a non-articulated palp bordered by sparse setae (Figure 2C). The fifth pair of oostegites was elongated and overlapped, completing the posterior end of the marsupium (Figure 2A). The posterior margins of the fifth oostegites were lined with long setae (Figure 2D).
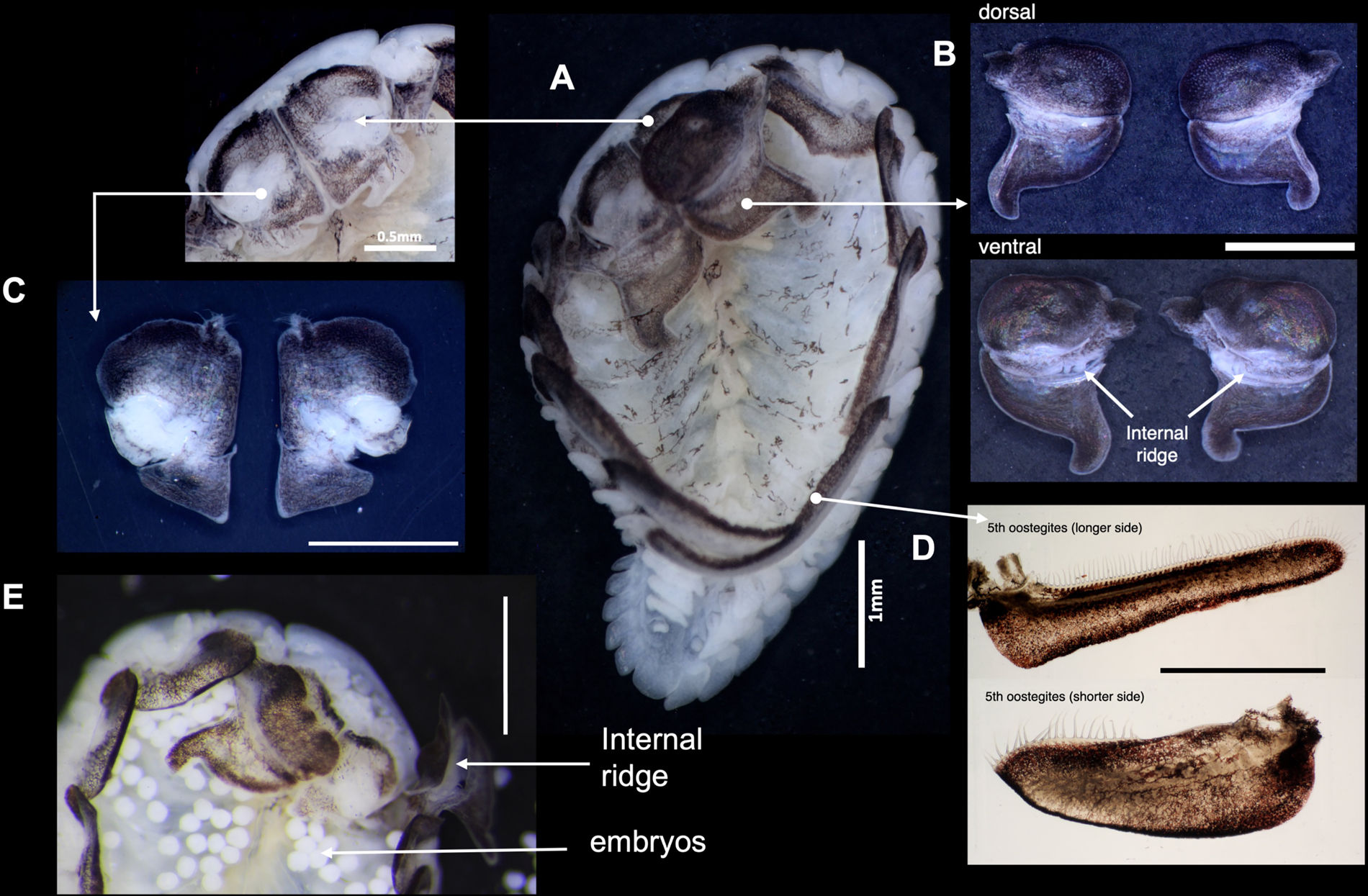
Figure 2. Morphology of female B. crangorum. (A) Ventral view of the female showing the location of each morphological structure; (B) dorsal and ventral views of left and right first oostegites; (C) dorsal view of left and right maxillipeds; (D) left and right fifth oostegites; (E) anterior region of the ovigerous female showing the accumulation of embryos, with the right first oostegite lifted to show the internal ridge. Scale bars: B–E, 1 mm.
