Zearalenone (ZEA) is a non-steroidal oestrogenic mycotoxin synthesised by a variety of Fusarium fungi, including F. graminearum (Gibberella zeae), F. culmorum, F. cerealis, F. equiseti, F. crookwellense and F. semitectum, which are common soil fungi, in temperate and warm countries, being regular contaminants of cereal crops worldwide( Reference Richard 1 – Reference Tabuc, Marin and Guerre 5 ). Toxin production mainly takes place before harvest, but it may also occur after harvest if the crop is not handled and dried properly( 6 ). ZEA is also commonly found as a contaminant of stored grains, and the toxin has been detected in cereal products such as flour, malt, soyabeans and beer.
It is well known that ZEA has oestrogenic effects on mammals, being a risk factor from both public health and agricultural perspectives. The major target of this mycotoxin is the reproductive system( Reference Tiemann and Dänicke 7 ) because of the structural similarity of ZEA and some of its metabolites with the oestrogen hormones. It has been shown that ZEA competitively binds to oestrogen receptors and causes alterations in the reproductive tract of laboratory (mice, rats, guinea pigs, hamsters and rabbits) and domestic animals in a number of in vitro or in vivo systems( Reference Kuiper, Lemmen and Carlsson 8 – Reference Kuiper-Goodman, Scott and Watanabe 10 ). ZEA has also been shown to be genotoxic, inducing DNA-adduct formation in in vitro cultures of bovine lymphocytes( Reference Lioi, Santoro and Barbieri 11 ), DNA fragmentation and micronucleus production in cultured cells( Reference Abid-Essefi, Baudrimont and Hassen 12 , Reference Abid-Essefi, Ouanes and Hassen 13 ). In addition, ZEA has been shown to be immunotoxic( Reference Atkinson and Miller 14 – 16 ) and hepatonephrotoxic( Reference Ouanes, Abid and Ayed 17 , Reference Bouaziz, Martel and Sharaf el dein 18 ) and an enhancer of lipid peroxidation( Reference Zourgui, Golli and Bouaziz 19 ). Once ZEA enters the body, it is mainly metabolised in the liver( Reference Kiessling and Pettersson 20 ), which seems to be one of the main targets of the toxin( Reference Jiang, Yang and Yang 21 ), and its derivatives discharge in the kidney( Reference Hou, Zhao and Xiong 22 ). ZEA is able to induce liver lesions with subsequent development of hepatocarcinoma( 23 ) and alterations of some enzymatic parameters of the hepatic function in rats( Reference Maaroufi, Chekir and Creppy 24 ), rabbits( Reference Conkova, Laciakova and Pastorova 25 ) and gilts( Reference Wang, Zhang and Peng 26 ). Moreover, ZEA is an activator of human pregnane X receptor, a transcription factor that regulates the expressions of many hepatic drug-metabolising enzymes, including several clinically important cytochrome P450. ZEA concentration–response analyses reveal that the mycotoxin activates human pregnane X receptor with an EC50 value of approximately 1·5 μm ( Reference Ding, Lichti and Staudinger 27 ).
Pigs are generally considered to be the most sensitive animal species to ZEA and its metabolites( Reference Luongo, De Luna and Russo 15 , 28 ). ZEA intoxication is associated with decreased fertility, reduced litter size, change in the weight of adrenal, thyroid and pituitary glands in offspring and change in the serum levels of progesterone and oestradiol( 28 ). Recently, ZEA and its derivatives have been demonstrated to have divergent effects on important parameters of swine innate immunity and have been described as the modulators of the expressions of pro-inflammatory cytokines in peripheral circulating blood cells( Reference Stec, Zmudzki and Rachubik 29 – Reference Marin, Taranu and Burlacu 31 ). Despite these aspects, only a few studies focusing on the hepato- and immunomodulatory effects of ZEA in swine have been reported, especially an in-depth analysis of these effects. However, the histopathological results provide evidence of liver dysfunction such as significant reduction in the levels of total superoxide dismutase and of glutathione peroxidase activity and increase in the levels of malondialdehyde( Reference Jiang, Yang and Yang 21 ). To our knowledge, there are no studies on the effects of dietary ZEA corroborated with the mode of action of ZEA in the liver of swine. For many contaminants of the agro-food chain (ZEA being among them), there are no norms of tolerance (only recommendations) issued by the European Commission, and recently the European Food Safety Authority( 32 ) has recommended that in-depth studies be conducted to determine that not just the readily visible alterations in performance, but also the deeper changes at the cellular and molecular levels are taken into account when determining the doses accepted as maximal limits; this is so, because they may have long-term consequences on animal health and economic repercussions such as the failure of some treatments or vaccinations.
Using in vivo approaches and new molecular biology techniques (real-time PCR), the aim of the present study was to obtain a better insight into the changes produced by this toxin in the expressions of several markers responsible for important defence processes (inflammation and T-helper (Th)1/Th2 pathway regulation) in the liver as the key organ in immune homeostasis and in the detoxification of food contaminants, pharmaceutical substances and xenobiotics. The possible mechanism of ZEA action is suggested.
Materials and methods
Animals and treatments
A total of ten TOPIGS-40 cross-bred weanling piglets (n 5 per group) with an initial average body weight of 9·5 (sem 0·6) kg and age of 35 d were studied for 18 d. The piglets that were individually identified by ear tags were divided into two groups (n 5 piglets/group per pen) and housed in pens and were allocated to the following two dietary treatments: (1) control (maize–soyabean meal basal diet only)( Reference Taranu, Marin and Manda 33 ) and (2) ZEA (control diet contaminated with 250 parts per billion (ppb) ZEA). Feed samples were taken at the beginning of the experiment and were analysed for Fusarium mycotoxin and nutrient content. During the experiment, the piglets were given ad libitum access to water and to the assigned diets. At the end of experiment, the piglets were weighed individually and cumulative feed consumption was measured for each pen. In order to study the effect of ZEA treatment on hepatic immune response, the piglets were killed at the end of the trial and liver samples were collected on ice from all the piglets and an aliquot (30–50 g) was stored at − 80°C until analysis. The piglets were observed twice daily and cared for in accordance with the Romanian Law 206/2004 for handling and protection of animals used for experimental purposes. The study protocol was approved by the Ethical Committee of the National Research-Development Institute for Biology and Animal Nutrition, Balotesti, Romania.
