Introduction
Age-related macular degeneration (AMD) is one of the global burdens of eye diseases in the ageing world(Reference Gheorghe, Mahdi and Musat1,Reference Mitchell, Liew and Gopinath2) . One of the main factors contributing to blindness is AMD in the industrialised Western countries among those over the age of 55. It is estimated that approximately 9% of all occurrences of blindness are caused by AMD. In a systematic review, the global prevalence of AMD is expected to rise from 196 million in 2020 to 288 million in 2040 worldwide. The progression rate of the disease is rapidly accelerating, resulting in an important public health problem. The incidence of AMD increases with advancing age, and the development of AMD is influenced by both genetic predisposition and environmental factors(Reference Wong, Su and Li3–Reference Koçyiğit and Nilüfer5). For the dry AMD type, there is currently no pharmaceutical therapy available, while intravitreal anti-vascular endothelial growth factor (anti-VEGF) inhibitors are utilised in the treatment of wet AMD(Reference Hernández-Zimbrón, Zamora-Alvarado and Velez-Montoya6,Reference Nashine7) . The treatment of AMD includes different approaches including drug therapy, quitting smoking, blood pressure regulation, combination therapy, photodynamic therapy, thermal laser photocoagulation, radiation therapy, surgical intervention and nutritional management(Reference Arroyo8).
AMD is a multifaceted disease that can be affected by a wide variety of modifiable and non-modifiable risk factors(Reference García-Layana, Cabrera-López and García-Arumí9). Primary modifiable risk factors for age-related macular degeneration include smoking, having a high body mass index, excessive sunlight exposure, alcohol usage, oxidative changes, a high-fat diet and an inadequate intake of dietary antioxidants. Hypertension, atherosclerosis, a family history of AMD, advanced age, cataract surgery, cardiovascular disease, increased plasma fibrinogen, diabetes and genetics are additional risk factors for AMD. Genome-wide association studies (GWAS) have shown to be by far the most effective method for constructing a genetic risk map related to AMD. Polymorphisms in the complement factor H (CFH), age-related maculopathy susceptibility 2 (ARMS2) and HtrA serine peptidase 1 (HTRA1) genes significantly contribute to the risk of AMD(Reference Stahl10,Reference Flores, Carneiro and Vieira11) .
When it comes to treatment strategies for AMD, there is currently no clinically proven treatment for AMD to be fully effective(Reference van Lookeren Campagne, LeCouter and Yaspan12). This situation increases the importance of strategies to prevent and delay AMD(Reference van Lookeren Campagne, LeCouter and Yaspan12,Reference Bernstein, Li and Vachali13) . As mentioned above, nutrition and some nutrients, as well as non-nutrient compounds, are among the most important modifiable risk factors for AMD(Reference Carneiro and Andrade14). In this context, in a pioneering study in this field on eye diseases known as The Age-Related Eye Disease Study (AREDS), there are some important findings that vitamins such as vitamins C, E and A and zinc reduce the 5-year prognosis of AMD by about 25%(15). These important findings indicated that nutrition has an important role in both the prevention and prognosis of AMD and accelerated research in this area. Again, there is some evidence that some vitamins, phytochemicals and bioactive fatty acids, which are known to have an antioxidant role in reducing the oxidative stress that increases with age, play a synergistic role in the neutralisation of free radicals/prooxidants(Reference Tomada and Andrade16). In addition to this, with the increasing awareness of nutrition and health among consumers in recent years, studies on both the market availability and health effects of natural products, some phytochemical-based nutraceuticals and important gut modulators such as probiotics and prebiotics, have increased significantly. However, the potential roles of these components in AMD seem to have not been entirely discussed. In the present manuscript, the potential roles and mechanisms of action of nutrients, non-nutrient bioactive compounds and some dietary patterns on AMD, which are closely associated with eye health and AMD, are discussed in detail.
Classifications and clinical alterations of AMD
The retina, situated posteriorly inside the eye, serves as the primary location for the processing of visual information(Reference Armento, Ueffing and Clark17). The retina is a divided, multilayered tissue consisting of photoreceptor cells (rods and cones), a network of four types of neurons (bipolar, horizontal, amacrine cells and ganglion), a neurosensory retina consisting of special glial cells, and retinal pigment epithelium (RPE) cells located on Bruch’s membrane. RPE cells have a high concentration of melanin. RPE cells have multiple roles in maintaining the health and function of photoreceptor cells and preventing retinal degeneration. These include absorbing light energy and protecting against photooxidation, as well as facilitating the transport of ions, metabolites and fluid across the blood–retinal barrier. Additionally, RPE cells provide essential nutrients such as glucose, retinol and fatty acids to photoreceptor cells(Reference Walchuk and Suh18,Reference Hadziahmetovic and Malek19) . The macula, which contains the largest density of cone photoreceptors in the eye and provides central visual acuity, is located in the fovea, the central part of the retina. The macula cells, particularly those in the fovea, play a crucial role in visual acuity. Any deterioration in this region might result in the impairment of central vision or total blindness(Reference Grant, Bernstein and Boesze-Battaglia20). AMD is an example of a retinal disease that impacts the macula. Nutrition plays a crucial role in preserving retinal health, with vitamins, minerals, lutein, zeaxanthin and omega-3 fatty acids playing significant roles in various biochemical processes within the eye and the nutritional composition of the diet impacting on the risk and severity of AMD. Furthermore, nutrition affects the gut microbiota, which through processes such as modulation of low-grade inflammation indirectly impacts on AMD.(Reference Rinninella, Mele and Merendino21,Reference Di Carlo and Augustin22) .
Multiple classification methods for AMD exist in the literature and clinical practices. Previous population-based studies have divided AMD into early and late types(Reference Ferris, Wilkinson and Bird23,Reference Klein, Meuer and Myers24) . In clinical applications, the AREDS severity scale and its simplified version were utilised(Reference Ferris, Davis and Clemons25). Regarding the Beckman classification, AMD is not likely to develop if the drusen are less than 63 µm in diameter. Retinal pigmentary abnormalities in the macula and the occurrence of medium-sized drusen (>63 to ≤125 µm) are considered early AMD, which are often asymptomatic(Reference Ferris, Wilkinson and Bird23). The intermediate stages of the illness, which are usually oligosymptomatic, are characterised by a rise in the size, amount and pigmentation of drusen, which may be seen using fundus imaging of the retina. The late AMD is subdivided into two subtypes, but both result in severe vision impairment. Late AMD may cause damage to the retina as either a ‘wet’ type, characterised by unstructured choroidal neovascularisation (CNV), or a ‘dry’ form, characterised by distinct areas of retinal pigmented epithelium (RPE) cell loss, known as geographic atrophy (GA). The neovascular type of late AMD can advance quickly in weeks or months, whereas the atrophic form progresses more slowly in years or decades(Reference Mitchell, Liew and Gopinath2,Reference García-Layana, Cabrera-López and García-Arumí9) . The different classifications of AMD are presented in Table 1.
Table 1. Different classifications of AMD
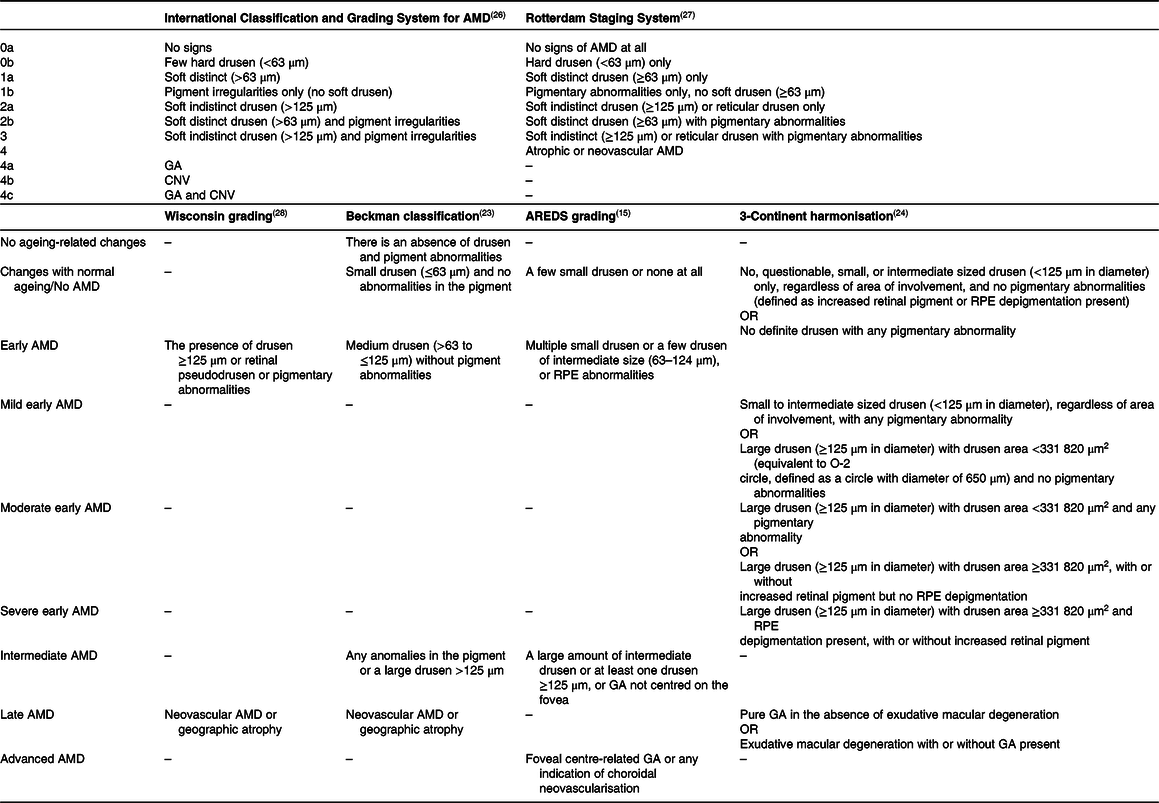
AMD, age-related macular degeneration; CNV, choroidal neovascularisation; GA, geographic atrophy; RPE, retinal pigmented epithelium.
Drusen are one of the first signs of dry AMD. They are small white-yellow deposits between the RPE and the underlying Bruch’s membrane. Ubiquitin, integrins, complement, collagen, fibronectins and β-amyloids accumulate in the drusen structure(Reference Imamura, Noda and Hashizume29,Reference Rastoin, Pagès and Dufies30) . The transition from an initial stage of AMD to GA can be defined as the progression of AMD. The soft drusen are less distinct, and their growth typically involves gradual coalescence. Finally, the soft drusen become a crystalline form, indicative of GA(Reference Lim, Mitchell and Seddon31). GA, an advanced form of dry AMD, is characterised by the presence of drusen, deterioration of the choriocapillaris, photoreceptors, RPE and choriocapillaris, atrophic loss of the outer retina, and a slowly progressive loss of visual function(Reference Schaal, Rosenfeld and Gregori32–Reference Fleckenstein, Mitchell and Freund34).
Wet AMD or neovascular AMD is defined by the choriocapillaris-derived formation of new fragile vessels through a breach in the outer aspect of Bruch’s membrane, known as CNV, into the sub-pigment epithelial region. Macular oedema/haemorrhaging can occur as these new vessels penetrate the retina. This can lead to a severe RPE detachment and a fast loss of vision, resulting in the formation of a hard exudate and fibrous scar tissue in the macula(Reference Heesterbeek, Lorés-Motta and Hoyng35). Neovascular AMD is relatively infrequent compared with dry AMD; however, it is responsible for nearly 90% of significant central vision impairment resulting from AMD(Reference Flaxel, Adelman and Bailey36). A model of the healthy human eye and the alterations that occur during the early/intermediate and late stages of AMD is given in Fig. 1. Modified risk factors such as a diet that can modulate these alterations are significant to manage this process positively and in a timely manner.

Fig. 1. Model of the healthy human eye and the alterations that occur during the early/intermediate and late stages of AMD(Reference Armento, Ueffing and Clark17). BM, Bruch’s membrane; CH, choroid; CNV, choroidal neovascularisation; GCL, ganglion cell layer; INL, inner nuclear layer; ONL, outer nuclear layer; RPD, reticular pseudodrusen; RPE, retinal pigment epithelium.
Dietary components and patterns in AMD
The potential role of micronutrients in AMD is one of the most researched topics. In the literature, the formulations of AREDS and AREDS2 containing certain nutrients have demonstrated positive effects in managing AMD because of their antioxidant composition. The AREDS and AREDS2 formulation contains specific amounts of various vitamins and minerals. The former includes AREDS 500 mg of vitamin C, 400 IU of vitamin E, 15 mg of β-carotene, 80 mg of zinc oxide and 25 mg of copper oxide. The AREDS2, in addition to these components, also contains 10 mg of lutein, 2 mg of zeaxanthin, 350 mg of docosahexaenoic acid and 650 mg of eicosapentaenoic acid(15,Reference Chew, Clemons and SanGiovanni37) . Based on the available data, it is recommended that individuals with AMD increase their daily dietary intake with green leafy vegetables and consume oily fish at least twice a week. Furthermore, the Mediterranean diet has a beneficial effect on patients with AMD(Reference Carneiro and Andrade14).