Video observation of the female parasites at 480 fps revealed continuous and synchronous beating of maxillipeds in both ovigerous and non-ovigerous female parasites. The left and right maxillipeds showed lateral abduction during forward pumping, creating an opening, and adduction during posterior pumping (Figure 3). The beating of the maxillipeds concurrently generates water pressure, causing the folded posterior lobe of the first oostegites to extend posteriorly, resulting in a power-stroke flapping motion (Figure 4B). When the posterior lobe of the first oostegites was fully extended, pumping of the maxillipeds stopped for approximately 21 s and resumed when it was retracted (Supplementary Video S1).
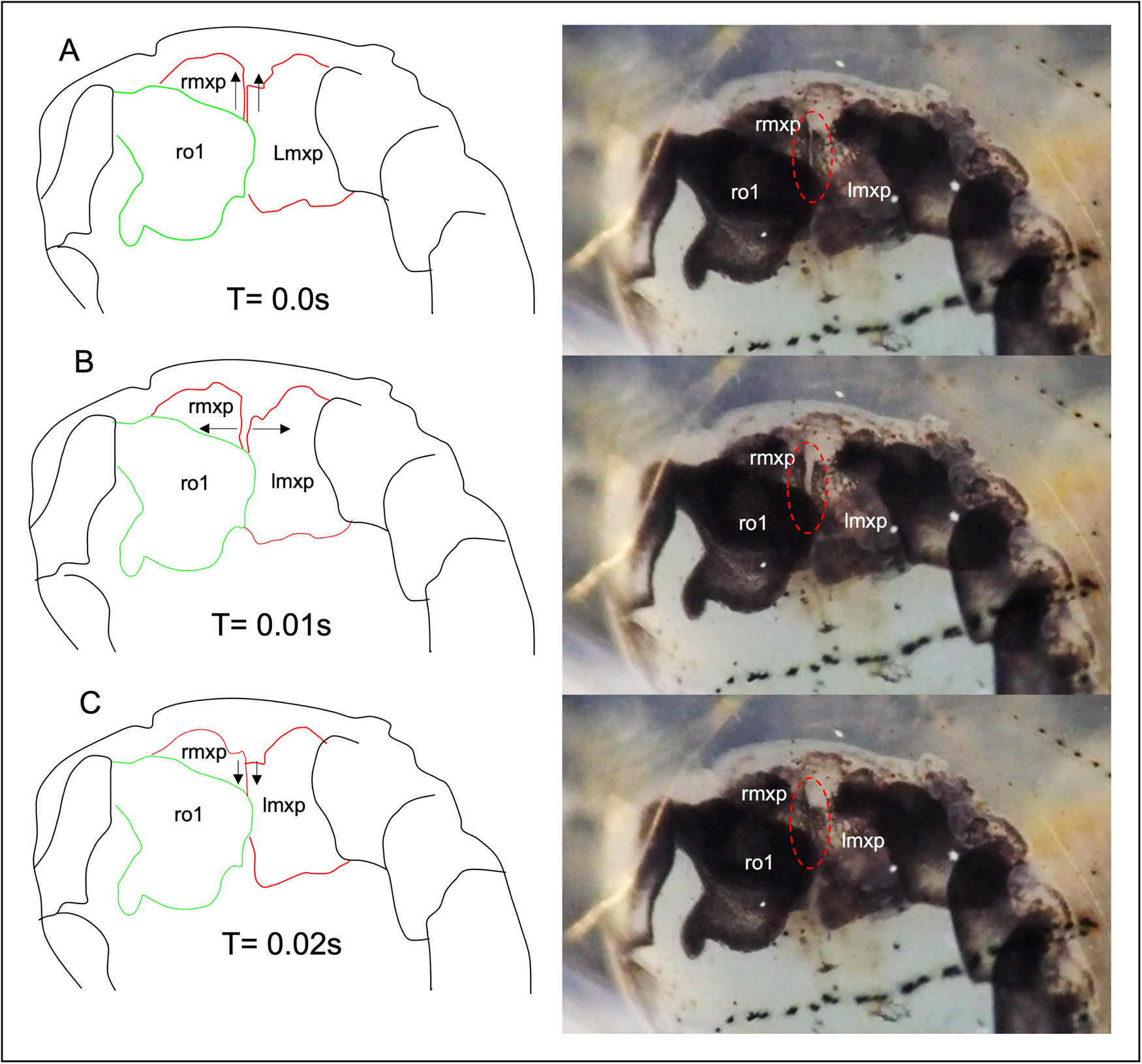
Figure 3. The movement pattern of the maxillipeds outlined from the frame-grabbed video images. (A) Maxilliped in the resting position; (B) The maxillipeds are abducted sideways, creating an opening; (C) The maxillipeds are adducted together when they pump posteriorly; rmxp, right maxilliped; lmxp, left maxilliped; ro1, right first oostegite. Note that the left oostegite was removed. See also Supplementary Video S1.