Analysis of mycotoxins
The content of ZEA in the feed was analysed by HPLC with fluorescence detection after clean-up with an immunoaffinity column (Inertsil ODS-3 V; GL Sciences, Inc.) at a detection limit of 6 ng/g. The contaminated diet contained 316·0 (sem 30·9) ppb of ZEA, whereas the control diet contained 40·92 (sem 0·15) ppb of ZEA in compound feed, without contamination with other Fusarium toxins. ELISA using a Veratox ELISA kit (Neogen) with detection limits of 100, 200, 10, 1 and 0·5 ppb, respectively, was carried out to detect for the presence of deoxynivalenol (DON), fumonisin B1, T-2/HT-2, ochratoxin A and aflatoxin toxins, and none of them was detected.
Measurement of cytokine production
Samples of frozen liver were weighed, and 500 mg of tissue samples were homogenised in phosphate buffer containing 1 % IGEPAL, 0·5 % sodium deoxycholate, 0·1 % SDS and complete (EDTA-free) protease inhibitor cocktail tablets. The homogenates were kept on ice for 30 min and then centrifuged at 10 000 rpm at 4°C for 10 min. The supernatants were analysed for protein content using a commercial kit (Pierce® BCA Protein Assay Kit; Thermo Fisher Scientific), and the concentrations of cytokines in the supernatants were determined by ELISA, using commercially available kits (R&D Systems), according to the manufacturer's instructions( Reference Marin, Taranu and Burlacu 30 ). Optical densities were measured on an ELISA reader (Tecan) at a wavelength of 450 nm. Dilutions of recombinant swine IL-1β, IL-8, TNF-α, IL-4, IL-6, IL-10 and interferon-γ (IFN-γ) were used as standards, and data were analysed against the linear portion of the generated standard curve. Results are expressed as pg cytokine/ml supernatant.
Measurement of matrix metalloproteinase-2 and matrix metalloproteinase-9 gelatinase activity
Frozen liver samples of 50 mg were weighed and homogenised for 30 min on ice in a lysis buffer (50 mm-Tris, pH 7·5, 1 % Triton X-100, 250 mm-NaCl, 10 % glycerol and complete EDTA-free protease inhibitor cocktail tablets). The homogenates were centrifuged at 1200 rpm for 10 min at 4°C, and the supernatants were re-centrifuged at 15 000 rpm for 15 min at 4°C. Finally, the resultant supernatants were analysed for total protein content using a commercial kit, and the aliquots were frozen at − 80°C until processing. The determination of gelatinase activity of matrix metalloproteinase (MMP)-2 and MMP-9 in the liver and plasma samples was done using zymography, realised by SDS–PAGE in the presence of 0·1 % gelatin( Reference Grenier, Bracarense and Schwartz 34 ). After electrophoresis, the proteins were renatured by incubating the gel in 2·5 % Triton X-100 for 30 min. The activity of gelatinase was developed by incubating the gel for 18 h at 37°C in an enzymatic substrate containing 50 mm-Tris, pH 7·4, with 5 mm-CaCl2 and 0·2 mm-NaCl2. The lytic activity of gelatinase was visualised, after staining the gel with Coomassie Brilliant Blue, as a clear lane on a blue background. The gelatin zymograms were scanned and matrix metalloproteinase activities were quantified using the Total Lab Quant software (TotalLab Limited). All densitometry results are expressed as arbitrary units.
Immunoblot assays
Samples of frozen liver were weighed, and 500 mg of tissue samples were homogenised in phosphate buffer containing 1 % IGEPAL, 0·5 % sodium deoxycholate, 0·1 % SDS and complete (EDTA-free) protease inhibitor cocktail tablets. The homogenates were kept on ice for 30 min and then centrifuged at 10 000 rpm at 4°C for 10 min. The resultant supernatants were analysed for total protein content using a commercial kit (Pierce® BCA Protein Assay Kit; Thermo Fisher Scientific), and the aliquots were frozen at − 80°C until processing. Immunoblot assays were used to analyse the expression of phospho-p38 mitogen-activated protein kinases (MAPK) in the lysed liver tissue samples. Later, 25 μg of total proteins were separated on 10 % SDS–PAGE and transferred onto nitrocellulose membranes. The membranes were blocked with Tris-buffered saline (pH 7·5) and 5 % bovine serum albumin and incubated with primary antibodies for 2 h at room temperature. Rabbit anti-phospho-p38 MAPK (Thr180/Tyr182) antibodies (diluted 1:200) were obtained from Cell Signaling Technology. Rabbit anti-β-actin antibodies (Cell Signaling Technology), diluted 1:500, were used as the control. The membranes were then washed and incubated for 1 h with secondary antibodies, goat anti-rabbit IgG conjugated with horseradish peroxidase, diluted 1:2000 (Cell Signaling Technology). Immunoreactivity was detected using the SuperSignal* West Pico Chemiluminescent Substrate developing system (Thermo Fisher Scientific). Immunoblotting images were scanned, and the level of proteins was evaluated using the Total Lab Quant software (TotalLab Limited). The results are expressed as the ratio of the phosphorylation level of p38 MAPK to the expression level of β-actin.
Extraction of total RNA
Frozen liver tissue samples of 100 mg were disrupted and homogenised in RTL buffer (QIAGEN GmbH) using an Ultra-Turrax homogeniser (IKA®-Werke GmbH & Co. KG). Total RNA was extracted using a Qiagen RNeasy midi kit (QIAGEN GmbH), according to the manufacturer's recommendations. After extraction, RNA was treated with a ribonuclease inhibitor (RNasin® Plus RNase Inhibitor; Promega Corporation), and the quantity and quality of the extracted total RNA were measured on a Nanodrop ND-1000 spectrophotometer (Thermo Fisher Scientific). The integrity of RNA was verified by agarose gel electrophoresis.