Many commercially accessible dietary supplements for people with AMD have been developed, and they often refer to AREDS formulations, considering their possible effects. These supplements benefit older individuals with AMD who cannot meet their micronutrient dietary requirements. Once-daily intake can reduce severe vision loss and prevent the development of advanced AMD in people with AMD since the AREDS2 formulation contains 10 mg of lutein + 2 mg of zeaxanthin. It has been reported that using supplements that contain β-carotene is not recommended for individuals who are current or former smokers. In addition, dietary modifications can prevent the onset and progression of AMD(Reference Chew, Clemons and SanGiovanni37,Reference Gorusupudi, Nelson and Bernstein38) . A recent systematic review and meta-analysis assessed the effect of nutritional supplements on individuals with AMD. The study found that taking supplements of lutein and zeaxanthin + omega-3 long-chain PUFAs resulted in significant improvements in best corrected visual acuity when compared with the control group (standardised mean difference: 4·59, 95% CI: 1·75, 7·43). Overall, multifocal electroretinogram data showed a considerable improvement following lutein + zeaxanthin intake (standardised mean difference: 4·59, 95% CI: 1·75, 7·43)(Reference Csader, Korhonen and Kaarniranta39). Specific human clinical trials focused on the effect of micronutrients in AMD are presented in Table 2.
Table 2. Certain human clinical trials focused on the effect of micronutrients in AMD.

AMD, age-related macular degeneration; CI, confidence interval; FFQ, food frequency questionnaire; HR, hazard ratio; I, intervention group; MPOD, macular pigment optical density; NHANES, National Health and Nutrition Examination Survey; OR, odds ratio; P, placebo group; RCT, randomised controlled trial; RR, relative risk.
Oral dietary supplements are easily accessible, non-invasive and cost-effective, and do not require a medical prescription. On the other hand, dietary supplements were formulated to respond to the nutritional requirements of individuals in optimal conditions to sustain or enhance their wellbeing. They did not serve as treatment for medical issues and were not designed for medical use. Also, a dietary supplement based on the AREDS2 formulation has no known adverse side effects. Compliance with supplements recommended by AMD professionals is high among patients(Reference Dave, Binns and Vinuela-Navarro56). Nevertheless, more consensus is required regarding the effects of AREDS-based supplements on AMD prevention. Furthermore, it should be noted that the AREDS2 trial only enrolled people with intermediate AMD, thereby constraining the generalisability of the findings to other types of AMD. Now, ophthalmologists and other healthcare professionals caring for patients with AMD should consider individual differences and potential adverse effects when recommending dietary supplements(Reference Carneiro and Andrade14,Reference Parodi, Zucchiatti and Cicinelli57) .
Research indicates that individuals with varying risk alleles for AMD may benefit from customised nutritional therapies. However, there needs to be more current information regarding the impact of genetics and various dietary components on the prognosis of AMD. Consequently, obtaining knowledge regarding the optimal nutritional supplements is crucial before carrying out more studies on dietary supplements, such as trials targeting carefully categorised subgroups of patients with AMD while considering genetic data(Reference Csader, Korhonen and Kaarniranta39,Reference Awh, Lane and Hawken58) .
Vitamin A and carotenoids
The typical visual function requires the presence of vitamin A, and any inadequacy of this vitamin leads to visual dysfunction(Reference Alvarez, Vaz and Gronemeyer59). In addition, vitamin A is a crucial component in various metabolic processes, including but not limited to promoting the growth of the eye’s epithelial tissue, participating in the visual cycle through biochemical transformations, facilitating the re-synthesis of rhodopsin, which is essential for mesopic vision, enhancing epithelial function and preventing conjunctival desiccation(Reference Biswal, Justis and Han60,Reference Paradowska and Kucharska61) .
The most common vitamin A compounds in foods derived from animals, including dairy products, eggs, fish, meat and liver, are retinol and retinyl esters. Provitamin A carotenoids, including β-carotene, α-carotene and β-cryptoxanthin, present in plant-based foods, convert to vitamin A within the organism. Sources of provitamin A go through metabolic processes that produce vitamin A, which is utilised by the organism according to its physiological needs. The rest of the carotenoids retain their antioxidant properties, such as lutein, zeaxanthin and lycopene(Reference Carazo, Macáková and Matoušová62,Reference Sajovic, Meglič and Glavač63) . Carotenoids are potent antioxidants that can reduce the systemic oxidative stress that influences the macula. α-Carotene, β-carotene and lycopene have been observed in the human RPE and choroid. These compounds protect these tissues against oxidative damage caused by exposure to light and the generation of free radicals within the local environment(Reference Rinninella, Mele and Merendino21).
Vitamin A has been associated with various retinal diseases, directly or indirectly. Some diseases are linked to pathogenic variations in genes that encode proteins that play a direct role in the vitamin A pathways in the retina. This can result in a deficiency of vitamin A in the affected area and/or the formation of bis retinoids. In the membranes of the outer photoreceptor segments, toxic bis retinoids are transformed into the bis retinoid pyridinium compound A2E and related bis retinoids. Due to the postmitotic character of RPE cells and the inability of A2E to go through further metabolism, the accumulation of A2E and its related bis retinoids occurs in the form of lipofuscin deposits within the RPE. Lipofuscin accumulation, which plays a role in the pathogenesis of AMD, impairs the functionality and longevity of RPE cells, leading to the degeneration of photoreceptors and consequent visual impairment. Others include local deficit brought on by the indirect transfer of vitamin A from the choroid to the retina or visual impairment induced by malfunction of the visual pigments. Since AMD results in the formation of bis retinoids, vitamin A supplementation should be controlled in those with AMD(Reference Biswal, Justis and Han60,Reference Paradowska and Kucharska61,Reference Sajovic, Meglič and Glavač63) .
Lutein, zeaxanthin and meso-zeaxanthin are macular xanthophylls synthesised from lutein within the retina(Reference Subczynski, Wisniewska and Widomska64). High levels of macular xanthophylls are found in the macular lutea of the eye. The centre of the fovea is known to possess the highest concentration of lutein and zeaxanthin; the lens also contains both(Reference Manayi, Abdollahi and Raman65,Reference Johra, Bepari and Bristy66) . When lutein and zeaxanthin interact with free radicals, lycopene takes in their unpaired electrons(Reference Paradowska and Kucharska61). Lutein and zeaxanthin levels are high in eggs and a variety of fruits and vegetables, especially green leafy vegetables, including kale, spinach, broccoli and parsley(Reference Koçyiğit and Nilüfer5,Reference Ranard, Jeon and Mohn67) .
Lutein and zeaxanthin can selectively absorb blue light and decrease oxidative stress in the retina, impeding the formation of reactive oxygen species, particularly singlet oxygen, within the retinal tissue. The mechanisms of lutein and zeaxanthin include their antioxidant, antiangiogenic and anti-inflammatory capabilities, as well as their ability to prevent phototoxic damage by absorbing solar radiation, to lessen oxidative stress by scavenging ROS and to reduce inflammation(Reference Narimatsu, Negishi and Miyake68,Reference Mrowicka, Mrowicki and Kucharska69) . Moreover, the pi-class glutathione S-transferase isoform, known as GSTP1, along with the zeaxanthin- and mesozeaxanthin-binding protein in the retina, are significant in protecting the retina against damage from oxidation and regulating the antioxidant levels in the macula(Reference Li, Vachali and Bernstein70). Similarly, the human retinal lutein binding protein significantly improves the localisation of lutein to the area under oxidative stress(Reference Hammond, Fletcher and Elliott71).
According to a prospective cohort study based on population, a longer intake of fruits and vegetables containing provitamin A carotenoid has decreased the risk of developing AMD(Reference de Koning-Backus, Buitendijk and Kiefte-de Jong72). However, epidemiological research indicates that dietary vitamin A intake does not lower the risk of AMD(Reference Seddon, Ajani and Sperduto40,Reference Delcourt, Cristol and Tessier73) . Supplementation with carotenoids has the potential to reduce the risk of developing AMD and other ocular diseases. The effect of dietary supplements containing β-carotene on the development and onset of AMD was assessed in a significant clinical study known as the AREDS. However, research indicates that high dosages of β-carotene (30 mg/d) may be detrimental to smokers and may increase the risk of lung cancer, making the use of β-carotene supplements inconsistent(15). It is recommended to avoid taking β-carotene supplements to individuals diagnosed with AMD who smoke. As a result, the revised AREDS2 formula has substituted β-carotene with lutein and zeaxanthin. Research has demonstrated that regular use of AREDS2 is associated with a lower risk of developing advanced AMD(Reference Chew, Clemons and SanGiovanni37).
In recent years, it has been suggested that lutein and zeaxanthin not only inhibit the formation of AMD but also its progression(Reference Walchuk and Suh18,Reference Huang, Coleman and Kim74) . In the Blue Mountains Eye Study, people who consume higher amounts of lutein and zeaxanthin exhibit a lower risk of developing drusen or AMD. However, a significant positive correlation between dietary β-carotene intake and AMD was discovered(Reference Tan, Wang and Flood45). Furthermore, a number of research studies have demonstrated that the intake of lutein may enhance the optical density of macular pigment(Reference Weigert, Kaya and Pemp47,Reference Ma, Yan and Huang75) . The decline in the concentrations of lutein, zeaxanthin and mesozeaxanthin within the ageing retina is a contributing factor to the important progress of AMD. Support for therapy with these carotenoids may be effective in addition to clinical treatment. The ageing retina has a substantial decline in its natural antioxidant capacity as lutein, zeaxanthin and meso-zeaxanthin levels decrease. This factor plays a crucial role in the advancement of the disease.
The use of carotenoid vitamin treatment as an additional nutraceutical approach in persons diagnosed with AMD has demonstrated positive clinical outcomes in terms of visual function.
Vitamin D
Two major forms of vitamin D are present, namely vitamin D2 (ergocalciferol) derived from plants and vitamin D3 (cholecalciferol) obtained from animal-based food sources (animal fats, eggs and fish oil). In humans, vitamin D3 is produced by the photolysis of 7-dehydrocholesterol when skin is exposed to sunlight (UV-B radiation). Vitamin D3 is synthesised by the skin and then undergoes two essential hydroxylation processes in the liver (25 (OH) hydroxylation) and kidneys (1,25 (OH)2 hydroxylation)(Reference Holick76,Reference Garcia Layana, Minnella and Garhöfer77) .
The expression of the vitamin D receptor and enzymes associated with vitamin D metabolism (CYP27B1 and CYP24A1) in the retina, RPE and choroid indicates a possible physiological function for vitamin D in AMD. Vitamin D has been found to protect against free radicals in the retina. Additionally, it has been observed to inhibit inflammation by preventing the accumulation of amyloid β plaques and suppressing pro-inflammatory cytokines that are secreted by macrophages and microglia. Furthermore, it has been found to inhibit angiogenesis through the inhibition of hypoxia-inducible factor (HIF-1) transcription, induction of endothelial cell apoptosis and metalloproteinase MMP-9(Reference Rinninella, Mele and Merendino21,Reference Garcia Layana, Minnella and Garhöfer77,Reference Morrison, Silveira and Huynh78) .
The association between vitamin D and AMD is a still developing field of study. The findings of a meta-analysis indicate a statistically significant association between late AMD and low circulating levels of 25-hydroxyvitamin D (25(OH)D) below 50 nmol/l (20 ng/ml)(Reference Annweiler, Drouet and Duval79). Observational studies(Reference Millen, Voland and Sondel80,Reference Itty, Day and Lyles81) and a case–control study(Reference Kabataş, Doğan and Yılmaz82) have demonstrated a negative correlation between plasma vitamin D levels and either early or late atrophic or neovascular AMD. Further research is required due to the heterogeneity in current studies and the lack of prospective studies.
The literature lacks sufficient research to assess how vitamin D supplementation affects AMD incidence and progression. In the United States, a study showed that there is a negative correlation between the early onset of AMD and the intake of milk enriched with vitamin D, which contains an additional 400 IU added to a quart or 946 ml of milk(Reference Parekh, Chappell and Millen43). The study found that there was a decrease in the severity of AMD and a reduction in the size of drusen in individuals with higher dietary vitamin D intake (a food frequency questionnaire was used to collect data) as compared with their identical twins who had lower dietary vitamin D intake(Reference Seddon, Reynolds and Shah83). Merle et al.(Reference Merle, Silver and Rosner54) showed that a diet rich in Vitamin D may prevent or delay progression to advanced AMD, especially neovascular AMD. The increase in dietary vitamin D intake has been shown to have a protective impact against AMD in the meta-analysis(Reference Annweiler, Drouet and Duval79) and recent prospective research(Reference Jacob, Mangelschots and Michez84). Vitamin D possesses antioxidant and anti-inflammatory capabilities inside the macula, which may have a protective impact on RPE and choroid cells by mitigating oxidative stress, inflammation and apoptosis. Future clinical studies examining the association between vitamin D and AMD will be prompted by possible effects of vitamin D.