Figure 4. The movement pattern of the right first oostegite outlined from the frame-grabbed video images. (A) The first oostegite during maxilliped pumping with the posterior lobe in a retracted position; (B) The posterior lobe of the first oostegite is fully extended during the flapping motion; rmxp, right maxilliped; lmxp, left maxilliped; ro1, right first oostegite. Note that the left oostegite was removed.
The rhythmic beating of the maxillipeds propels water into the brood chamber through the opening between the posterior fifth oostegites and exits through the anterior first oostegites. Water drawn into the marsupium during maxilliped pumping was clearly visible in the ovigerous female parasites (Figure 5). The maxilliped pumping moved embryos anteriorly and accumulated them under the first oostegites (Figure 5A), whereas the flapping motion of the first oostegites pushed embryos posteriorly (Figure 5B). The embryos were blocked by the internal ridges of the first oostegites during the beating of the maxillipeds (Figure 5A). Meanwhile, extension of the posterior lobe lifted the anterior lobe of the first oostegites, preventing embryos from escaping the marsupium. Posteriorly, the embryos were blocked by elongated and overlapping pairs of fifth oostegites (Figure 5B).

Figure 5. Video images of ovigerous female B. crangorum incubating epicaridium larvae. (A) Movement of embryo and water during maxillipeds beating, as shown in dashed arrows; (B) Movement of the embryos during the extension of the posterior lobe of the first oostegites. See also Supplementary Video S2.
The frequency of maxillipeds beating was significantly higher in ovigerous (4.9 ± 1.6 beats s−1) than non-ovigerous (2.0 ± 2.1 beats s−1) female parasites (Wilcoxon test, z = −3.49829, P < 0.0005) (Figure 6A). Female parasites incubating eggs (3.0 ± 1.5 beats s−1) exhibited a significantly lower frequency of maxilliped beating compared to those incubating epicaridium larvae (6.1 ± 0.5 beats s−1) (Kruskal–Wallis test, df = 3, P < 0.0066, Steel Dwass test between epicaridium and egg stage, P < 0.0254) (Figure 6B). The flapping of first oostegites was also significantly higher in ovigerous (7.5 ± 4.2 flaps mins−1) compared to non-ovigerous (3.8 ± 2.9 flaps mins−1) female parasites (Wilcoxon test, z = −2.49671, P < 0.0125) (Figure 7A). In contrast, no significant difference was detected in the frequency of oostegite flapping (Kruskal–Wallis test, df = 3, P < 0.3613) among the female parasites carrying embryos at different developmental stages (Figure 7B). The highest frequency of first oostegites flapping was recorded in the egg stage (9.1 ± 4.9 flaps mins−1) and the lowest in females brooding epicaridium larvae (5.2 ± 3.0 flaps mins−1) (Figure 7B). The mean interval between each beat cycle of the maxillipeds was one second, whereas the mean interval between each flap of the first oostegites was 16 s (=0.3 min).