Complementary DNA synthesis
Total RNA from each sample was used to generate complementary DNA using the M-MuLV Reverse Transcriptase kit (Fermentas; Thermo Fisher Scientific) according to the manufacturer's protocol. Briefly, 1 μg of total RNA was used as the starting material, to which 0·5 μg of oligo-(deoxythymine) was added. RNA and oligo-(deoxythymine) were mixed gently and then centrifuged and incubated at 65°C for 5 min, chilled on ice, centrifuged and again placed on ice. The following components were added later: 4 μl of 5 × reaction buffer; 2 μl of deoxyribonucleotide triphosphate mix (1 mm of each deoxyribonucleotide triphosphate); 2 μl of Moloney murine leukaemia virus (MMuLV) RT (40 U). The samples were incubated at 42°C for 60 min, and the reaction mixtures were inactivated at 70°C for 10 min.
Quantitative real-time PCR
Fluorescent real-time PCR was used to evaluate the expressions of pro-inflammatory (TNF-α
IL-1β, IL-8 and IFN-γ) and anti-inflammatory (IL-4, IL-6 and IL-10) cytokines, MMP (MMP-2 and MMP-9), tissue inhibitors of matrix metalloproteinases (TIMP-1 and TIMP-2), PPARγ and MAPK/NF-κB1 genes. The reaction mixtures were set up at a total volume of 20 μl using 5 μl of complementary DNA (diluted 1:10), 12·5 μl Maxima SYBR Green/Fluorescein qPCR Master Mix 2X (Fermentas; Thermo Fisher Scientific) and 0·3 μm of each gene-specific primer (Table 1), and the reactions were carried out in the Rotor-Gene-Q (QIAGEN GmbH) machine. The cycling conditions were as follows: uracil-DNA glycosylase (UDG) pre-treatment at 50°C for 2 min; initial denaturation step at 95°C for 15 s, followed by forty cycles of 95°C for 15 s, 60°C for 15 s and 72°C for 15 s with a single fluorescence measurement; a final elongation step at 72°C for 10 min. The specificity of the PCR products was confirmed by analysis of the dissociation curve. The melting curve programme consisted of temperatures between 60 and 95°C with a heating rate of 0·1°C/s and a continuous fluorescence measurement. All samples were measured in triplicate. The relative product levels were quantified using the
$$2^{( - \Delta \Delta C _{T})} $$
method(
Reference Pistol, Matache and Calugaru
46
). The expression levels of two endogenous control genes, cyclophilin A and β2-microglobulin, were used for data normalisation.
Table 1 Nucleotide sequences of primers used in real-time PCR

IFN-γ, interferon-γ; MMP, matrix metalloproteinases; TIMP, tissue inhibitors of matrix metalloproteinases; TAK1, mitogen-activated protein kinase kinase kinase 7; p38α, mitogen-activated protein kinase 14; JNK1, mitogen-activated protein kinase 8; JNK2, mitogen-activated protein kinase 9.
Statistical analysis
All data are expressed as means with their standard errors. ANOVA and t test analysis were carried out to investigate the statistical differences between the groups for all the parameters analysed. Further differences between the means were determined using the least square difference Fisher procedure. A simple regression (y= ax 2+b) procedure (StatView software; SAS Institute, Inc.) was used to establish correlations between the gene expressions of signalling (PPARγ, NF-κB1 and MAPK) and inflammation-related (IL-1β, IL-8, TNF-α, IL-4, IL-6 and IL-10) molecules in the liver of piglets that were given dietary mycotoxin. Briefly, correlations between nuclear receptors (PPARγ and NF-κB1), MAPK (mitogen-activated protein kinase kinase kinase 7 (TAK1)/mitogen-activated protein kinase 14 (p38α)/mitogen-activated protein kinase 8 (JNK1)/mitogen-activated protein kinase 9 (JNK2)) as independent variables (y) and inflammation-related markers as dependent variables (x) were established. Values of P< 0·05 were considered significant.
Results
Effect of zearalenone on animal performance
Piglets fed the control or ZEA-contaminated diet appeared to be clinically normal during the whole experimental period; at the end of the trial, average daily gain (0·367 v. 0·344 kg/piglet per d, P= 0·58), daily feed intake (0·681 v. 0·716 kg/piglet per d, P= 0·41) or feed:gain ratio (1·87 v. 2·17, P= 0·25) was not influenced by the dietary treatments.
Dietary zearalenone modulates the concentrations and gene expressions of pro- and anti-inflammatory cytokines in swine liver
First, we examined the in vivo effect of ZEA on the pro-inflammatory cytokine profile as a marker of inflammation. The present results clearly showed a strong decrease in the protein concentrations of TNF-α, IL-8, IL-6, IL-1β and IFN-γ in the liver of piglets treated with ZEA when compared with the control piglets (TNF-α: − 52·76 %; IL-8: − 49·79 %; IL-6: − 58·05 %; IL-1β: − 49·73 %; IFN-γ: − 54·81 %, respectively) (Fig. 1). The alteration in the levels of pro-inflammatory markers caused by ZEA was more profound, also being observed at the mRNA expression level (TNF-α: − 83·44 %; IL-8: − 63·68 %; IL-6: − 92·68 %; IL-1β: − 85·06 %; IFN-γ: − 88·85 %, respectively) (Fig. 2). The protein concentrations and expressions of cytokines known to be involved in anti-inflammatory reactions, IL-10 and IL-4, were then analysed in order to get a comprehensive picture of the effects of ZEA on swine cytokine response with possible repercussion on Th1/Th2 cytokine balance. The concentrations of both IL-10 and IL-4 in the liver decreased significantly by − 50·69 and − 58·26 %, respectively (Table 2). The expression of IL-4 mRNA was also significantly reduced in the liver of piglets treated with dietary ZEA ( − 89·95 %, P< 0·0001), while that of IL-10 gene in the liver of piglets fed the ZEA-contaminated diet was unmodified in comparison with the control piglets ( − 0·87 %) (Table 3).