Vitamin E
Vitamin E is a fat-soluble antioxidant, found in the cellular membrane of photoreceptors and the RPE, and an effective scavenger of free radicals. Age-related changes in the eyes are increased in cases of vitamin E deficiency. A deficiency of vitamin E may result in an accumulation of lipofuscin, impairment of the retina, and depletion of photoreceptor cells(Reference Tanito, Yoshida and Kaidzu85,Reference Khoo, Ng and Yap86) . Eight distinct chemical forms of vitamin E occur naturally, with α-tocopherol being the most biologically active among them(Reference Edwards, Olson and Euritt87). Dietary products rich in vitamin E include almonds, walnuts, olive oil, rapeseed oil, hazelnuts, fish, oysters, eggs and butter(Reference Akram, Munir and Daniyal88).
The Rotterdam Study, a population-based cohort that includes individuals aged 55 and above, has revealed an inverse correlation between dietary vitamin E intake and AMD after an 8-year follow-up period(Reference Van Leeuwen, Boekhoorn and Vingerling41), whereas the Eye Disease Case-control Study Group did not observe a statistically significant interaction between the risk of AMD and vitamin E(Reference Seddon, Ajani and Sperduto40). Furthermore, in the population cohort Blue Mountains Eye Study, the participants were divided into three groups based on their vitamin E intake, and a significant correlation was found between vitamin E intake and late AMD incidence in individuals who consumed moderate and high amounts of vitamin E(Reference Tan, Wang and Flood45).
A high vitamin E supplementation dose may not always be beneficial for eye health according to the previous studies. The recommended tolerated upper intake level for synthetic vitamin E is 1100 IU. Higher dosages may result in bleeding diathesis, particularly in those using anticoagulants and antiaggregants. Furthermore, a research study revealed that increased intake of vitamin E was associated with a higher risk of developing prostate cancer(Reference Booth, Golly and Sacheck89,Reference Klein, Thompson and Tangen90) . Clinical studies have demonstrated potential benefits of vitamin E for individuals diagnosed with AMD. Literature suggests that the use of vitamin E supplements, in addition to a regular diet, may have adverse effects and should be administered by medical professionals.
Vitamin C
Vitamin C (ascorbic acid) is a potent antioxidant that is soluble in water and non-enzymatic in nature. Its primary function is to protect proteins, lipids, carbohydrates and nucleic acids from the detrimental effects of free radicals and reactive oxygen species. Many fruits (i.e. oranges, lemons, mangoes, strawberries and blueberries) and vegetables (i.e. broccoli, peppers, potatoes, cauliflower, leafy greens and tomatoes) are considered to be great sources of vitamin C(Reference Andreatta and El-Sherbiny91).
Through its powerful antioxidant effects, vitamin C benefits eye health and reduces the risk of AMD. Although vitamin C has been shown to have beneficial effects on eye health, the findings from various studies investigating the association between dietary vitamin C intake and the risk of AMD are uncertain(Reference Schmidl, Garhoefer and Schmetterer92,Reference Parodi, Mollo and Brunoro93) . In a systematic review and meta-analysis conducted by Chong et al., antioxidant compounds, such as vitamin C, did not significantly contribute to the primary prevention of early AMD(Reference Chong, Wong and Kreis42). A case–control study showed that dietary intake of vitamin C was associated with a reduced risk of neovascular AMD(Reference Aoki, Inoue and Nguyen52). A Cochrane analysis reported that there was no evidence that vitamin C alone prevents the development of AMD(Reference Evans and Lawrenson94). The outcome was consistent with the research findings described in the manuscript by Delcourt et al. There was no statistically significant association between the intake of vitamin C and the incidence of AMD(Reference de Koning-Backus, Buitendijk and Kiefte-de Jong72). Considering the positive effects of vitamin C and the findings of the research, further studies employing larger cohorts are necessary to assess the adequacy of vitamin C in reducing the risk of AMD.
B vitamins
The B vitamins, especially B6, folate and B12, play a regulatory role in homocysteine levels(Reference Pinna, Zaccheddu and Boscia95). Homocysteine is a powerful oxidiser that has the potential to aggravate injury caused by oxidative stress. Elevated levels of homocysteine in the serum have been found to have a direct impact on the damage of epithelial tissue and the disruption of the junction of RPE, potentially resulting in the development of neovascularisation. Increased levels of homocysteine have the potential to stimulate inflammatory processes that can lead to the development of atherosclerosis. High levels of homocysteine have been identified as a possible risk factor for AMD(Reference Silverman, Tumuluri and Davis96,Reference Fritsche, Fariss and Stambolian97) .
Studies in the field of epidemiology have established a correlation between increased consumption of vitamin B6 and folate and a decreased risk of developing AMD(Reference Christen, Cook and Chiuve98–Reference Merle, Silver and Rosner100). In a 10-year population-based cohort study, an inverse correlation was found between the total intake of vitamin B12 and folate equivalents and the incidence of AMD over a period of 10 years. These correlations were not affected by potential confounders including smoking, leucocyte count, fish consumption, renal function or antioxidant intake(Reference Gopinath, Flood and Rochtchina49). A randomised, double-blind, placebo-controlled study with females showed that women with high cardiovascular disease risk who used a combination of folic acid (2·5 mg/d), vitamin B6 (50 mg/d) and vitamin B12 (1 mg/d) for an average duration of 7·3 years had a decrease in AMD risk by 35–40%(Reference Christen, Glynn and Chew46).
AMD risk is increased by low plasma folate and B12 concentrations(Reference Gopinath, Flood and Rochtchina49,Reference Kamburoglu, Gumus and Kadayıfcılar101) . The Alienor Study assessed the association between the diet and plasma levels of B vitamins, including folate, vitamin B6 and vitamin B12. The study findings indicated that maintaining normal serum folate levels (≥10 nmol/l) may lead to a 51% reduction in the risk of developing AMD. Additionally, a 28% decrease in risk was observed in individuals who consumed higher dietary vitamins B5 and B6 (Reference Merle, Barthes and Féart102). Research indicates that B vitamins play a beneficial role in preventing the onset and advancement of macular degeneration. Reducing AMD-related vision loss may be possible by using a balanced diet high in B vitamins.
Zinc
It has been determined that approximately 30% of older adults in industrialised countries have a zinc deficiency, and that zinc may play a significant role in AMD and other ageing-related degenerative diseases(Reference Prasad103). Zinc-rich foods include pork, chicken and beef. Zinc is present in legumes, cereals and nuts; however, plant-based phytates can inhibit zinc absorption(Reference McCusker, Durrani and Payette104). Zinc is involved in the process of rhodopsin regeneration and the phototransduction cascade in photoreceptor cells. It is an antioxidant ion that serves as a cofactor for several ocular enzymes that are involved in the oxidative process, including superoxide dismutase and catalase(Reference Ugarte and Osborne105,Reference Ferrington, Ebeling and Kapphahn106) . Zinc also helps suppress the chronic inflammation in the retina that contributes to AMD by binding complement factor H and inducing the loss of complement 3b’s inhibitory activity(Reference Nan, Tetchner and Rodriguez107).
In AMD, the level of zinc in the human RPE/choroid is reduced by 24%(Reference Ugarte and Osborne105). Smailhodzic et al. found that the daily intake of 50 mg of zinc sulphate had an inhibitory effect on complement catabolism in patients with age-related AMD who exhibited increased complement activation(Reference Smailhodzic, van Asten and Blom51). The systematic review determined that the current evidence regarding the effectiveness of zinc intake in preventing AMD was not conclusive(Reference Vishwanathan, Chung and Johnson108). AREDS study showed that the use of a zinc supplement (80 mg) alone resulted in a decrease in the risk of developing advanced AMD in individuals with intermediate AMD and those with advanced AMD in one eye. The risk of losing visual acuity in AREDS patients was not decreased by zinc treatment alone. The formulation of AREDS included a copper supplementation of 2 mg/d, as it has been observed that elevated levels of zinc can inhibit the absorption of copper(15). The AREDS2 clinical trial investigated the impact of a reduced dosage of zinc (25 mg versus 80 mg) on the progression of AMD, but no statistically significant difference was observed(109). The Blue Mountains Eye Study suggested a threshold dose of 15·8 mg to prevent any adverse effects(Reference Tan, Wang and Flood45). Zinc has shown potential for preventing the progression of advanced AMD. Nevertheless, it should be noted that the use of zinc alone may not yield substantial clinical improvements in visual acuity. When combined with antioxidants, zinc can enhance visual acuity in patients with AMD.
Copper and selenium
Copper plays a significant role in the scavenging and reduction of free radicals due to its unique capacity to quickly acquire and distribute electrons(Reference Mares, Millen and Lawler110). Due to the possibility that high zinc dosages might result in copper deficiency anaemia, 2 mg of copper oxide was included in the formulation of AREDS(15).
Selenium has been identified as a constituent element in various enzymes within the organism and has been observed to exhibit antioxidant characteristics. Glutathione peroxidase that is selenium dependent is crucial in preventing oxidative damage to membrane lipids. It was hypothesised that the macula may be protected from oxidative damage by glutathione peroxidase because it had a lowering effect on hydrogen peroxide and other potential hydroperoxides in the cell(Reference Tsang, Penfold and Snitch111–Reference Zampatti, Ricci and Cusumano113). Furthermore, it was determined that a low intake of selenium in the laboratory animals resulted in a reduction of overall polyunsaturated fatty acids in both the RPE and the outer segments of the retinal rods(Reference Tokarz, Kaarniranta and Blasiak114).
Over recent years, many studies have been conducted to investigate the impact of selenium on AMD(Reference Tsang, Penfold and Snitch111,112) . Nevertheless, the outcomes of these investigations have not yielded conclusive evidence. Consumption of an excessive amount of selenium has been found to be detrimental to the human body, leading to adverse effects on endocrine and neurological functioning, as well as causing hepatotoxicity and gastrointestinal problems. Furthermore, it is widely recognised that there exists an antagonistic interaction among zinc and copper. Elevated consumption of zinc in the diet has been seen to result in a decrease in the concentration of copper. Further investigation is required to investigate the relationship between selenium and copper with AMD and ocular diseases.
Genetics and dietary supplements
The importance of genetic testing prior to supplement selection and the potential effects of supplements on AMD within distinct genotype groups are topics of continuing research discussion. The two genes most closely linked to AMD and its development are CFH and ARMS2. Subjects who have homozygous CFH and ARMS2 risk alleles exhibit an increased tendency for disease progression compared with those who have homozygous non-risk alleles(Reference Seddon, Francis and George115,Reference Seddon, Silver and Rosner116) . A recent large GWAS has identified the first genetic association specific to wet AMD near the matrix metallopeptidase 9 (MMP9) gene. Rare coding variations in TIMP metallopeptidase inhibitor 3 (TIMP3), complement factor I (CFI) and CFH as well as a splice variant in solute carrier family 16 member 8 (SLC16A8) have been suggested as having causal involvement for these genes(Reference Fritsche, Igl and Bailey117).
The use of zinc supplementation did not result in any beneficial outcomes for individuals with one or two CFH risk alleles during a 10·2-year duration, as evidenced by a study. However, the same individuals were able to derive the maximum benefit from antioxidant therapy (in the AREDS formulation). Individuals with the ARMS2 risk allele reported that antioxidant treatment alone increased disease progression(Reference Awh, Lane and Hawken58). The authors acknowledged the observation that the efficacy of antioxidants diminished in the presence of two ARMS2 risk alleles, an outcome that can be attributed to their impact on mitochondrial localisation and consequent oxidative phosphorylation(Reference Fritsche, Loenhardt and Janssen118,Reference Mukhtar and Ambati119) . The research findings indicate that the mean 10-year progression rates for patients administered with placebo, AREDS formulation and genotype-directed therapy were 47%, 40·5% and 31·7%, respectively, based on the distribution of genetic risk alleles. These results suggest the potential effectiveness of genotype-directed nutritional therapy(Reference Awh, Lane and Hawken58). Based on the findings of the Rotterdam Study, an intake of dietary antioxidants could decrease the risk of early AMD in individuals with a high genetic risk for CFH and ARMS2 (Reference Ho, van Leeuwen and Witteman120). In addition, genetic differences may account for 27% of variation in response to lutein and zeaxanthin supplementation during a 6-month period among women participants in the AREDS study, as well as 67% of variation in macular pigment optical density(Reference Hammond, Liew and Van Kuijk121,Reference Meyers, Johnson and Bernstein122) .
The growing importance of genetic research and the identification of risk alleles in individuals diagnosed with AMD may facilitate the progression of nutrigenomic research. Thus, the encouragement of the selection of dietary modifications that exhibit positive effects on AMD in particular genotype groups may be enabled. However, future investigations are needed to decide on the role and possibility of the usage of dietary supplements and effectiveness regarding personalised medicine in AMD.
Dietary fats
Dietary fats can be classified mainly as saturated fatty acids (SFA) and unsaturated fatty acids (monounsaturated (MUFA) and polyunsaturated (PUFA))(Reference Tvrzicka, Kremmyda and Stankova123). Several studies have been conducted to clarify the impact of different dietary habits on AMD development. Therefore, several attempts have been performed to define the connection between dietary fat intake and the risk of AMD(Reference Tan, Wang and Flood124–Reference Parekh, Voland and Moeller129).