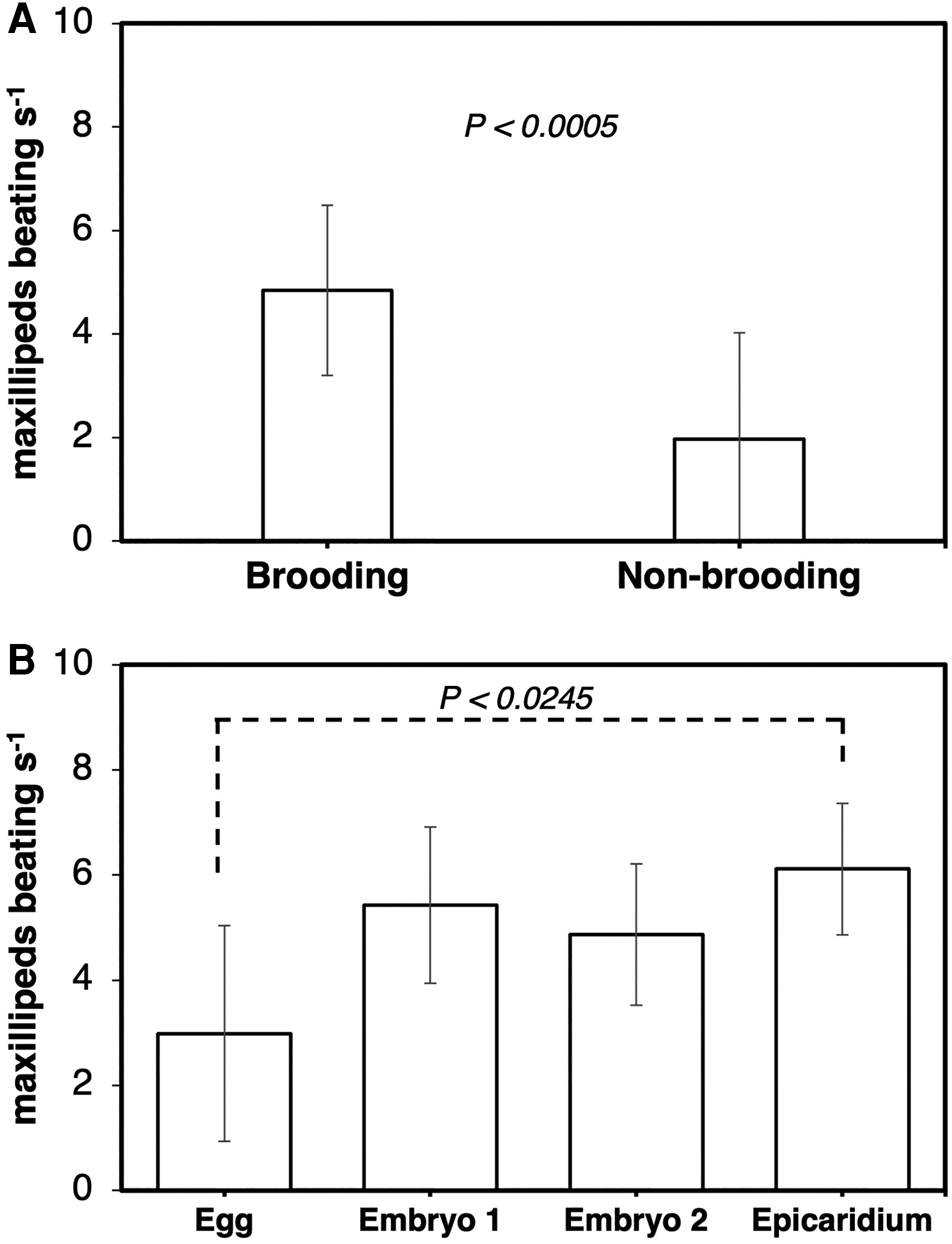
Figure 6. Frequency of maxilliped beating per second at 480 frames per second in female B. crangorum. (A) ovigerous (N = 28) vs non-ovigerous (N = 11); (B) between ovigerous females carrying embryos at each developmental stage (N = 7 per stage).
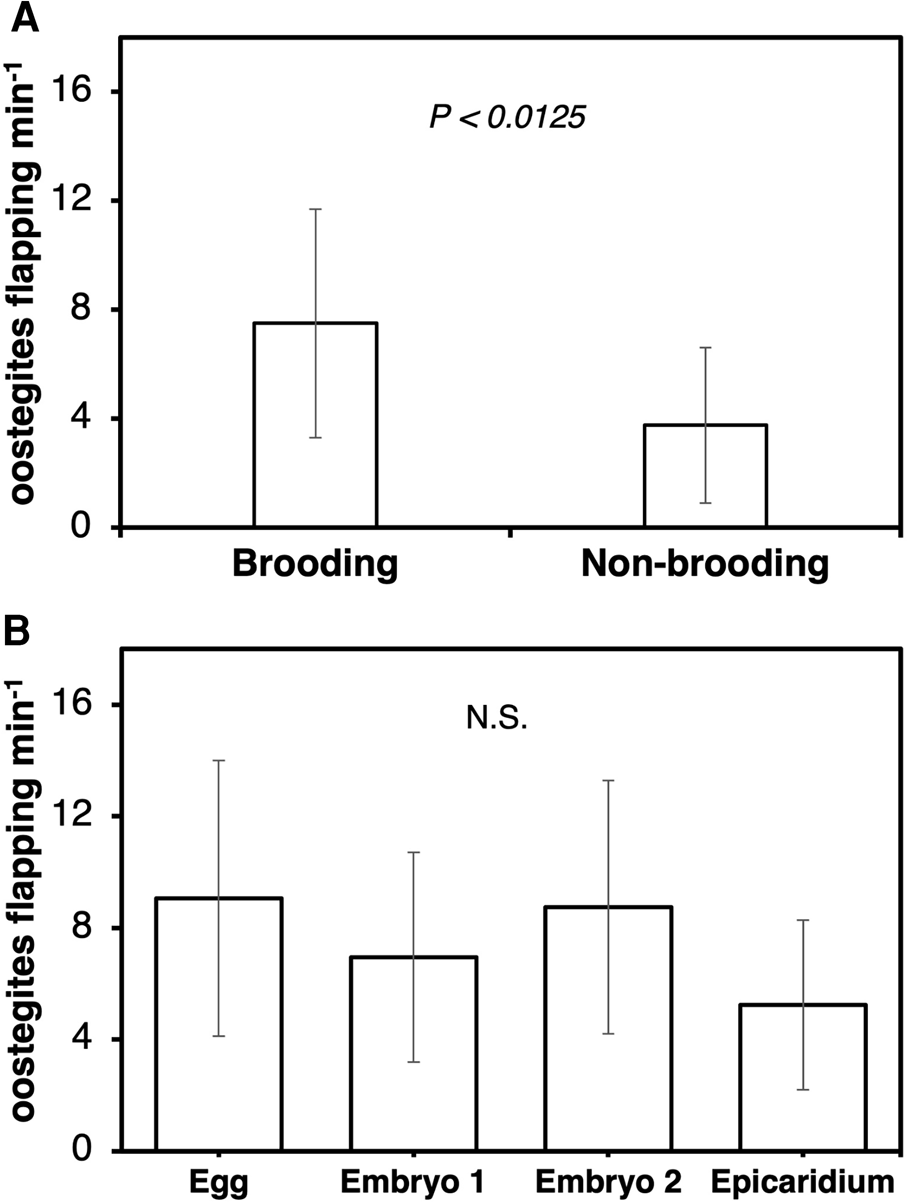
Figure 7. Frequency of first oostegite flapping per minute at 25 frames per second in female B. crangorum. (A) ovigerous (N = 28) vs non-ovigerous (N = 9); (B) between ovigerous females carrying embryos at each developmental stage (N = 7 per stage).
Microscopic observation using dye showed that the methylene blue residue entered the brood chamber of the female parasite through the opening between the fifth oostegites. This was shown by the scattered dye residues in the posterior region of the marsupium (Figure 8A). The movement of the first oostegites and the maxillipeds causes the dye residues to move anteriorly, accumulating at the junction of the anterior and posterior lobes of the first oostegites (Figure 8B, C). Eventually, the residue was expelled from the female brood chamber through the dorsal surface or beneath the first oostegites. This was shown by the decreasing size of the accumulated residues on the first oostegites (Figure 8D, E). The dye was then transported by the water current generated by the scaphognathite of the host shrimp (Figure 8F; Supplementary Video 3). The average time for the female parasites to eliminate the dye residues was 51.2 ± 18.8 min (N = 10). Along with the dye residue, some of the dyed embryos of B. crangorum were also expelled through the first oostegites by the movement of the maxillipeds and first oostegites (Supplementary Video S3).