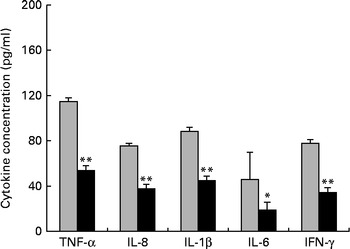
Fig. 1 Effects of the zearalenone (ZEA)-contaminated diet on the synthesis of cytokines, TNF-α, IL-8, IL-1β, IL-6 and interferon-γ (IFN-γ), in the liver of piglets. The supernatants were analysed for total protein content, using a commercial kit. The concentrations of TNF-α, IL-8, IL-1β, IL-6 and IFN-γ in the supernatants were determined by ELISA using R&D Systems kits (R&D Systems), according to the manufacturer's instructions. Optical densities were measured on an ELISA reader (Tecan) at a wavelength of 450 nm. Results are expressed as pg/ml, and they were normalised to total protein concentration. Values are means (n 5), with their standard errors represented by vertical bars. Mean value of the ZEA-contaminated group (■) was significantly different from that of the control group (): * P< 0·05, ** P< 0·001 (one-way ANOVA test).
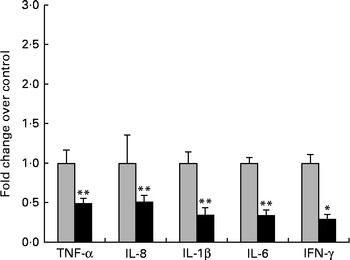
Fig. 2 Influence of dietary zearalenone (ZEA) on the expressions of pro-inflammatory cytokine genes and interferon-γ mRNA in the liver of piglets. Liver samples were analysed for the mRNA expressions of cytokines using quantitative real-time PCR. Results are expressed as fold changes after normalisation of the expression of target cytokine gene to the arithmetic mean of two internally expressed reference genes. Values are means from three independent replicates, with their standard errors represented by vertical bars. Mean value of the ZEA-contaminated group (■) was significantly different from that of the control group (): * P< 0·05, ** P< 0·001 (one-way ANOVA followed by Fisher's test).
Table 2 Effect of in vivo exposure of piglets to zearalenone (ZEA) on the protein levels of anti-inflammatory cytokines in the hepatic tissue (Mean values with their standard errors)

* Mean value was significantly different from that of the control group (P< 0·05; one-way ANOVA followed by Fisher's test).
† Piglets were fed a control diet or a diet contaminated with 316 μg ZEA/kg diet for 18 d. At the end of the experiment, liver samples from all the piglets (n 5) were collected and analysed for the levels of cytokines using ELISA.
Table 3 Effect of in vivo exposure of piglets to zearalenone (ZEA) on the gene expressions of anti-inflammatory cytokines in the hepatic tissue (Mean values with their standard errors)

* Mean value was significantly different from that of the control group (P< 0·05; one-way ANOVA followed by Fisher's test).
† Piglets were fed a control diet or a diet contaminated with 316 μg ZEA/kg diet for 18 d. At the end of the experiment, liver samples from all the piglets (n 5) were collected and analysed for the mRNA expressions of cytokines using quantitative real-time PCR.
‡ Results are expressed as fold changes after normalisation of the expression of target cytokine gene to the arithmetic mean of two internally expressed reference genes.
Dietary zearalenone modulates the activity of matrix metalloproteinases and their inhibitors in swine liver
The next step in the trial was to determine the effect of ZEA on MMP as important molecules involved in inflammatory processes that are closely associated with the cytokine network. Gelatin zymography technique was used to investigate MMP activity in the liver and plasma samples. Densitometric analysis of the scanned zymograms (Fig. 3) showed that the activities of both MMP-2 and MMP-9 were corroborated with the gene expression analysis, being decreased in the liver of piglets fed the ZEA-contaminated diet ( − 60·30 % for MMP-2 and − 34·10 % for MMP-9) in comparison with the control group. The same profile of gelatinase activity was obtained for the plasma samples ( − 90·74 % for MMP-2 and − 89·32 % for MMP-9) (Fig. 3). The concentration of 316 μg ZEA/kg diet also led to a significantly marked decrease in the expressions of both hepatic MMP genes (MMP-2: − 81·55 %, P< 0·05; MMP-9: − 96·37 %, P< 0·001) (Fig. 4).
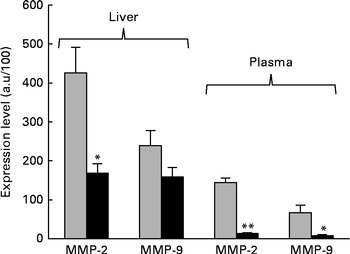
Fig. 3 Effects of dietary zearalenone (ZEA) on the activity of matrix metalloproteinases (MMP) in the liver tissue and plasma samples. Liver extracts were resolved on SDS–PAGE zymography using 0·1 % gelatin. Results are expressed as arbitrary units (a.u.). Values are means, with their standard errors represented by vertical bars. Mean value of the ZEA-contaminated group (■) was significantly different from that of the control group (): * P< 0·05, ** P< 0·001 (one-way ANOVA test).
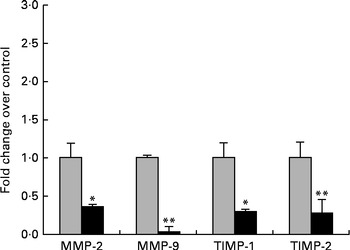
Fig. 4 Effects of zearalenone (ZEA) treatment on the gene expressions of matrix metalloproteinases (MMP)/tissue inhibitors of matrix metalloproteinases (TIMP) in the liver tissue samples. Liver samples were analysed for the expressions of MMP/TIMP mRNA using quantitative real-time PCR. Results are expressed as fold changes after normalisation of the expression of target cytokine gene to the arithmetic mean of two internally expressed reference genes. Values are the means from three independent replicates, with their standard errors represented by vertical bars. Mean value of the ZEA-contaminated group (■) was significantly different from that of the control group (): * P< 0·05, ** P< 0·001 (one-way ANOVA followed by Fisher's test).
Knowing that TIMP are the most efficient natural inhibitors of MMP activity and secretion, we evaluated the gene expressions of two members of the TIMP family, TIMP-1 and TIMP-2, using quantitative real-time PCR. We observed that the expressions of these genes at the hepatic level were significantly decreased in comparison with the control samples (TIMP-1: − 80·48 %, P< 0·05; TIMP-2: − 79·34 %, P< 0·05; Fig. 4).