Saturated fatty acids
Although the results of the studies vary, several researchers have evaluated the link between the consumption of SFA and AMD(Reference Tan, Wang and Flood124–Reference Mares-Perlman, Brady and Klein130). Some scientific evidence points to a link between dietary fat intake – particularly SFA and cholesterol – and an elevated risk of atherosclerosis. Therefore, consuming excess SFA can encourage atherosclerosis and increase the risk of AMD. The proposed mechanism is that the presence of common risk factors between cardiovascular disease and AMD, such as dietary fat, which is linked to atherosclerosis, may have an impact on the blood vessels in the eyes or may participate in oxidative processes that contribute to the progression of AMD in advanced stages, which are characterised by choroidal atrophy or neovascularisation(Reference Snow and Seddon131) (Fig. 2). Mares-Perlman et al. found an elevated risk of early AMD in individuals with the highest intake of cholesterol and SFA(Reference Mares-Perlman, Brady and Klein130).

Fig. 2. Potential relationship between the excess intake of saturated fatty acids and AMD risk.
Similarly, Seddon et al. described a trend for an increase in risk for higher intake of SFA for the highest intake compared with the lowest intake (odds ratio (OR) 2·03, p = 0·07)(Reference Seddon, Cote and Rosner132). Delcourt et al. revealed an association between SFA intake and increased risk for AMD (OR 2·70, p = 0·04)(Reference Delcourt, Carrière and Cristol128), and according to a study by Cho et al., people who consume a high amount of SFA in their diet have a slightly increased chance of developing AMD(Reference Cho, Hung and Willett126). In another study, higher SFA intake was associated with a higher prevalence of overall intermediate AMD in females <75 years (p = 0·01)(Reference Parekh, Voland and Moeller129), and Smith et al. reported a borderline significant association between SFA intake and the risk for early AMD(Reference Smith, Mitchell and Leeder125). In contrast to the previous studies, Sasaki et al. found that participants in the highest quartile of SFA intake were less likely to have early AMD compared with the lowest quartile (OR 0·71, 95% CI: 0·52–0·96), and a significant trend for decreased risk of early AMD with increased SFA intake was determined (p = 0·011)(Reference Sasaki, Harada and Tsubota133). Similarly, Yasukawa et al. revealed that SFA intake was inversely associated with the prevalence of AMD in males (OR 0·86), and significant trends were found for decreasing AMD with increased SFA (p = 0·02)(Reference Yasukawa, Sasaki and Motomura134). In a meta-analysis by Zhong et al., a 10 g/d increment in SFA was not correlated with the risk of any AMD, and there was no proof of a non-linear association between dietary SFA and early and advanced AMD risk (p = 0·675 and p = 0·814, respectively)(Reference Zhong, Wang and Jiang135). Likewise, Tan et al. and Seddon et al. found no significant association between the intake of SFA and AMD(Reference Tan, Wang and Flood124,Reference Seddon, Rosner and Sperduto136) . Diets that are high in saturated fat encourage the flowering of bacteria that produce endotoxins and break down sulphates, including Bilophila wadsworthia (Proteobacteria phylum), which is linked to the degeneration of mucus layers and low-grade inflammation(Reference Devkota, Wang and Musch137). Since inflammation is the basis of AMD, its association with saturated fat may be relevant. In addition, cholesterol is the primary sterol in the retina. It is extensively present in the drusen, a defining feature of early AMD, and the risk of AMD is increased by a diet high in cholesterol and saturated fat(Reference Pikuleva and Curcio138,Reference Klein, Cruickshanks and Nash139) . ApoB can produce lipoprotein-like particles that build up in Bruch’s membrane with ageing. RPE and photoreceptor outer segments are used to assemble lipoprotein lipids. Additionally, there was evidence that the microbiota affected systemic lipids, and systemic lipid dysregulation was a component of AMD. Large cholesteryl ester-rich lipoproteins built up in healthy people’s macular Bruch membrane(Reference Curcio, Johnson and Huang140,Reference Curcio, Johnson and Huang141) . In addition, the TT genotype of the hepatic lipase gene, LIPC, was linked to a 50% reduction in risk for AMD, compared with individuals with the CC variation, in genes implicated in cholesterol transport(Reference Hadley, Orlin and Brown142). The intricacy of this relationship between SFA and AMD is further compounded by other factors, including the impact of microbiota on lipid metabolism, genetic polymorphisms associated with cholesterol transport, and lipid dysregulation in AMD. Although some studies suggest a potential link between SFA and the risk of AMD, the varied results and the complex nature of AMD’s multifaceted aetiology necessitate further research to gain a more comprehensive understanding of the intricate interplay among dietary fats, lipid metabolism, genetic factors and the onset of AMD.
Monounsaturated fatty acids
Parekh et al.(Reference Parekh, Voland and Moeller129) demonstrated the role of MUFAs as a preventative factor for intermediate AMD. In a study by Delcourt et al., after adjustments for age and gender, the risk of AMD was increased in subjects with a high intake of MUFA (OR 3·50, p = 0·03)(Reference Delcourt, Carrière and Cristol128). The Blue Mountains Eye Study reported an OR of 1·48 for MUFA for early AMD(Reference Smith, Mitchell and Leeder125), and MUFA increased the likelihood of progression of AMD in another study (Relative risk (RR)2·21, p = 0·04)(Reference Seddon, Cote and Rosner132). Additionally, a higher risk of AMD development was linked to a diet high in MUFA(Reference Agrón, Mares and Clemons143), and Seddon et al. found a significant relationship between MUFA and advanced AMD (OR 1·71, p = 0·03). It was thought that this might be because MUFA sources are also a source of SFA, and after additional adjustments for protein and SFA, PUFA and trans saturated fats, it was determined that the relationship was no longer statistically significant(Reference Seddon, Rosner and Sperduto136). The AREDS team suggested a possible justification for these disparate outcomes: People who consumed the most MUFAs had a higher risk of developing neovascular AMD. However, this link disappeared when docosahexaenoic acid (DHA), arachidonic acid and other nutrients (lutein/zeaxanthin) were considered(Reference SanGiovanni, Chew and Clemons127). In a meta-analysis, consumption of MUFA was not significantly associated with early or advanced AMD(Reference Zhong, Wang and Jiang135). Similarly, Sasaki et al. did not find any significant relationship in their study(Reference Sasaki, Harada and Tsubota133). Oleic acid is one of the MUFAs that may protect against AMD. However, only a few studies have looked at this relationship. In studies, olive oil, one of the primary dietary sources of oleic acid, has been strongly linked to a lower risk of AMD(Reference Cougnard-Gregoire, Delyfer and Korobelnik144,Reference Chong, Robman and Simpson145) .
Furthermore, in addition to oleic acid, olive oil also includes other substances, such as polyphenols, that may play a protective function in AMD. It is, therefore, challenging to determine if these health advantages are due to oleic acid or other olive oil substances(Reference van Leeuwen, Emri and Merle146). A study determined that after adjustment for potential confounders, regular use of olive oil was significantly associated with a decreased risk of late AMD (OR 0·44). However, there were no statistically significant correlations between regular olive oil consumption and the CFH rs1061170, ARMS2 rs10490924, LPL rs12678919 or LIPC rs493258 polymorphisms, which are genetic risk factors for AMD(Reference Cougnard-Gregoire, Merle and Korobelnik147). The bioactive components of olive oil may be responsible for the preventive mechanisms behind the link between the consumption of olive oil and lower odds of late AMD. The unsaponifiable fraction of olive oil consists of phenolic substances with antioxidant and anti-inflammatory characteristics, such as oleuropein, hydroxytyrosol, tyrosol and oleocanthal(Reference Cicerale, Lucas and Keast148). Oleocanthal, a substance that resembles the non-steroidal anti-inflammatory medicine ibuprofen, appears to be the primary mediator of the anti-inflammatory effects(Reference Parkinson and Keast149). Additionally, hydroxytyrosol and oleuropein, which have antioxidant properties, inhibit the oxidation of LDL-cholesterol and decrease the expression of genes and proteins that control inflammation(Reference Castaner, Covas and Khymenets150,Reference Lockyer and Rowland151) . In addition, hydroxytyrosol protects against mitochondrial malfunction and oxidative damage in retinal pigment epithelial cells in vitro (Reference Zhu, Liu and Feng152).
The interaction of MUFAs, constituents of olive oil, and the AMD encompasses several processes, encompassing the effects of reducing inflammation and oxidative stress. However, in addition to the aforementioned points, it is evident that the consumption of monounsaturated fatty acids (MUFAs), particularly olive oil, and therefore the adherence to the Mediterranean diet, provide a multitude of advantageous effects on human health. In this particular situation, it is recommended to keep to the consumption of MUFAs in accordance with the prevailing dietary recommendations.
Polyunsaturated fatty acids
In the body, PUFAs (linoleic acid (LA) and α-linolenic acid (ALA)) are converted to very-long-chain PUFAs by fatty acyl-CoA synthetases, and their respective elongases designated as elongation of very long fatty acids. Eicosapentaenoic acid (EPA) and docosahexaenoic acid (DHA) are among the very-long-chain PUFAs(Reference Saini and Keum153). The suggested AMD pathogenesis and progression mechanism is cumulative oxidative stress in the RPE. Because of their antioxidative and anti-inflammatory properties(Reference Djuricic and Calder154), marine-based omega-3 polyunsaturated fatty acids (EPA and DHA) in the retina were suggested to reduce the risk of AMD(Reference Khandhadia and Lotery155). Moreover, DHA is assumed to support retinal photoreceptor cell development because it is a major lipid component of the outer segments of the retina(Reference Abokyi, To and Lam156). Other suggested mechanisms are the inhibition of choroidal neovascularisation by omega-3 fatty acid, the interaction of triacylglycerol lipoproteins in RPE cells to delay AMD, structural replacement of omega-3 fatty acid with omega-6 fatty acid, induction of cellular responses, and mobilisation of cellular resistance to misfolded proteins and oxidative stress(Reference Fan and Song157). In a prospective cohort study by Wu et al., the hazard ratio (HR) for DHA compared with the extreme quintiles of intake for intermediate AMD was 0·78 (p = 0·008), and for EPA + DHA, it was 0·83 (p = 0·03)(Reference Wu, Cho and Giovannucci158). Also, in a meta-analysis, a high dietary intake of omega-3 fatty acid was associated with a 38% reduction (OR 0·62) in the risk of late AMD(Reference Chong, Kreis and Wong159). As a result of another meta-analysis, higher omega-3 fatty acid intake was associated with a 14% (RR 0·86) and 29% (RR 0·71) lower risk of early and late AMD, respectively. In addition, the dose–response analysis revealed that each additional 1 g/d omega-3 fatty acid intake reduced the risk of early and late AMD by 6% and 22%, respectively. Intake of omega-3 fatty acid, EPA and DHA was inversely related to lower AMD risk(Reference Jiang, Shi and Fan160). According to a prospective cohort study from the AREDS, individuals with the highest omega-3 fatty acid consumption had a 30% lower risk of central geographic atrophy and Neovascular AMD than others ((OR 0·65, p = 0·02) and (OR 0·68, p = 0·02), respectively)(Reference SanGiovanni, Agron and Meleth161). Similarly, in another report of AREDS, those who consumed the most EPA and EPA + DHA had a lower chance of developing central geographic atrophy, which is the next stage following bilateral drusen (OR 0·44 and OR 0·45, respectively)(Reference SanGiovanni, Chew and Agron162). Additionally, one of the primary sources of omega-3 fatty acid in the diet is the consumption of fish and fishery products, and one of the typical characteristics of them is being rich in omega-3 fatty acid, mainly EPA and DHA(Reference Gil and Gil163). Studies have examined the relationship between fish and fishery consumption and AMD(Reference Tan, Wang and Flood124,Reference Chong, Robman and Simpson145,Reference Wu, Cho and Giovannucci158,Reference Christen, Schaumberg and Glynn164–Reference Heuberger, Mares-Perlman and Klein166) . As a result of a study by Christen et al., women who consumed one or more fish per week, compared with those who consumed less than one serving per month, had an RR of AMD of 0·58 (p = 0·001). Dinu et al. conducted a meta-analysis study for fish consumption and AMD risk, and they determined a significant (p < 0·05) reduction of risk for total AMD, as well as for both early and late AMD (RR 0·82, RR 0·84 and RR 0·79, respectively)(Reference Dinu, Pagliai and Casini167). Also, according to the results of a meta-analysis by Jiang et al., the pooled RRs comparing extreme categories of fish intake were 0·79 and 0·71 for early and late AMD risk, and every 15 g/d of fish consumption was associated with a 13% and 14% lower risk of early and late AMD, respectively. In addition, fish intake was associated with a significantly reduced risk of AMD progression (RR 0·73)(Reference Jiang, Shi and Fan160). Excessive amounts of omega-6 fatty acid and a high omega-6/omega-3 fatty acid ratio, as is found in Western diets, promote the pathogenesis of many chronic diseases, including cardiovascular, cancer and autoimmune diseases. In contrast, increased levels of omega-3 fatty acid exert suppressive effects(Reference Liput, Lepczyński and Ogłuszka168). Mance et al. found that the omega-6/omega-3 fatty acid ratio was related to the development of neovascular AMD, and a decreased ratio protected against neovascular AMD(Reference Čaljkušić Mance, Kovačević and Alpeza-Dunato169). When omega-6 fatty acid and omega-3 fatty acid compete for the same enzymes in the cyclooxygenase and lipoxygenase pathways, a high intake of omega-6 fatty acids blocks the anti-inflammatory effects of omega-3 fatty acid. While omega-3 fatty acids produce anti-inflammatory and anti-angiogenic metabolites, omega-6 fatty acids produce pro-inflammatory metabolites(Reference Serhan170).