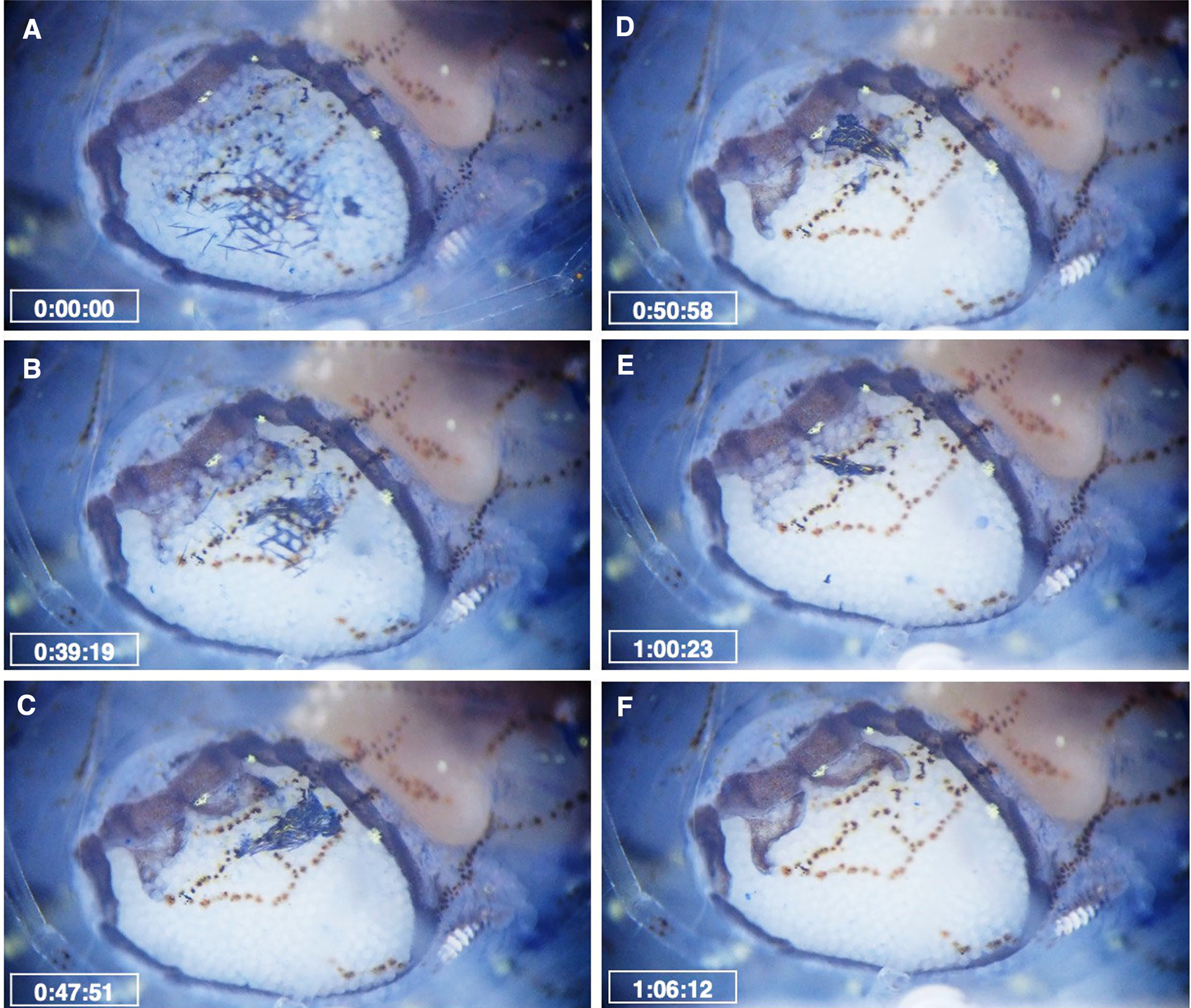
Figure 8. Movement of methylene blue residue inside the brood chamber of the female B. crangorum. Time elapsed after the incubation of the infested shrimp in the solution was provided. (A) Dye residues are scattered between the embryos; (B) Dye residues move anteriorly due to the movement of maxillipeds and first oostegites; (C–D) Dye residues accumulate on the first oostegites; (E) Some of the dye residues were already expelled from the brood chamber; (F) All of the residues were expelled, and the brood was clean. See also Supplementary Video S3.
Discussion
The findings of the present study confirmed that maxilliped beating and oostegite flapping facilitate brood ventilation in female bopyrid isopods, as previously reported (Beck, Reference Beck1980a; Cash and Bauer, Reference Cash and Bauer1993; Cericola and Williams, Reference Cericola and Williams2015). The ventilation of the brood was further facilitated by the beating of the host scaphognathite, which circulates water over the parasites (Beck, Reference Beck1980b). However, unlike previous reports, which only made observations on ovigerous females, movements were recorded in both ovigerous and non-ovigerous females, but with significantly higher frequencies in ovigerous individuals. This difference may be attributed to the increased oxygen demand of embryos during brooding, consistent with observations in other crustaceans (Baeza and Fernández, Reference Baeza and Fernández2002; Fernández et al., Reference Fernández, Pardo and Baeza2002). Moreover, the increasing trend in maxilliped beating with embryonic development, particularly in females brooding epicaridium larvae, indicates much higher oxygen consumption in late-stage embryos, probably due to higher metabolic demands and increased cell differentiation (Dick et al., Reference Dick, Faloon and Elwood1998; Baeza and Fernández, Reference Baeza and Fernández2002; Brante et al., Reference Brante, Fernández, Eckerle, Mark, Pörtner and Arntz2003).