Effects of zearalenone toxin on the gene expression of PPARγ in swine liver
In order to investigate the possible involvement of nuclear receptor PPARγ as a signal molecule in inflammatory processes, we analysed the expression of PPARγ gene in swine liver using real-time PCR. The present experiment demonstrated that after dietary exposure to ZEA toxin, the expression of PPARγ gene was not modified in the liver of the intoxicated piglets (a decrease of − 23·33 % in comparison with the control group) (Table 4).
Table 4 Effect of in vivo exposure of piglets to zearalenone (ZEA) on the gene expressions of nuclear receptors and mitogen-activated protein kinases (MAPK) in the hepatic tissue (Mean values with their standard errors)

TAK1, mitogen-activated protein kinase kinase kinase 7; p38α, mitogen-activated protein kinase 14; JNK1, mitogen-activated protein kinase 8; JNK2, mitogen-activated protein kinase 9.
* Mean value was significantly different from that of the control group (P< 0·05; one-way ANOVA followed by Fisher's test).
† Piglets were fed a control diet or a diet contaminated with 316 μg of ZEA/kg diet for 18 d. At the end of the experiment, liver samples from all the piglets (n 5) were collected and analysed for the mRNA expressions of cytokines using quantitative real-time PCR.
‡ Results are expressed as fold changes after normalisation of the expression of target cytokine gene to the arithmetic mean of two internally expressed reference genes.
Effects of dietary zearalenone on the gene expressions of transcription signalling molecules mitogen-activated protein kinases (mitogen-activated protein kinase kinase kinase 7/mitogen-activated protein kinase 14/mitogen-activated protein kinase 8/mitogen-activated protein kinase 9) and NF-κB1 in swine liver
To further investigate the involvement of MAPK and/or NF-κB signalling pathways in ZEA-induced abnormalities in the liver, we evaluated the expressions of TAK1, p38α, JNK1/JNK2 MAPK and NF-κB1 genes using real-time PCR. The present results showed that the treatment with 316 ppb dietary ZEA significantly down-regulated the expression of p38α gene ( − 74·60 %, P< 0·0001) and that of its activator TAK1 ( − 98·48 %, P< 0·0001), while the expressions of JNK1 and JNK2 mRNA were not modified in the liver samples of piglets treated with dietary ZEA (6·24 and − 0·88 %, respectively) (Table 4). The analysis of the NF-κB1 signalling pathway showed that the expression of NF-κB1 gene in the liver was significantly (P< 0·0001) reduced ( − 77·33 %) (Table 4).
The immunoblot analysis showed that the phosphorylation level of p38 MAPK was significantly reduced ( − 36·37 %, P< 0·001) in the liver samples collected from piglets treated with dietary ZEA (Fig. 5).
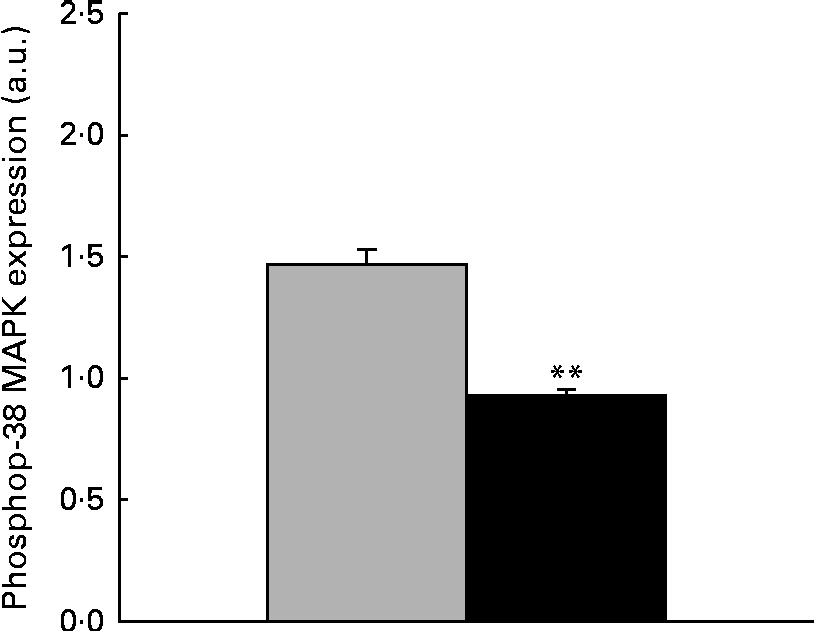
Fig. 5 Influence of dietary zearalenone (ZEA) on the p38 mitogen-activated protein kinase (MAPK) phosphorylation level in the liver of piglets. p38 MAPK phosphorylation level at Thr180/Tyr182 was determined using Western blot analysis, and it is expressed as the ratio of phospho-p38 MAPK to β-actin band intensities. Values are means for each group of piglets, with their standard errors represented by vertical bars. ** Mean value of the ZEA-contaminated group (■) was significantly different from that of the control group () (P< 0·001; one-way ANOVA test). a.u., Arbitrary units.
Correlations between the gene expressions of the nuclear receptor NF-κB1, inflammation-related markers (cytokines/matrix metalloproteinases) and signalling mitogen-activated protein kinases (mitogen-activated protein kinase kinase kinase 7/mitogen-activated protein kinase 14/mitogen-activated protein kinase 8/mitogen-activated protein kinase 9) in the hepatic tissue of piglets fed the zearalenone-contaminated diet
Mathematical correlations were established in order to better understand the relationships between the expressions of signalling molecules and inflammation genes in the liver samples derived from piglets fed dietary ZEA. The linear regression analysis showed no correlation between the expressions of PPARγ and inflammatory-related genes (data not shown) induced by the ZEA treatment.