At the ends of developing blood vessels, matrix metalloproteinases (MMPs) dissolve the extracellular matrix, essential for the steady growth of new blood vessels. MMPs govern numerous elements of angiogenesis and inflammation, and omega-3 fatty acid contributes to the control of MMPs(Reference Shinto, Marracci and Bumgarner171). Animal studies further show that the adiponectin pathway is one of the mechanisms through which omega-3 fatty acid prevents choroidal neovascularisation. Human retinal microvascular endothelial cells revealed that the adiponectin pathway reduces MMP-2 and MMP-9 expression and ocular blood vessel development. Omega-3 fatty acid reduces MMP-2 and MMP-9 levels, phosphorylates AMPKa, activates the adiponectin pathway and inhibits the development of new blood vessels(Reference Fu, Liegl and Wang172). One of the neurodegenerative diseases defined by an accumulation of misfolded proteins is AMD. Damaged proteins may adversely affect cellular function. NFE2L2, also known as NRF2, is a crucial gene regulator of genes that influence the redox status of cells. By making it easier for NRF2 activation and autophagy to work together, DHA makes RPE cells more resistant to damage from misfolded proteins and oxidative stress. An increase in protein levels and the nuclear translocation of NRF2 followed a brief increase in ROS due to physiological levels of DHA. Later, gene expression arrays were used to find that the NRF2-regulated protein Sequestosome-1 (SQSTM1) had more of it and that the expression of NRF2-regulated genes had increased. SQSTM1 selectively instructs misfolded ubiquitinated proteins to be destroyed in the lysosome(Reference Johansson, Monsen and Pettersen173). In animal models, DHA has been shown to make photoreceptors live longer and protect them from apoptotic signs such as broken photoreceptor nuclei and mitochondrial dysfunction(Reference SanGiovanni and Chew174). From a genetic point of view, increased intake of DHA significantly protected geographical atrophy progression among those with the ARMS2/HTRA1-associated genes with AMD-homozygous risk genotype(Reference Reynolds, Rosner and Seddon175). The Blue Mountain Eye Disease study revealed that weekly fish consumption decreased the risk of progression to late AMD among individuals with the homozygous risk genotype for CFH. However, there were only forty-seven late AMD cases for both geographical atrophy and neovascularisation combined, and they did not discover any associations with early AMD(Reference Wang, Rochtchina and Smith176). The Rotterdam study found that people with the highest combined EPA and DHA intake had a lower chance of progressing to early AMD than those with the ARMS2/HTRA1 homozygous risk genotype(Reference Ho, van Leeuwen and Witteman120).
The maintenance of a proper balance between omega-6 fatty acid and omega-3 fatty acid is of greatest significance. Western diets, which tend to have elevated omega-6/omega-3 fatty acid ratios, may contribute to the development of AMD. The significance of maintaining an appropriate ratio is highlighted by the competition that occurs among these fatty acids for enzymes implicated in inflammatory pathways. It is consistently indicated that EPA and DHA, predominantly obtained from marine sources, as well as the consumption of fish, have a preventive role in mitigating the risk and advancement of AMD.
Dietary glycaemic index
The relationship between AMD and dietary carbohydrates is mainly related to the diet’s glycaemic index (GI). Jenkins et al. proposed the concept of the GI in 1981 to describe how fast carbohydrates were absorbed after a meal(Reference Jenkins, Wolever and Taylor177). GI is calculated as the difference between the area under the glucose response curve after consuming 50 g of a test food and the area under the curve after consuming 50 g of a control food, such as white bread or glucose(Reference Wolever, Jenkins and Jenkins178). The retina has one of the most extensive blood and nutrient supplies, including glucose. It depends on sufficient glucose transport from the systemic circulation to sustain its physiologic function, and retinal glucose reserves are insignificant compared with glucose demand(Reference Kumagai179). However, due to age-related inefficiencies or improper metabolism, such as the production of advanced glycation end-products (AGEs) and their aftereffects, sustained high glucose levels or glucose spikes can cause serious health problems(Reference Stitt180). The eye is particularly susceptible to harm from direct and indirect effects of persistently elevated blood glucose due to low capacity for cellular turnover, the limited roles of glucose transporters, and the high metabolic activity of the retina; therefore, AMD is linked to hyperglycaemia. The formation of AGEs and protein kinase C activation might cause the abnormal vasculature found in retinopathy(Reference Kearney, Fagan and Al-Qureshi181). Through the formation of AGEs, increased metabolism leads to the accumulation of reactive oxygen species and a decrease in glutathione, and protein kinase C activation leads to immunological hyperactivity. This increase in cellular glucose can cause cell damage and apoptosis(Reference Stitt182). According to a cross-sectional analysis of AREDS baseline data, the quantity and glycaemic quality of dietary carbohydrates may be related to the prevalence of nuclear and cortical opacities; for cortical opacities of any severity, the OR in a comparison of the highest with the lowest quartile of consumption was 1·27 (p = 0·09), and for all pure nuclear opacities it was 1·29 (p = 0·02)(Reference Chiu, Milton and Gensler183). In a case–control analysis of the Nutrition and Vision Project (NVP), a substudy of Nurses’ Health Study (NHS), women in the third tertile of dietary GI compared with those in the first tertile had an approximately 2·7-fold greater risk for early AMD, mainly pigment abnormalities (p = 0·01). Contrarily, dietary carbohydrate intake was not significantly associated(Reference Chiu, Morris and Hubbard184).
Similarly, in a study by Chiu et al., eyes in the fourth and fifth quintiles of dietary GI had a significantly increased risk of drusen, geographic atrophy and neovascularisation compared with the eyes in the first quartile of dietary GI. Moreover, a clear association between dietary GI and the severity of AMD was observed (p = 0·001)(Reference Chiu, Milton and Gensler185). In a 10-year follow-up study by Kaushik et al., higher mean dietary GI was linked to a higher risk of developing early AMD in a comparison of quartiles 1 and 4 after adjustments for age, smoking, other risk factors and dietary components (relative risk (RR): 1·77, 95% CI: 1·13, 2·78, p = 0·03), but the authors did not find a significant relationship between GI and late AMD(Reference Kaushik, Wang and Flood186). A recent case–control study showed a significant, direct correlation between daily GI, glycaemic load and insulin load on cataract risk(Reference Movahedian, Thomas and Rahmani187). Animal studies also report the relationship between a high-GI diet and AMD(Reference Rowan, Jiang and Chang188,Reference Rowan, Jiang and Korem189) .
Based on available evidence, a higher dietary GI is believed to be associated with an increased risk of early AMD, the severity of AMD, and specific conditions linked to AMD, such as atrophy, neovascularisation and drusen. Therefore, in formulating dietary recommendations, avoiding high-GI foods may be considered among potential dietary strategies.
Dietary fibres
Dietary fibres (DFs) are carbohydrate polymers with three or more monomeric units(Reference Champ, Langkilde and Brouns190). DF is classified into two types based on its solubility in water: soluble DF and insoluble DF. Soluble DF is the edible part of the plant that is resistant to digestion but can be partially or entirely fermented into short-chain fatty acids in the large intestine by colonic bacteria. Meanwhile, insoluble DF passes unchanged through the digestive tract. While cellulose, some hemicellulose and lignin are examples of insoluble DF, pectin, gums, mucilage extracted from psyllium husk, glucan and fructans, as well as some hemicellulose, are examples of soluble DF(Reference Soliman191). One of the mechanisms explaining the relationship between DF intake and AMD is the gut microbiota. Dietary fibres not broken down by the body’s enzymes in the upper gut are turned into short-chain fatty acids (SCFAs) by the microbiota in the cecum and colon. The main microbial fermentation products in the gut are acetate, propionate and butyrate(Reference Macfarlane and Macfarlane192). Aside from the physiological effects of SCFAs, the gut microbiome has been shown to influence multiple pathways involved in eye diseases. Increased gut permeability allows microbial products to be transported to the eye, where they can bind to receptors and signals ocular inflammation(Reference Andriessen, Wilson and Mawambo193).
Furthermore, microbial metabolites have the potential to cross the blood–retina barrier and act as signalling molecules in the eye. Multiple molecules produced in the gut, including serotonin, have been linked to the severity of eye disease(Reference Rowan, Jiang and Korem189). In a study conducted by Agrón et al. with AREDS and AREDS2, a significant relationship was found between the risk of progression to large drusen and insoluble DF intake of the first to fourth and first to fifth quartiles (p = 0·011 and p = 0·009, respectively). In contrast, no significant relationship was found with soluble DF(Reference Agrón, Mares and Clemons143). Another study found no significant relationship between total DF intake and early AMD or its component lesions. However, cereal fibre consumption reduced the long-term risk of early AMD(Reference Kaushik, Wang and Flood186). In the case–control study of Tinkılıç et al., DF intake was lower in the advanced AMD group than in the control group (p = 0·006)(Reference Tınkılıç, Aksoydan and Çıtırık194). Data from the Beaver Dam Eye Study also suggest that a high DF intake may be protective against nuclear cataracts(Reference Mares-Perlman, Brady and Klein195) in contrast to the Blue Mountains Eye Study results of 5-year indecent cataracts(Reference Townend, Townend and Flood196).
The role of intestinal microbiota is considered crucial in understanding the connection between fibres and AMD. Metabolites generated by the microbiota are believed to influence pathways relevant to eye diseases. Further discussions on the relationship between intestinal microbiota and AMD, along with dietary strategies, will be explored in the following sections.
Phytochemicals
The use of particular nutrients and antioxidant supplements has decreased the possibility of progression from early AMD to late AMD in a comprehensive evaluation of trials published in the past 35 years(Reference Pameijer, Heus and Damen197). In addition, researchers are currently investigating the preventive and therapeutic roles of various phytochemicals, including carotenoids, flavonoids, polyphenols and anthocyanidins in the context of AMD(Reference Bosch-Morell, Villagrasa and Ortega198). The protective effects of phytochemicals are attributed to several mechanisms, such as expanding and reducing reactive oxygen species, inhibiting factor-α and endothelial growth factor pathways, suppressing apoptosis and modulating inflammatory markers(Reference Bungau, Abdel-Daim and Tit199).
Carotenoids
Carotenoids are natural pigments in numerous fruits and vegetables, lending to their yellow, orange and red hues. They can be classified into two groups: xanthophylls (lutein, zeaxanthin and meso-zeaxanthin) and carotenes (α-carotene, β-carotene and lycopene)(Reference Lem, Gierhart and Davey200). Carotenoids are potent antioxidants that reduce oxidative stress in the macula and shield the RPE and choroid from light damage(Reference Manikandan, Thiagarajan and Goutham201). Higher dietary β-carotene, lutein and zeaxanthin consumption are linked to a reduced risk of AMD progression(Reference Agrón, Mares and Clemons143). Higher plasma lutein levels were associated with a 37% reduced incidence of advanced AMD in the Alienor study, which included participants aged 73 or older(Reference Merle, Rosner and Seddon202). Further details about the role of carotenoids can be found in this manuscript’s ‘Vitamin A and carotenoids’ section.
Polyphenols
Dietary polyphenols represent many phytochemicals in fruits, vegetables, cacao powder, red wine, green tea and coffee. They possess antioxidant, anti-inflammatory, anti-allergic, antimicrobial and antiviral properties, contributing to overall health benefits(Reference Bungau, Abdel-Daim and Tit199). One of the polyphenols’ prominent effects on health is its effects on vision. In addition, polyphenols help reduce oxidative stress, provide anti-inflammatory effects in RPE cells, and modulate interleukins and signalling pathways(Reference Pawlowska, Szczepanska and Koskela203). A study conducted with elderly Korean women from the Korea National Health and Nutrition Examination Survey (2010–2012) revealed that a higher dietary flavonol content was protective against the development of AMD(Reference Kim, Kim and Kwon204).
Studies on the therapeutic use of resveratrol, a polyphenol found in various foods such as blueberries, cocoa, peanuts, wine and grape juice(Reference Baur and Sinclair205), have been growing in recent years(Reference Abu-Amero, Kondkar and Chalam206–Reference Richer, Patel and Sockanathan209). Experimental studies have indicated that resveratrol may effectively target various pathways involved in the pathogenesis of age-related eye diseases, such as oxidative stress, inflammation, mitochondrial dysfunction, apoptosis or angiogenesis(Reference Abu-Amero, Kondkar and Chalam206). In a study by King et al., it was observed that resveratrol (50 and 100 µmol/l) inhibited intracellular oxidation and protected RPE cells from apoptosis. Therefore, it has been reported that resveratrol can decrease oxidative stress and hyperproliferation of RPE. This protective effect of resveratrol is attributed to its ability to inhibit the mitogen-activated protein kinase (MAPK) signalling cascade and the activities of extracellular signal-regulated kinases 1 and 2 (ERK 1/2)(Reference King, Kent and Bomser210). In addition, sirtuin-1 (SIRT1) activation by resveratrol has ameliorated hydrogen peroxide-induced damage to lens epithelial cell morphology, promoting cell proliferation and inhibiting apoptosis(Reference Zheng and Lu211). In the case report of three patients treated with oral resveratrol (100 mg), extensive bilateral ocular structure and function improvements were observed over a long time(Reference Richer, Patel and Sockanathan209). Resveratrol treatment prevented increased intracellular reactive oxygen species and inflammatory marker production, lipofuscin expression and accumulation of carbonylated proteins(Reference Luna, Li and Liton212). It is emphasised that resveratrol can achieve this effect by reconstructing the retinal structure, reducing the accumulation of lipofuscin, increasing choroidal thickness, shortening the recovery period of glare, enlarging the macular pigment volume, and improving both contrast sensitivity and visual function(Reference Bosch-Morell, Villagrasa and Ortega198).