The short interval between the incubation and intermoult periods of female parasites might be the reason behind the continuous ventilatory movement in non-ovigerous female parasites. This is because the reproduction of bopyrid isopods is closely synchronised with the moulting cycle of the host (Cash and Bauer, Reference Cash and Bauer1993; Burgos and Itani, Reference Burgos and Itani2023). The brooding period is typically longer than the intermoult period of the parasites. For instance, in B. crangorum, the incubation period typically lasts 8–12 days, whereas the intermoult period lasts only 1–4 days (Burgos and Itani, Reference Burgos and Itani2023). Continuous ventilation might also be a residual behaviour owing to delayed hormonal or neural signals after hatching, similar to that seen in crabs (Rittschof et al., Reference Rittschof, Forward and Mott1985; de Vries and Forward, Reference de Vries and Forward1991). However, further research is needed to confirm this hypothesis and to determine whether similar mechanisms occur in bopyrids. Another possible explanation for ventilatory movement in non-ovigerous females could be the respiratory function of the oostegites. However, this is unlikely since the body surface, particularly the five pairs of pleopods, functions as ‘gills’ in aquatic isopods (Bubel and Jones, Reference Bubel and Jones1974; Wägele, Reference Wägele1982; Hessler and Strömberg, Reference Hessler and Strömberg1989; Alexander, Reference Alexander1991). Furthermore, this has not been studied in bopyrid isopods and is almost certainly not true for males, which lack oostegites.
Although the attachment sites differ, posterior-to-anterior water movement due to maxilliped beating resembles that of abdominal bopyrid isopods (Cericola and Williams, Reference Cericola and Williams2015). The authors of that study suggested that the movement of the maxillipeds was responsible for the flapping of the first oostegites, although the frequency of this movement was not quantified. Additionally, their observations were limited to ovigerous female parasites. Cericola and Williams (Reference Cericola and Williams2015) mentioned the movement of haemolymph concurrent with maxilliped pumping, which was not observed in the present study.
The present observation showed that the first oostegites move less frequently than the maxillipeds, suggesting a lesser role in the pumping of water into the marsupium. Instead, it appears that the first oostegites facilitate ventilation by actively ‘stirring’ and ‘rearranging’ the brood inside the marsupium (Heinrich Janssen and Hoese, Reference Heinrich Janssen and Hoese1993). This may explain the absence of an increasing trend in the frequency of oostegite flapping across different embryonic developmental stages. The morphology of the first oostegites in B. crangorum, particularly the movable posterior lobe, is highly adapted for this function. With some variations in shape and length, the movable posterior lobe of the first oostegites is consistent across all families of bopyrid isopods. Hence, a comparable function can be inferred for other species, as in A. takanoshimensis (Cericola and Williams, Reference Cericola and Williams2015).
The function of maxillipeds and oostegites in ventilating the brood chamber has been reported in other isopods. In aquatic species, such as those in Asellota, Idotea, and several species of sphaeromatids, ventilation is facilitated by the beating of modified maxillipeds (Harrison, Reference Harrison1984; Johnson et al., Reference Johnson, Stevens and Watling2001). In janiroidean isopods, brood ventilation occurs via rhythmic expansion and contraction of the brood chamber due to the movements of the oostegites or pereopodal coxae to which they are attached (Hessler and Strömberg, Reference Hessler and Strömberg1989). These structures produce a posterior-to-anterior current through a specific opening at the posterior end of the marsupium (Heinrich Janssen and Hoese, Reference Heinrich Janssen and Hoese1993; Johnson et al., Reference Johnson, Stevens and Watling2001). In parasitic isopods that brood eggs, such behaviour has never been documented, except for bopyrid isopods. A similar posterior-to-anterior movement of water was also noted in this study.
In B. crangorum, maxilliped movement was likely caused by contraction of muscular attachment (Figure 2C), as suggested in A. takanoshimensis (Cericola and Williams, Reference Cericola and Williams2015). In addition to the water pressure created by the maxillipeds, the extension of the first oostegites may be due to muscle contraction between the lobes (Figure 2B). In other arthropods, the presence of elastic resilin in the joint system is thought to be responsible for the rapid movement of appendages (Burrows, Reference Burrows2009; Michels et al., Reference Michels, Appel and Gorb2016). Such protein might also be responsible for the movement of the maxillipeds and first oostegites; however, further examination is needed to confirm.