When a linear regression analysis was carried out using the expression of NF-κB1 gene as the dependent variable, we obtained good correlations between the expressions of NF-κB1 and pro-inflammatory cytokine genes (TNF-α: R 2 0·469; IL-1β: R 2 0·655) and also between those of NF-κB1 gene and genes coding for TIMP-1 (R 2 0·697) and TIMP-2, respectively (R 2 0·582). A significantly high correlation was established between the expressions of NF-κB1 and IFN-γ genes (R 2 0·912, P< 0·01; Table 5). Good correlations were also found between the expressions of IL-10, IL-4 and NF-κB1 mRNA (R 2 0·454 for IL-10; R 2 0·662 for IL-4). As expected, significant correlations were found between the expressions of NF-κB1 and p38α/JNK2 genes (R 2 0·951, P< 0·01 for p38α; R 2 0·923, P< 0·001 for JNK2) (Table 5).
Table 5 Correlations between the gene expressions of nuclear receptor (NF-κB1), inflammation-related markers (cytokines/matrix metalloproteinases (MMP)) and signalling mitogen-activated protein kinases (MAPK) (mitogen-activated protein kinase kinase kinase 7 (TAK1)/mitogen-activated protein kinase 14 (p38α)/mitogen-activated protein kinase 8 (JNK1)/mitogen-activated protein kinase 9 (JNK2)) in the hepatic tissue of pigs fed the zearalenone-contaminated diet

IFN-γ, interferon-γ; TIMP, tissue inhibitors of matrix metalloproteinases.
* Values were significantly different (P< 0·05).
† A simple regression (y= ax 2+b) procedure was used to establish relationships between the gene expressions of NF-κB1, inflammation-related markers and signalling MAPK. r 2= coefficient of determination.
Correlations between the gene expressions of mitogen-activated protein kinases (mitogen-activated protein kinase kinase kinase 7/mitogen-activated protein kinase 14/mitogen-activated protein kinase 8/mitogen-activated protein kinase 9), inflammation-related markers (cytokines/matrix metalloproteinases) and nuclear receptors (PPARγ) in the hepatic tissue of piglets fed the zearalenone-contaminated diet
A linear regression analysis was used to evaluate the possible correlations between the expressions of MAPK (TAK1/p38α/JNK), pro/anti-inflammatory cytokine and MMP/TIMP genes in the hepatic tissue of ZEA-treated piglets. The results showed high correlations between the gene expressions of MAPK and Th1/Th2 cytokines and low correlations between the gene expressions of IL-8 and IL-6 cytokines, which are produced by different cell types (Table 6). High correlations were observed between the expressions of TAK1 and TNF-α genes (R 2 0·846, P< 0·05) and also between those of TAK1 and the other cytokine genes (IL-1β: R 2 0·614; IFN-γ: R 2 0·446; IL-4: R 2 0·620; IL-6: R 2 0·673) (Table 6). Similarly, high linear correlations were found between the expressions of p38α and IL-1β (R 2 0·634), IL-10 (R 2 0·644), IL-4 (R 2 0·822) and TIMP-1 (R 2 0·679) genes, with a significant one for the expression of IFN-γ gene (R 2 0·766, P= 0·05) and between the expressions of JNK2 and TNF-α (R 2 0·530), IL-1β (R 2 0·584) and TIMP-2 (R 2 0·799) genes as well as between those of JNK2 and IFN-γ genes (R 2 0·986, P< 0·001) (Table 6). A significant linear correlation was also established between the expressions of p38α and JNK2 genes (R 2 0·767, P= 0·05) (Table 6). No correlations between the expressions of JNK1 and inflammation-related genes were found (data not shown).
Table 6 Correlations between the gene expressions of mitogen-activated protein kinases (MAPK), inflammation-related markers (cytokines/matrix metalloproteinases (MMP)) and nuclear receptors in the hepatic tissue of pigs fed the zearalenone-contaminated diet

TIMP, tissue inhibitors of matrix metalloproteinases; IFN-γ, interferon-γ; TAK1, mitogen-activated protein kinase kinase kinase 7; p38α, mitogen-activated protein kinase 14.
* Values were significantly different (P< 0·05).
† A simple regression (y= ax 2+b) procedure was used to establish relationships between the gene expressions of MAPK (TAK1/p38α/JNK2), inflammation-related markers (cytokines/MMP) and nuclear receptors (PPARγ/NF-κB). r 2= coefficient of determination.
Discussion
Pigs, particularly the gilts, seem to be the most sensitive animal species to ZEA and its metabolites( 32 ). Various studies have indicated that the female pigs are particularly sensitive to the oestrogenic effects of ZEA( 32 ), but there are only a few studies that have investigated the effect of ZEA on swine immune response( 28 – Reference Taranu, Marin and Manda 33 ) and even fewer on the effect of ZEA on the immune response in the liver. It is known that after an oral exposure, ZEA is metabolised in various tissues, particularly in the liver( Reference Dänicke, Swiech and Buraczewska 47 ). Situated in a unique position between the gastrointestinal tract and the systemic venous system, the liver plays a key role in immune system homeostasis( Reference Lapierre and Alvarez 48 ) being constantly exposed to food antigens (e.g. toxins), bacterial products and potential pathogens through the mesenteric circulation. Fetal liver is also a haematopoietic organ along with the adult spleen( Reference Pahl, Krauss and Schulze-Osthoff 49 ). That is why in the present study weaned piglets were exposed to 316 ppb ZEA through the diet for 18 d and the significant changes produced by ZEA in the levels of several markers involved in the liver defence processes were investigated. The consumption of the experimentally contaminated diet did not alter animal performance.