Furthermore, resveratrol has been reported to exert a protective effect against light-induced retinal degeneration by reversing the activation of retinal activating protein-1 and inhibiting light-induced up-regulation of SIRT1 activation(Reference Kubota, Kurihara and Ebinuma213) in addition to protecting RPE cells from apoptosis induced by autoimmune antibodies(Reference Anekonda and Adamus214). Resveratrol contributes to the antioxidant defence system by increasing superoxide dismutase, glutathione peroxidase and catalase activities against hydrogen peroxide-induced cytotoxicity in human retinal D407 RPE cells(Reference Pintea, Rugină and Pop215). In addition, resveratrol suppresses the secretion of inflammatory cytokines, transforming growth factor β (TGF-β) and hypoxia-enhanced VEGF-A and VEGF-C secretion by human RPE cells. SIRT1, a significant modulator of resveratrol, suppresses hypoxia-induced factor-1 signalling, leading to decreased VEGF-A secretion(Reference Chung, Yao and Caito216). In human crystal epithelial cells, resveratrol also lessens the accumulation of intracellular reactive oxygen species (ROS) caused by hydrogen peroxide. It is emphasised that, due to its ability to restore vessels, resveratrol may also be beneficial for the microcirculation of the eye(Reference Bosch-Morell, Villagrasa and Ortega198). Furthermore, resveratrol has been shown to protect RPE cells against acrolein-induced oxidative cytotoxicity by increasing mitochondrial bioenergy(Reference Sheu, Liu and Ou208).
A cohort study investigating the relationship between total dietary flavonoids and AMD found an independent and protective association between dietary flavonoid intake and the probability of developing AMD(Reference Gopinath, Liew and Kifley217). Anthocyanins in blue, purple and red fruits, vegetables and flowers are water-soluble pigments belonging to the phenolic group and exhibit significant antioxidant and antimicrobial properties(Reference Khoo, Ng and Yap86,Reference Sin, Liu and Lam218) . Anthocyanins are thought to be important for visual health due to their antioxidant effects. Their effects on visual health include improving visual function in patients with normal tension glaucoma, preserving photoreceptor cell function during retinal inflammation, reducing lens opacity accompanied by lower malondialdehyde (MDA) levels, suppressing cell death of lens epithelial cell line under oxidative stress induced by hydrogen peroxide, preventing retinal degeneration induced by N-methyl-N-nitrosourea, increasing ocular blood flow(Reference Khoo, Azlan and Tang219). In a study where anthocyanins from black soybean seed pods were taken by mouth for 4 weeks, it was found that they could protect retinal neurons from structural and functional damage caused by N-methyl-N-nitrosourea(Reference Paik, Jeong and Jung220). In addition, anthocyanins provided resistance to membrane permeability induced by the detergent-like effects of pyridinium bis-retinoid, light-induced damage to the cell on RPE cells(Reference Jang, Zhou and Nakanishi221).
Quercetin, another flavonoid found in fruits and vegetables that gives them their colour, is a powerful antioxidant commonly found in foods such as apples, onions and tea(Reference Wang, Zhao and Wang222). The study conducted by Cao et al. showed that quercetin altered the transcription of genes involved in the regulation of apoptosis in RPE cells exposed to oxidative stress induced by hydrogen peroxide(Reference Cao, Liu and Tuo223). In a rabbit study investigating the effect of quercetin on light-induced retinal damage, it was observed that quercetin effectively suppressed increased levels of oxidative stress markers and inhibited the production of inflammatory cytokines in the retina. Quercetin also regulated changes in the expression levels of apoptotic proteins and angiogenesis factors induced by photooxidative stress(Reference Wang, Zhao and Wang222). Furthermore, it modulates the activity of antioxidant enzymes, including phase II enzymes(Reference Pawlowska, Szczepanska and Koskela203). The protective effect of quercetin in the retina comes from its ability to directly remove ROS and its indirect anti-inflammatory, anti-apoptotic and antiangiogenic effects, as well as its ability to reduce A2E photooxidation, lower methylglyoxal levels and stop the activation of enhanced end glycation product receptors(Reference Lashay, Sadough and Ashrafi224). In addition, quercetin appears to inhibit laser irradiation-induced choroidal neovascularisation and increase choroidal blood flow(Reference Bosch-Morell, Villagrasa and Ortega198).
The beneficial effects of polyphenols on the lens of the eye can be summarised as inhibition of several inflammatory mediators, regeneration of rhodopsin, scavenging of reactive oxygen species, reduction of the formation of advanced glycation end products, inhibition of aldose reductase and enhancement of ocular blood flow(Reference Bosch-Morell, Villagrasa and Ortega198). Figure 3 summarises the mechanisms by which phytochemicals are thought to be involved in preventing AMD development. Saffron(Reference Lashay, Sadough and Ashrafi224,Reference Di Marco, Carnicelli and Franceschini225) , ginkgo(Reference Spadiene, Savickiene and Jurgeviciene226), blueberry and bilberry(Reference Huang, Wu and Li227,Reference Osada, Okamoto and Kawashima228) , goji berry(Reference Li, Holt and Keen229), and curcuma or turmeric(Reference Park, Lee and Kim230,Reference Jitsanong, Khanobdee and Piyachaturawat231) are among the herbal products most extensively studied about macular degeneration. The effectiveness of these plants may be attributed to their phytochemical constituents(Reference Bosch-Morell, Villagrasa and Ortega198). Consumption of foods rich in these phytochemicals may offer protection against AMD; however, further research is needed to establish more substantial evidence. Phytochemicals stand out for their potential preventive and therapeutic roles in AMD by reducing oxidative stress, inflammation and apoptosis. Although these phytochemicals protect against AMD and have promising therapeutic effects, more research is needed for more robust evidence. However, based on the data we have, it is clear that adding foods rich in phytochemicals to the diet and ensuring nutritional diversity is very important for eye health.

Fig. 3. The components of the Mediterranean diet and possible effects in AMD prevention/progression.
Biotics
The gut microbiome plays a substantial role in human health by influencing the development of various chronic diseases, including gastrointestinal disorders and metabolic conditions(Reference Hills, Pontefract and Mishcon232). The presence of diverse gut bacteria has beneficial effects on health by producing short-chain fatty acids, essential in maintaining lipid homeostasis and reducing inflammation through the fermentation of dietary fibre(Reference Hills, Pontefract and Mishcon232). Recent evidence suggests a connection between the gut and the eyes, known as the gut–eye axis, where an imbalance in gut microbiota, known as gut dysbiosis, may contribute to the onset and progression of AMD(Reference Napolitano, Filippelli and Davinelli233). Differences in taxonomic and functional characteristics of the human microbiome are reported between people with AMD and healthy controls(Reference Zisimopoulos, Klavdianou and Theodossiadis234). This emerging field of research indicates that the gut microbiome could significantly impact the development and management of AMD. In an AMD mouse model, a high-fat diet worsened laser-induced choroidal neovascularisation and led to alterations in the composition of the gastrointestinal microbiome(Reference Andriessen, Wilson and Mawambo235). Mice on a high-fat diet showed a higher prevalence of Firmicutes and Proteobacteria, while those on a regular diet had a higher prevalence of Bacteroidetes.
Similarly, another study in mice compared a high-GI diet and a low-GI diet and found that the high GI diet-induced AMD symptoms in the retina while switching to the low-GI diet either halted or reversed these symptoms(Reference Rowan, Jiang and Korem189). In addition, the high-GI diet was associated with abundant Firmicutes and Proteobacteria, specifically within the Clostridia and Bacilli classes. In contrast, the low-GI diet promoted a higher prevalence of Bacteroidetes and Erysipelotrichia(Reference Rowan, Jiang and Korem189,Reference Rowan and Taylor236) . In studies investigating the association between the gut microbiome and AMD in humans, specific microbial changes have been observed in patients with AMD compared with controls. The genera Anaerotruncus and Oscillibacter and the species Ruminococcus torques and Eubacterium ventricose were found to be more abundant in patients with AMD. At the phylum level, patients with AMD had a higher relative abundance of Firmicutes than Bacteroidetes(Reference Zinkernagel, Zysset-Burri and Keller237). At the phylum level, patients with AMD had a higher relative abundance of Firmicutes compared with Bacteroidetes(Reference Zinkernagel, Zysset-Burri and Keller237). Another study identified that the genus Oscillibacter and species Bacteroides were more prevalent in healthy controls, whereas the Negativicutes class was more abundant in patients with AMD. Negativicutes showed the highest potential as a possible biomarker for detecting AMD in patients(Reference Zysset-Burri, Keller and Berger238).
Metabolic processes have also been found to differ in patients with AMD. In the gut microbiota of patients with AMD, genes related to the fatty acid elongation pathway were down-regulated. In contrast, genes related to l-alanine fermentation, glutamate degradation and arginine biosynthesis were up-regulated(Reference Zinkernagel, Zysset-Burri and Keller237). Depleting glutamate, an excitatory neurotransmitter for the retina and brain, may lead to insufficient neurotransmission in the retina, which could explain the connection between increased glutamate breakdown and patients with AMD (Reference Bui, Hu and Acosta239). Additionally, higher ornithine levels have been observed in patients with choroid and retinal atrophy due to decreased ornithine-ketoacid aminotransferase activity. This suggests that arginine may play a crucial role in the development of retinal degeneration(Reference Zysset-Burri, Morandi and Herzog240). A mice study found that low GI diets reduced the accumulation of C3-carnitine in the retina, plasma or urine, as well as long-chain polyunsaturated lipids and their peroxidation end products(Reference Rowan, Jiang and Korem189). Serotonin, particularly from microbial cometabolites, is suggested to have anti-AMD potential(Reference Rowan, Jiang and Korem189).
Additionally, a positive correlation between harmful inflammation and CFH in a study suggests that the gut microbiota may contribute to the pathogenesis of AMD through the dysregulated regulation of the complement system(Reference Zysset-Burri, Keller and Berger238). These findings highlight the potential role of specific gut microbial compositions and metabolic pathways in the pathogenesis of AMD. Further research is needed to understand the mechanisms better and explore the therapeutic potential of modulating the gut microbiome for AMD prevention and treatment.
Recent research has shed light on the concept of an intestinal–eye axis, where intestinal dysbiosis plays a significant role in the development and progression of macular degeneration(Reference Napolitano, Filippelli and Davinelli233). Dietary factors can influence the composition of the gut microbiota, which regulates the immune system and inflammation(Reference Singh, Chang and Yan241). Furthermore, the gut microbiota may have an indirect impact on ocular health through its influence on insulin sensitivity and obesity(Reference Francisco, Smith and Aragones242). Bacterial translocation from the gut to the eye is one possible mechanism for the relationship between the gut microbiota and AMD(Reference Lin243). Andriessen et al. (2016) state that intestinal dysbiosis can lead to increased intestinal permeability and chronic low-grade inflammation. This dysbiosis can result in elevated production of pro-inflammatory cytokines such as IL-6, IL-1, TNF and VEGF, exacerbating AMD pathological angiogenesis(Reference Zisimopoulos, Klavdianou and Theodossiadis234). Intestinal dysbiosis can also impair immune responses and disrupt mucosal barrier function, allowing pathogenic microbes and their metabolites to enter the bloodstream. This bacterial translocation can induce local and systemic inflammation, potentially contributing to the development of AMD(Reference Floyd and Grant244). However, it is still unclear whether mucosal inflammation is the cause or consequence of dysbiosis(Reference Zysset-Burri, Morandi and Herzog240). The potential of microbial substances to translocate through the intestinal wall and bind to receptors in the eye to signal ocular inflammation is increased(Reference Andriessen, Wilson and Mawambo235,Reference Floyd and Grant244) . Mice with intestinal dysbiosis induced by a high-fat diet had a twofold increase in microglia and macrophages within CNV lesions(Reference Andriessen, Wilson and Mawambo235). Researchers did high-throughput RNA sequencing on germ-free (GF) mice to look at the changes in gene expression caused by a high-fat diet (HFD) in the RPE and choroid. The study revealed that HFDs could lead to transcriptional alterations in the RPE and choroid tissue, even without gut microbiota. A total of 649 differentially expressed genes (DEGs) were identified in the HFD group, with 616 genes showing up-regulation and 33 genes showing down-regulation at the transcriptional level. Through Gene Ontology (GO) analysis of the DEGs, HFD induced up-regulation of angiogenesis, the immune response and inflammatory processes. Furthermore, ECM binding, ECM structural components and heparin binding were altered(Reference Xiao, Xie and Dao245).