The results of microscopic dye observation suggest that the movement of maxillipeds and first oostegites not only facilitates ventilation but also serves as a grooming mechanism for female parasites. Embryo grooming is commonly reported in stenopodidean and caridean shrimps (Bauer, Reference Bauer1979, Reference Bauer1981), as well as in anomuran crabs (Bauer, Reference Bauer1981; Pohle, Reference Pohle, Felgenhauer, Watling and Thistle1989; Förster and Baeza, Reference Förster and Baeza2001). In contrast, reports on embryo cleaning in peracarids, particularly isopods, are limited. In caridean shrimps, females typically use the grooming chelipeds to brush and pick between the embryos (Bauer, Reference Bauer1979, Reference Bauer1981), whereas anomurans use the fifth pereopods as gill-grooming chelipeds (Pohle, Reference Pohle, Felgenhauer, Watling and Thistle1989; Förster and Baeza, Reference Förster and Baeza2001). Since continuous ventilation also introduces particulate matter into the brood, grooming is important to prevent biofouling and the settlement of parasites (Bauer, Reference Bauer1979, Reference Bauer1981). Experimental studies have shown that the removal of grooming appendages can lead to a significant increase in embryo mortality during incubation (Bauer, Reference Bauer1981; Förster and Baeza, Reference Förster and Baeza2001). To the best of our knowledge, this is the first observation of embryo grooming in parasitic isopods, particularly bopyrid isopods. Additionally, the long setae in the posterior margin of the fifth oostegites help prevent the entry of particulate matter into the brood chamber. This is evident in the accumulation of dye residues on the posterior margin of the fifth oostegites, consistent with previous observations (Cericola and Williams, Reference Cericola and Williams2015). Interestingly, female B. crangorum were also observed to expel dyed embryos from the marsupium. The embryos might be dead before or during staining, as dead cells retain the blue stain more than living cells due to a lack of enzyme activity that can reduce the dye (Painting and Kirsop, Reference Painting and Kirsop1990; Zetsche and Meysman, Reference Zetsche and Meysman2012; Kwolek-Mirek and Zadrag-Tecza, Reference Kwolek-Mirek and Zadrag-Tecza2014). The ejection of non-viable embryos has been reported in crabs and amphipods, but not in isopods. The mechanism underlying the possible selective removal of these embryos is unknown. Earlier studies have indicated that manipulating the brood with grooming appendages and chelae leads to the incidental removal of nonviable embryos (Fleischer et al., Reference Fleischer, Grell, Høeg and Olesen1992). The amphipod, Crangonyx pseudogracilis inserts its mouth into the brood to check the condition of embryos and selectively expel non-viable embryos (Dick et al., Reference Dick, Faloon and Elwood1998). In the current study, the female parasites may have recognised the dyed embryos as non-viable and expelled them together with the dye residues. The grooming function may also explain the ventilatory movements of maxillipeds and first oostegites in non-ovigerous female parasites to prevent biofouling of the marsupium.
In summary, the dual function of maxillipeds and first oostegites in ventilation and grooming appears to be an adaptive strategy to compensate for the reduced pereopods associated with parasitic lifestyles. This adaptation is crucial for embryo survival and increases the likelihood of future host infestation. While the frequency of maxilliped and first oostegite movements may vary across species, it would be interesting to observe if similar grooming behaviour occurs in abdominal bopyrid isopods, given their attachment site to the host. The findings of the present study significantly contribute to the understanding of the ecological dynamics and parasitic adaptations of bopyrid isopods. Nonetheless, further research is necessary to fully comprehend the functional morphology of bopyrid isopods, particularly their feeding behaviour.
Supplementary material
The supplementary material for this article can be found at https://doi.org/10.1017/S0025315424000596
Acknowledgements
We wish to thank the members of the Laboratory of Marine Symbiotic Biology, Kochi University, for providing assistance with the field sampling. We are also grateful to Dr Y. Henmi (Kyoto University) and Dr S. Kappalli (Central University of Kerala), whose feedback substantially improved the investigation.
Author Contributions
LAB performed the laboratory observations, data analyses, and writing the original draft. GI participated in the conceptualization, the interpretation of the results, and revision of the manuscript. All authors read and approved the final manuscript.
Financial Support
This work was supported by JSPS KAKENHI grant number JP21K05635 and JSPS Bilateral Program grant number JPJSBP120207715 to GI.
Competing interest
None.
Ethical Standards
Not applicable.
Data availability
All data underlying the results are available as part of the manuscript. Additional data can be shared on request.