Dietary zearalenone modulates the gene expressions and protein concentrations of inflammation-related markers (cytokines/matrix metalloproteinases) in swine liver
The consumption of the ZEA-contaminated diet dramatically reduced the concentrations of several pro-inflammatory cytokines (TNF-α, IL-8, IL-1β, IL-6 and IFN-γ) as well as their gene expressions in the liver of piglets fed the ZEA-contaminated diet (Figs. 1 and 2). Very importantly, ZEA also decreased the expressions of IFN-γ (at the gene and protein levels) and Th1 master cytokines, which play a major role in the host defence against intracellular infections. The ingestion of the ZEA-contaminated diet also led to the down-regulation of the gene expressions and protein levels of the characteristic Th2 cytokine (IL-4) involved in humoral immune response. The dietary ZEA seems to have had no effect on the gene expression of the anti-inflammatory cytokine IL-10, but it altered the protein concentration of this cytokine in the hepatic tissue. These results are in agreement with the results of the study of Wang et al. ( 16 ), who found the alteration in the expressions of cytokines (IL-2, IL-6 and IFN-γ) in chicken splenocytes to be associated in vitro with increasing concentrations of ZEA. It has been reported that the genotoxic effect of ZEA is due to the DNA fragmentation brought about by it, which leads to the inhibition of protein synthesis( Reference Salah-Abbès, Abbès and Haous 50 , Reference Abid-Essefi, Bouaziz and Golli-Bennour 51 ). In other studies, ZEA and its derivatives have also been described as the suppressors( Reference Marin, Taranu and Burlacu 31 , Reference Salah-Abbès, Abbès and Houas 52 ) of the expressions of pro-inflammatory cytokines and as inducers of DNA fragmentation( Reference Gazzah, El Golli Bennour and Bouaziz 53 ). DNA fragmentation was among the chronological succession of some events that characterised the toxicity of ZEA in human hepatocarcinoma cells( Reference Gazzah, El Golli Bennour and Bouaziz 53 ). Besides ZEA, Fusarium sp. produces toxins such as DON or fumonisin B1, which are also immunomodulators, being able to decrease or increase the production of cytokines in the liver and other tissues. For example, in vivo ingestion of 2·8 μmol fumonisin B1/kg body weight per d decreased the expressions of IL-6, IL-10, IL-18 and IFN-γ in the liver of piglets, while the expressions of IL-1β and IL-8 were increased( Reference Royaee, Husmann and Dawson 36 ). DON induced modest expressions of TNF-α, IL-6 and IL-1β in the liver relative to the spleen( Reference Azcona-Olivera, Ouyang and Murtha 54 , Reference Pestka and Amuzie 55 ), but increased the expressions of Th2-mediated cytokines IL-4 and IL-6 and suppressed those of IFN-γ and Th1 cytokine in Peyer's patches of mice infected with reovirus exposed previously to DON contamination( Reference Li, Cuff and Pestka 56 ). This divergent effect may be due to the differences in their toxicities and animal models.
In the present study, the ZEA-contaminated diet exerted a significant inhibitory effect on the gene expressions of other hepatic inflammatory mediators, MMP and TIMP (Fig. 4). The gelatin zymography analysis also showed a decrease in MMP-2 and MMP-9 gelatinolytic activities in both the liver and plasma samples obtained from piglets fed the ZEA-contaminated diet. Only a few studies have analysed the effect of mycotoxins on MMP activity and/or secretion, and to our knowledge, this is the first study to investigate the effect of ZEA on metalloproteinases and their inhibitors in pigs. But other studies have shown that in mice, T-2 toxin induced alterations in blood–brain barrier permeability, mediated partially through the activation of MMP-9( Reference Ravindran, Agrawal and Gupta 57 ), while in humans, rubratoxin B exhibited inhibitory activities against MMP-2 and MMP-9 in fibrosarcoma HT 1080 cells( Reference Wang, Zhang and Wang 58 ). By contrast, Gagliano et al. ( Reference Gagliano, Donne and Torri 59 ) demonstrated that chronic treatment with ochratoxin A did not affect the protein levels of MMP-2 and MMP-9 in rat liver.
Dietary zearalenone alters the gene expressions and protein concentrations of transcription signalling molecules in the hepatic tissue of piglets fed the zearalenone-contaminated diet
We further investigated whether the alterations induced by ZEA in the liver implied nuclear receptors (PPARγ and NF-κB1) and MAPK (TAK1, p38α, JNK1 and JNK2) to be sentinel effectors in the signal transduction pathways of inflammation. PPARγ is a member of the nuclear receptor superfamily PPAR involved in the regulation of the immune response, particularly in inflammation control; the majority of studies support an anti-inflammatory role for PPARγ( Reference Zhan, Huang and Luo 60 – Reference Cuzzocrea, Wayman and Mazzon 62 ) in terms of cytokine regulation. In the present study, piglets fed the diet contaminated with 316 ppb of ZEA exhibited only a slight increase in the expression of PPARγ gene and, despite the significant decrease in the expressions of pro-inflammatory cytokine genes, no correlation between the expressions of PPARγ and pro-inflammatory cytokine genes was found in the liver of piglets fed the ZEA-contaminated diet. Similarly, Ding et al. ( Reference Ding, Lichti and Staudinger 27 ) found that PPARγ was not among the nuclear receptors activated in vitro by 10 μm of ZEA in CV-1 cells at the mRNA level. In a docking study, Prouillac et al. ( Reference Prouillac, Koraichi and Videmann 63 ) suggested that ZEA, which has smaller size and lower flexibility, could not establish high interactions with this receptor because of its high flexibility and of the particular conformation of its ligand-binding site (much larger bended ligand-binding pocket than other nuclear receptors). Conversely, PPARγ could be activated by other mycotoxins and feed compounds. Moon et al. ( Reference Moon, Yang and Park 64 ) proved that DON induced the production of IL-8 through the activation of PPARγ and MAPK (extracellular signal-regulated protein kinases 1/2 and early-growth response protein 1), while Zhan et al. ( Reference Zhan, Huang and Luo 60 ) demonstrated that n-3 PUFA from the linseed diet lead to a significant increase in the expression of PPARγ gene with significant negative linear correlations between the expressions of PPARγ and pro-inflammatory cytokine genes in the muscle and spleen of fattening pigs.