In the same way that they can impact the bioavailability of numerous micronutrients, such as folic acid, vitamin B12 and iron, gut microorganisms can also affect the bioavailability of carotenoids and zinc, which are protective against AMD(Reference Zysset-Burri, Morandi and Herzog240). These findings suggest that the gut microbiota and its metabolites, including those impacted by SCFAs, can influence the development and progression of AMD by modulating intestinal permeability, inflammation and nutrient bioavailability. SCFAs may positively affect AMD by increasing intestinal epithelial integrity and reducing systemic inflammation(Reference Zysset-Burri, Morandi and Herzog240)
Modulating the human gut microbiota is a promising strategy for promoting overall health and preventing and treating various diseases. Indeed, the modulation of the gut microbiota through dietary adjustments or probiotic intake holds potential as a treatment option in conditions with an inflammatory component like AMD. These approaches aim to maintain immunological homeostasis, promote the production of beneficial metabolites by gut bacteria, and reduce complement activation(Reference Zisimopoulos, Klavdianou and Theodossiadis234). Various studies have demonstrated that specific dietary fibres can significantly affect the human gut microbiota’s abundance, diversity and metabolism(Reference Jefferson and Adolphus246–Reference Loo, Howell and Chan249). The systematic review of human intervention studies on the effects of intact cereal fibres and their active subfractions on gut microbiota composition in healthy adults included forty-two studies(Reference Jefferson and Adolphus246). Thirty-nine studies reported increased microbiota diversity and/or abundance after consuming intact cereal fibre. Based on the findings, it has been determined that an increase of 6–8 g in wheat fibre intake can significantly affect the gut microbiota(Reference Jefferson and Adolphus246,Reference Hervert Hernández250) . One of the proposed mechanisms underlying the protective effects of prebiotic cereal dietary fibres is their fermentation by the colonic microbiota. During this fermentation process, the gut microbiota produces several metabolic byproducts, including acetate, propionate and butyrate, which are SCFAs. The addition of different grains to the diet has been found to have varying effects on the concentrations of acetate, butyrate and propionate. When considering acetate, its concentration tends to increase with the consumption of various grains. As for butyrate, its concentration specifically increases with the addition of rice and oats, and similarly, propionate concentrations tend to increase with the addition of wheat or sorghum to the diet(Reference Gamage, Tetu and Chong247). These results show that different grains can have different effects on the production of SCFAs by the gut microbiota and that the changes in the concentrations of acetate, butyrate and propionate depend on which grains are eaten. Overall, the fermentation of the prebiotic effects of cereal dietary fibres by the colonic microbiota and the production of SCFAs contribute to the anti-inflammatory effects and modulation of glucose and lipid metabolism, thereby playing a role in the promotion of gut health and overall wellbeing.
The role of omega-3 fatty acids is essential in modulating the gut microbiota. The gut microbiota, omega-3 fatty acids and host immune cells work together to maintain the integrity of the gut wall(Reference Parolini251). Clinical studies have shown that omega-3 fatty acids can reduce the Firmicutes/Bacteroidetes ratio and decrease the levels of Coprococcus and Faecalibacterium. Also, Bifidobacterium, Lachnospira, Roseburia and Lactobacillus, which are types of bacteria that make butyrate, are more common when there are more omega-3 fatty acids(Reference Andersen, Mølbak and Michaelsen252). Furthermore, omega-3 PUFAs can reduce the Firmicutes/Bacteroidetes ratio and decrease the levels of Coprococcus and Faecalibacterium. Also, omega-3 fatty acids have been linked to a rise in the number of bacteria that make butyrate, such as Bifidobacterium, Lachnospira, Roseburia and Lactobacillus. This suggests that omega-3 PUFAs promote the growth and activity of butyrate-producing bacteria, which are essential for gut health. Additionally, a significant correlation was observed between plasma levels of omega-3 PUFAs and the Lachnospiraceae family, known for its ability to produce SCFAs such as butyrate(Reference Andersen, Mølbak and Michaelsen252). These findings highlight the interaction between omega-3 PUFAs, the gut microbiota and SCFA production, emphasising the importance of a balanced gut microbial community and the potential role of omega-3 PUFAs in promoting gut health and overall wellbeing.
Probiotics are one of the issues addressed to ensure intestinal microbiota modulation. In a randomised, double-blind, placebo-controlled study, participants aged between 50 and 85 years with AMD took either a probiotic capsule or a placebo daily for 8 weeks(Reference Farajipour, Sadr and Matin253). The results showed that probiotic supplementation led to increased plasma total antioxidant capacity and HDL-cholesterol levels while reducing malondialdehyde levels, indicating a decrease in oxidative stress and improved antioxidant status. However, no significant effects were observed on other clinical indicators or metabolic profiles(Reference Morita, Miwa and Jounai254). In addition, specific strains of lactic acid bacteria, such as Lactobacillus paracasei KW3110, have shown promise in reducing inflammation and protecting against ocular diseases. In both mouse and human studies, L. paracasei KW3110 has been found to activate macrophages that reduce inflammation(Reference Choi, Kim and Lee255). In addition, taking L. paracasei KW3110 was linked to less inflammation and better activation of M2 macrophages in a study on people with eye diseases caused by visual display terminal (VDT) load.
Additionally, in a mouse model of light-induced retinopathy, L. paracasei KW3110 supplementation decreased inflammation and protected against photoreceptor degradation, suggesting its potential for protection against retinal disorders(Reference Morita, Miwa and Jounai254). These results suggest that taking probiotics, especially certain strains like L. paracasei KW3110, may help protect against inflammation and oxidative stress in AMD and other retinal diseases that are similar to it. However, further research is needed to understand the mechanisms and establish optimal treatment protocols fully. These results suggest that taking probiotics, especially certain strains like L. paracasei KW3110, may help protect against inflammation and oxidative stress in AMD and other retinal diseases that are similar to it. However, further research is needed to fully understand the mechanisms and establish optimal treatment protocols(Reference Choi, Kim and Lee255).
The association between intestinal microbiota imbalance (dysbiosis) and the initiation and progression of age-related macular degeneration (AMD) takes centre stage, introducing the concept of the gut–eye axis. Studies reveal alterations in the composition of intestinal microbiota and metabolic processes among individuals with AMD. Promising developments in AMD treatment include dietary adjustments (such as increasing omega-3 fatty acids and reducing the glycaemic index), incorporating prebiotics and probiotics, and modulating the intestinal microbiota through postbiotics. To better understand the role of the microbiome and the potential benefits of probiotics, prebiotics and postbiotics in AMD, further randomised clinical trials with larger sample sizes are needed. These studies would help to assess the effectiveness and safety of interventions that target the gut microbiome in patients with AMD. Conducting more research to unravel the specific mechanisms through which gut dysbiosis influences AMD is essential. This will provide insights into the underlying biological processes and help identify therapeutic strategies that directly target the gut microbiome to prevent and treat AMD. Continued investigation in this area holds great potential for advancing our understanding and management of this complex eye condition.
Dietary patterns
In the preceding sections of our manuscript, we discussed individual nutrients’ effectiveness on AMD. However, assessing the overall diet in conjunction with specific nutritional elements is crucial. Previous studies primarily focused on specific foods, food groups and nutrient intake using data from food frequency questionnaires or food consumption records. More recent studies have also examined general dietary patterns(Reference Hogg and Woodside256). This concept emphasises that foods supply essential nutrients to the body and contain numerous compounds that interact synergistically(Reference Gastaldello, Giampieri and Quiles257). These interactions often occur when these molecules are present in their natural matrices. More than merely assessing individual nutrients or components is required to understand the impact of a diet on health. When evaluating the impact of diet on health and disease, it is essential to consider all dietary patterns rather than focusing solely on certain food groups or nutrients(Reference Hogg, Woodside and McGrath258). Diet is a multifaceted lifestyle behaviour that influences the consumption of particular foods due to cultural, geographic and economic factors(Reference Hogg, Woodside and McGrath258). Furthermore, while exogenous supplementation may appear practical, adherence to a healthy diet is crucial. This suggests that the risk of AMD can be modified through diet alone without exogenous supplementation(Reference Raimundo, Mira and Cachulo259).
Numerous studies have examined the relationship between diet and AMD(Reference Merle, Rosner and Seddon202,Reference Hogg, Woodside and McGrath258,Reference Kang and Kim260,Reference Sasaki, Miyagawa and Harada261) . Evaluation of baseline AREDS dietary data revealed two main components, which the researchers referred to as Oriental and Western patterns. A notable intake of vegetables, legumes, fruits, whole grains, tomatoes and seafood distinguished the Oriental dietary pattern. In contrast, the Western dietary pattern was characterised by an elevated consumption of red meat, processed meat, high-fat dairy products, French fries, refined grains and eggs(Reference Chiu, Chang and Li262). As a result, the Oriental pattern has been linked with considerably lower early and advanced AMD rates.
In contrast, the Western pattern was related to significantly higher rates of early and advanced AMD. According to the Melbourne Collaborative Cohort Study, a diet high in grains, fish, chicken, vegetables and nuts and low in red meat has been linked to a lower prevalence of late AMD(Reference Amirul Islam, Chong and Hodge263). The Coimbra Eye Study and the Korea National Health and Nutrition Examination Survey found that higher vegetable, fruit and nut consumption was associated with a statistically significant lower risk of developing AMD(Reference Nunes, Alves and Barreto264,Reference Kim, Kim and Vijayakumar265) . This correlation is likely because fruits and vegetables, particularly dark-green leafy varieties, are rich in carotenoids (e.g. lutein and zeaxanthin), and nuts are rich in fatty acids(Reference Tang, Mitchell and Flood266). These nutrients support a robust immune system, reduce oxidative stress and prevent retinal inflammation. However, in the Atherosclerosis Risk in Communities (ARIC) study, no significant association was observed between dietary patterns and any incident or early AMD events. However, it was reported that the Western pattern may be a risk factor for the development of late AMD(Reference Dighe, Zhao and Steffen267). Similar to this study, the Irish Nun Eye Study observed no association between a diet model and the risk of AMD(Reference McCarter, Neville and Silvestri268). The complexity of DP composition, geographic variations in dietary intake, genetic factors and AMD definitions can be confounding factors limiting the association between diet and AMD outcomes. The low prevalence of AMD in the Irish Nun Eye study population, their relatively good health for their age, and their restricted dietary intake may have limited the statistical power to detect an association between diet and AMD(Reference McCarter, Neville and Silvestri268). The presence of certain factors, such as variations in geographic dietary patterns, genetic variations, and differences in how AMD is classified, could introduce confusion and limit the ability to detect clear associations with dietary outcomes. Furthermore, the study’s authors indicated that the relatively low prevalence of AMD in the study population might not be attributed to AMD itself but rather to the participants’ overall good health and adherence to a restricted diet. It is worth noting that the study’s statistical power may be limited in detecting specific associations(Reference McCarter, Neville and Silvestri268).
Advanced AMD is linked to total diet quality, according to a study using the Healthy Eating Index (HEI) and Alternative Healthy Eating Index (AHEI)(Reference Montgomery, Kamel and Pericak-Vance269). In case–control research examining the link with advanced AMD, HEI, used to analyse the relationship of diet with health, was found to be protective(Reference Montgomery, Kamel and Pericak-Vance269). The components of trans fat intake and the proportion of polyunsaturated to saturated fats are AMD-protecting characteristics of alternative HEI. In the Carotenoids in Age-Related Eye Disease Study, the Healthy Eating Index (HEI) 2005 score was calculated to assess dietary quality. It was observed that individuals in the highest quintile of the index had a 46% lower risk of developing early AMD compared with those in the lowest quintile(Reference Merle, Colijn and Cougnard-Grégoire270).
The Mediterranean diet stands out among the discussed dietary models due to its well-documented health benefits. This dietary pattern is characterised by high consumption of plant-based foods, moderate intake of fish and wine, limited consumption of dairy products and meat, and the use of monounsaturated fatty acids (MUFAs), such as olive oil, as the primary source of fat(Reference Merle, Silver and Rosner271). Multiple studies have shown that greater adherence to the Mediterranean diet is associated with a decreased risk of developing AMD and a lower likelihood of disease progression(Reference Hogg and Woodside256–Reference Hogg, Woodside and McGrath258,Reference Nunes, Alves and Barreto264) . In addition, a comprehensive analysis of eight human observational studies conducted in 2022 confirmed that increased adherence to a Mediterranean eating pattern is linked to a decreased risk of acquiring AMD and experiencing disease progression(Reference Gastaldello, Giampieri and Quiles257).
Similarly, a systematic review published in 2022 summarised research spanning 35 years and found moderately specific evidence supporting the association between adherence to a Mediterranean diet and a decreased risk of progressing from early to late AMD(Reference Pameijer, Heus and Damen197). The Coimbra Eye Study conducted in Portugal also reported that high adherence to the Mediterranean diet was protective against the development of AMD, with fruit consumption specifically identified as having a potential protective role(Reference Nunes, Alves and Barreto264). Figure 3 shows the components of the Mediterranean diet and their possible roles in AMD prevention and progression.
There are indeed interactions between genes and nutrients in AMD. The Mediterranean diet is associated with a reduced risk of late AMD among individuals with protective alleles of the CFH gene(Reference Keenan, Agron and Mares272). The CFH gene is one of the essential genes implicated in AMD. Higher adherence to the Mediterranean diet has been associated with a decreased risk of developing advanced AMD, and genetic susceptibility may play a role in this association(Reference Merle, Silver and Rosner271). In the AREDS study, when accounting for various factors, a high alternate Mediterranean Diet (aMeDi) score was significantly associated with a decreased risk of progressing to advanced AMD. However, this association was not observed in individuals with homozygous risk alleles ©(Reference Merle, Silver and Rosner271).
On the other hand, people with the complement factor H Y402H non-risk (T) allele had a significantly lower risk of getting advanced AMD if they had a higher aMedi score(Reference Merle, Silver and Rosner271). According to the European Eye Study, following the Mediterranean diet reduced the risk of wet AMD, regardless of the presence of the CFH risk allele. At the same time, no significant correlation was observed with early AMD(Reference Hogg, Woodside and McGrath258). It should be noted that the AREDS study initially included individuals with intermediate AMD, which may have an overrepresentation of the CFH risk allele, and the presence of the CFH protective allele in individuals without the CFH risk allele could be a factor contributing to these findings(Reference Keenan, Agron and Mares272). Using data from the Age-Related Eye Disease database, researchers looked at how the size of drusen changed over time and how it related to genetic susceptibility and following an alternative Mediterranean diet. They found that genetic susceptibility was linked to drusen growth on its own. Additionally, it has been reported that moderate to high adherence to the alternative Mediterranean diet score tends to reduce the risk of drusen growth(Reference Merle, Rosner and Seddon202).
Additionally, the precise mechanism by which the Mediterranean diet influences AMD still needs to be fully understood(Reference Hogg and Woodside256). It is challenging to determine whether the effect is directly attributable to the specific foods included in the diet, indirectly attributed to a reduction in the consumption of unhealthy foods, or a combination of both. Cross-sectional and prospective studies conducted in population-based and clinical cohorts, as well as in different geographic regions, have consistently shown that a diet high in fruits, vegetables, legumes and fish while avoiding unhealthy foods like red meat and moderate alcohol intake may help prevent AMD(Reference Hogg and Woodside256). The protective effects of the Mediterranean diet against the pathogenesis and progression of AMD are attributed to its rich antioxidant and anti-inflammatory components. It is logical to assume that the Mediterranean diet emphasises the consumption of food groups high in vitamins C and E and rich in antioxidants like lutein and zeaxanthin(Reference Gastaldello, Giampieri and Quiles257). Carotenoids exhibit a protective effect against AMD in the retina through two mechanisms. Firstly, it acts as a blue light filter, absorbing harmful blue light and reducing the risk of light-induced damage. Secondly, lutein functions as an antioxidant, counteracting free radicals and reactive oxygen species, thereby reducing oxidative stress and protecting the retina from damage(Reference Merle, Cougnard-Grégoire and Korobelnik273)
The potential preventive effect of the Mediterranean diet on AMD is likely mediated through the consumption of vegetables, fruits and nuts, as these food groups provide significant levels of antioxidants(Reference Nunes, Alves and Barreto264). In addition to vitamins and carotenoids, various fruits and vegetables also contain phytochemicals that may contribute to the observed associations between dietary intake of these nutrients and a lower prevalence of AMD(Reference Keenan, Agron and Mares272). Furthermore, it is now widely recognised that certain vegetables and diets high in fruits and vegetables promote a healthy gut microbiome(Reference Keenan, Agron and Mares272). Higher adherence to the Mediterranean diet has been associated with specific changes in the gut microbiota composition(Reference Loo, Howell and Chan274). Individuals with greater adherence to the Mediterranean diet have been shown to have higher SCFA levels and an increased abundance of Bacteroidetes. Conversely, reduced adherence to the Mediterranean diet has been related to an elevated ratio of Firmicutes to Bacteroidetes (F/B ratio)(Reference Loo, Howell and Chan274). An imbalance in the F/B ratio has been implicated in various health conditions, including obesity and metabolic disorders. Also, a Mediterranean diet with more nuts and legumes has been shown to boost the growth of good bacteria, which is linked to positive changes in the way the gut microbiome works, including more short-chain fatty acid production, less secondary bile acids and fewer inflammation markers(Reference Loo, Howell and Chan274). In addition, a study on the Korean population revealed that a diet rich in legumes may protect against age-related macular degeneration(Reference Kang and Kim260). These results suggest that the Mediterranean diet, which focuses on plant-based foods, whole grains, legumes and olive oil, may help create a healthier gut microbiota by increasing SCFA production and keeping the number of Firmicutes to Bacteroidetes healthy.
The positive effects of the Mediterranean diet on health can be attributed in part to the great use of olive oil used in this diet. Olive oil is a rich MUFA source and contains polyphenols, which possess various beneficial properties such as antioxidant, anti-inflammatory, antithrombotic and antimicrobial properties(Reference Martín-Peláez, Covas and Fito275). The relationship between MUFA intake and AMD has yielded inconsistent findings, suggesting that the protective effects of olive oil may be attributed to its polyphenol content(Reference Cougnard-Gregoire, Merle and Korobelnik147). Olive oil contains soluble phenolic compounds like oleuropein, hydroxytyrosol, tyrosol, and oleocanthal, recognised for their antioxidant and anti-inflammatory properties(Reference Armento, Ueffing and Clark17). Nonetheless, the available evidence suggests that the presence of polyphenols in olive oil plays a significant role in its protective effects against AMD. In the Alienor study, which focused on the elderly population, the associations between olive oil use and the prevalence of AMD were examined. The results showed that regular olive oil use was significantly associated with a reduced risk of late AMD, but no significant association was found with early AMD. Further research is needed to understand better the specific mechanisms by which olive oil may impact AMD development and to explore its potential benefits in preventing the early stages of the disease(Reference Cougnard-Gregoire, Merle and Korobelnik147).
The consumption of fish, an excellent source of omega-3 fatty acids, is vital for the Mediterranean diet. omega-3 fatty acids have also been shown to play a significant role in this protective effect for AMD. The high content of omega-3 fatty acids in the retina may explain the association between fish consumption and AMD progression(Reference Kang and Kim260). Also, omega-3 fatty acids may be protective because higher levels of docosapentaenoic acid in the blood have been linked to a higher macular pigment optical density(Reference Merle, Buaud and Korobelnik276). In addition, a nationwide survey conducted in Korea found that more frequent fish consumption was associated with a lower risk of developing early AMD(Reference Kang and Kim260).
Other dietary patterns or scores, such as the dietary inflammatory index or GI, which could potentially be important for AMD, have not received as much attention as the Mediterranean diet due to the need for more detailed dietary data and new prospective cohort studies(Reference Hogg and Woodside256). However, some studies have explored the association between these dietary patterns and AMD. For example, according to the Tsuruoka Metabolomic Cohort Study findings, individuals who followed a variable staple diet characterised by a higher intake of bread and noodles but a lower intake of rice had a reduced prevalence of intermediate AMD(Reference Sasaki, Miyagawa and Harada261). Additionally, a high GI diet has been found to significantly increase the risk of AMD progression compared with a low-GI diet. Therefore, adopting a low-GI diet and reducing the consumption of refined carbohydrates may be advisable for preventing AMD(Reference Chiu, Milton and Klein277).
Calorie restriction (CR) is one of the dietary strategies being examined to prevent age-related functional decline, including ocular illnesses(Reference Francisco, Smith and Aragones242). A study with Brown Norway mice examined the effect of 40% energy restriction on retinal ageing. It was found that the group on a calorie-restricted diet had significantly higher outer nuclear layer (ONL) cell densities, ONL height and retinal thickness than the group on an ad libitum diet(Reference Obin, Pike and Halbleib278). In addition, CR was associated with higher peripheral INL cell density and thickness. Another study examining the effect of 40% CR on photoreceptor cell loss in Fischer 344 rodents found that the CR group lost more photoreceptor cells, especially in the retina’s centre. This suggests that the loss of photoreceptor cells is caused by light. Furthermore, the detrimental effect of intense light on photoreceptor cell density was more pronounced in the CR group. The authors hypothesise that calorie restriction may affect circadian activity, which could explain its apparent inability to delay retinal ageing in albino rats(Reference Obin, Halbleib and Lipman279). It has been suggested that the protective function of photoreceptors may vary among rat species(Reference Francisco, Smith and Aragones242). A study with male Wistar rats found that reducing the amount of food eaten or the number of calories in the food led to a significant decrease in the amount of RPE lipofuscin(Reference Katz, White and Gao280). Due to the challenges of implementing calorie restriction as a disease prevention or treatment strategy, intermittent fasting has been developed to mimic the benefits of calorie restriction with less stringent practices(Reference Francisco, Smith and Aragones242). Intermittent fasting is currently attracting much interest due to its ability to defend against several systemic diseases, such as eye, cardiovascular and even metabolic disorders(Reference Choi, Kim and Lee255). Every-other-day fasting (EODF) has been suppressing retinal degeneration without changing intraocular pressure in a glaucoma mouse model(Reference Guo, Kimura and Azuchi281). The improvement of visual impairment with EODF highlights its neuroprotective effect. This protective effect is related to the up-regulation of blood β-hydroxybutyrate levels and increased histone acetylation in the retina, which reduce oxidative stress levels. After rats were injured, dietary restriction helped their retinas improve by lowering oxidative stress and increasing the activity of an enzyme involved in oxidative phosphorylation(Reference Kong, van Bergen and Bui282). Only one human study evaluated the association of intermittent fasting with AMD. According to a cross-sectional study from Korea, adults over 70 years of age who are obese and live in urban areas have a significantly lower risk of AMD when they practice intermittent fasting by skipping breakfast(Reference Choi, Kim and Lee255). Interventional prospective studies are needed to confirm the effect of intermittent fasting on AMD.
When assessing the impact of foods and nutrients on health, it is crucial to focus on general nutritional patterns rather than individual foods alone. Among the dietary models under consideration, the Mediterranean diet stands out for its protective effects against AMD. Essentially, the Mediterranean diet encompasses a combination of foods and nutritional elements discussed in preceding sections of the article, believed to be protective against the formation or progression of AMD. Embracing the Mediterranean diet, recognised as one of the healthiest dietary models globally, would be not only logical in terms of overall health effects but also specifically beneficial for AMD. Nevertheless, when delving into dietary patterns and nutritional elements, it is imperative to consider the interplay of genetics with diet and explore potential interactions influencing the development of AMD. In this context, examining nutrition individually and adopting a nutrigenomic approach can prove to be highly valuable.
Conclusions
In this modern world, where we are getting older and exposed to oxidative stress daily, AMD remains an essential eye disease. Some nutrients have long been associated with eye health. Less well-known roles of diet, nutrients, certain bioactive compounds and beneficial biotics appear specific to AMD. At this point, all kinds of nutrients, non-nutrient compounds and nutritional models that can combat oxidative stress and inflammatory situations, which form the basis of the pathophysiology of AMD, come to the fore. On the other hand, when the studies on this subject are examined, it can be easily seen that most of them are not RCTs; instead, they are primarily observation based and have model study results. In addition, studies are focused on supplementation rather than nutrients found in natural foods. This may mislead us about the doses that can be effective, especially for AMD.
In addition to being more cost-effective than clinical or pharmaceutical interventions, diet-based preventive measures are promising; however, due to the lack of clear guidelines in this area, the variability in dietary recommendations provided by physicians, and the need for more consensus between the recommendations and the actual dietary practices of patients with AMD, there is a need for collaboration between physicians and dietitians and for the dietitian support for AMD patients. In light of the information gathered so far, it is advisable to consume high amounts of vegetables, especially incredibly dark-green leafy vegetables and fruits, to prevent the progression of AMD. Besides, choosing low-GI foods instead of high-GI foods and increasing fish and seafood may also be beneficial. Furthermore, regularly consuming nuts (for example, peanuts and walnuts) is recommended as a part of a healthy diet (Fig. 4.). As a dietary model, adherence to the Mediterranean diet, which incorporates all these components, can be recommended, and the Mediterranean-style diet can be considered a reliable diet style for AMD and all kinds of diseases. Moreover, the modulation of the gut microbiota through dietary adjustments with biotics holds potential as a treatment option in conditions with an inflammatory component like AMD. Future studies should be conducted to develop personalised therapeutic and preventive approaches that also consider the interaction between nutrition and genetics.

Fig. 4. Summary of the relationship between food groups/components and AMD.
Acknowledgements
Figures were created with BioRender.com.
Financial support
This research received no specific grant from any funding agency, commercial or not-for-profit sectors.
Competing interests
The authors declare no conflict of interest.
Authorship
Conceptualisation, D.A. and E.K.; writing – original draft preparation, D.A., E.K., N.E.G. and S.A.; writing – review and editing, D.A.; visualisation, N.E.G. and S.A.; supervision, D.A. All authors have read and agreed to the published version of the manuscript.