The study of Ding et al. ( Reference Ding, Lichti and Staudinger 27 ) showed that ZEA binds to hepatic pregnane X receptor and serves as a direct ligand for the NF-κB1 receptor, other important transcription factors involved in the pro-inflammatory signal transduction pathway. NF-κB has been reported to induce the transcription of various cytokine genes such as IL-1, IL-2 and IL-6 ( Reference Pahl, Krauss and Schulze-Osthoff 49 ). It has been shown in mouse hepatocytes that the activation of pregnane X receptor decreases the transcriptional activity of NF-κB( Reference Zhou, Tabb and Nelson 65 ). In the present study, we showed that dietary consumption of 316 ppb ZEA caused a significant decrease in the expression of NF-κB1 gene. This was associated with a significant decrease in the expressions of pro-inflammatory cytokines and a high correlation between them, which was significant for the expression of IFN-γ. A significant reduction in the translocation of NF-κB has also been demonstrated by treatments with other mycotoxins, gliotoxins in lipopolysaccharide-stimulated dendritic cells (DC)( Reference Schütze, Lehmann and Bönisch 66 , Reference Wilson, Leone and Anderson 67 ) and citrinin in MES-13 cells, followed by the suppression of the expressions of IFN-γ, NO and inducible NO synthase( Reference Liu, Chi and Hsiao 68 ). No effect on the expression of NF-κB was observed on treatment with patulin in lipopolysaccharide-stimulated DC( Reference Schütze, Lehmann and Bönisch 66 ).
The MAPK family of serine/threonine kinases is a key component of the signalling pathways that regulate a variety of intracellular processes, including growth, differentiation and the response to cytokines and stress( Reference Johnson and Lapadat 69 ). Thus, p38α MAPK plays a role in the induction of cell cycle and cell development and in the inducible expressions of many pro-inflammatory cytokine genes, whereas JNK proteins are involved in cytokine production, inflammatory responses, apoptosis, actin organisation and metabolism( Reference Erkel, Rether and Anke 70 , Reference Raman, Chen and Cobb 71 ). TAK1 is also a key molecule in the pro-inflammatory signalling pathways, being responsible for the phosphorylation and activation of several kinases, which in turn activate both MAPK (JNK and p38α) and NF-κB (IκB kinase)( Reference Ninomiya-Tsuji, Kajino and Ono 72 , Reference Ninomiya-Tsuji, Kishimoto and Hiyama 73 ). The intake of 316 ppb ZEA diet decreased the expressions of p38α MAPK and JNK1 genes as well as that of their activator TAK1 in the liver of piglets from the experimental group, while that of JNK2 gene remained unaffected by ZEA contamination. Similar to that found for the expression of NF-κB1 gene, significantly high correlations were found between the expressions of p38α MAPK, JNK2 and IFN-γ genes. A low correlation was found between the expressions of TAK1 and p38α MAPK genes, which may be due to the intercalation of other MAPK between them. It seems that the effects of ZEA on MAPK depend on the type of the cells. The study of Yu et al. ( Reference Yu, Zheng and Son 74 ) showed that ZEA induced cytotoxicity in RAW264.7 cells through the activation of p53 and MAPK (JNK/p38) signalling pathways. In contrast, the phosphorylation state of MAPK has been reported to be strongly reduced in pig endometrial cells by β-ZEA, a ZEA derivative( Reference Wollenhaupt, Jonas and Tiemann 75 ), similar to that observed in the present study, in which ZEA also significantly reduced the protein expression of phosphorylated p38 in porcine hepatic cells. Other mycotoxins, e.g. DON, have been found to induce the activation of MAPK in many other cell types, macrophages( Reference Pestka and Zhou 76 ) and Jurkat cells( Reference Pestka, Uzarski and Islam 77 ) and, recently, Pinton et al. ( Reference Pinton, Tsybulskyy and Lucioli 78 ) have demonstrated that DON and acetylated DON modulated differently the expressions of MAPK in pig enterocytes with respect to their concentrations and may thus influence their inflammatory and anti-inflammatory responses. These opposite results suggest that structurally different mycotoxins employ different mechanisms while exerting their effects on molecular signalling pathways.
In summary, the results presented herein suggest that ZEA is not only a powerful oestrogen endocrine disruptor, but also a hepatoimmunotoxic Fusarium toxin in pigs. By inhibiting the gene expressions and protein concentrations of MAPK (TAK1/JNK/p38) and NF-κB, the key effectors of signal transduction pathways, this mycotoxin caused severe hepatic immunosuppression (significant reduction in the expressions and protein concentrations of several markers of pro- and anti-inflammatory processes), which might have important consequences during an infection process. The present results suggest that the toxic action of ZEA begins in the upstream of the signal transduction pathway of MAPK by the inhibition of the expression of TAK1 gene, an activator of MAPK and NF-κB. This hypothesis is also supported by the study of Ninomiya-Tsuji et al. ( Reference Ninomiya-Tsuji, Kajino and Ono 72 ), who showed that 5Z-7-oxozeaenol, another natural fungal metabolite, induced a high inhibition of TAK1 kinase. Exposure of cells to 5Z-7-oxozeaenol has been reported to inhibit the catalytic activity of TAK1 and consequently p38, JNK, IκB kinase and NF-κB by interacting with the ATP-binding site of TAK1. In the other possible scenario, the repression of NF-κB could result from the oestrogenic activity of ZEA. It is known that autocrine hormones such as PG, e.g. 15d-PGJ2 (prostanoid 15-deoxy-PGJ2), can repress NF-κB activity independent of PPARγ by inhibiting IκB kinase and/or by alkylating p50–65 NF-κB heterodimers( Reference Szanto and Nagy 79 ).
Considering that ZEA is a frequent contaminant of animal feed and human food preparations, the present results might have clinical relevance and could contribute to the establishment of the maximal limit of tolerance in feeds for pigs.
Acknowledgements
The authors thank Dr Mihaela Mocanu and Dr Gina Manda from the National Institute of Research and Development in the Pathology Domain and Biomedical Sciences ‘Victor Babes’ from Bucharest for their help with the immunoblot analysis.
The present study was supported by funds from PNII – IDEI 101/2011–2014 and PNII – PARTENERIATE 102/2012–2015, granted by the Romanian Ministry of Research and Technology. The Romanian Ministry of Research and Technology had no role in the design and analysis of the present study or in the writing of this article.
The contribution of each author was as follows: G. C. P. wrote the manuscript, carried out the quantitative real-time PCR analyses and cytokine and Western blot determinations, and analysed the data; M. A. G. and D. E. M. contributed to the progress of the in vivo experiment (sample collection and preparation); M. S. carried out the cytokine ELISA measurement; F. I. R. conducted the HPLC for ZEA analyses in compound feed; I. T. conducted and designed the research experiment and contributed to manuscript preparation.
The authors have no conflicts of interest.