Book contents
- The Cambridge Handbook of Intelligence
- The Cambridge Handbook of Intelligence
- Copyright page
- Dedication
- Contents
- Figures
- Tables
- Contributors
- Preface
- Part I Intelligence and Its Measurement
- Part II Development of Intelligence
- Part III Intelligence and Group Differences
- Part IV Biology of Intelligence
- Part V Intelligence and Information Processing
- Part VI Kinds of Intelligence
- Part VII Intelligence and Its Role in Society
- Part VIII Intelligence and Allied Constructs
- Part IX Folk Conceptions of Intelligence
- Part X Conclusion
- Author Index
- Subject Index
- References
Part IV - Biology of Intelligence
Published online by Cambridge University Press: 13 December 2019
- The Cambridge Handbook of Intelligence
- The Cambridge Handbook of Intelligence
- Copyright page
- Dedication
- Contents
- Figures
- Tables
- Contributors
- Preface
- Part I Intelligence and Its Measurement
- Part II Development of Intelligence
- Part III Intelligence and Group Differences
- Part IV Biology of Intelligence
- Part V Intelligence and Information Processing
- Part VI Kinds of Intelligence
- Part VII Intelligence and Its Role in Society
- Part VIII Intelligence and Allied Constructs
- Part IX Folk Conceptions of Intelligence
- Part X Conclusion
- Author Index
- Subject Index
- References
Summary
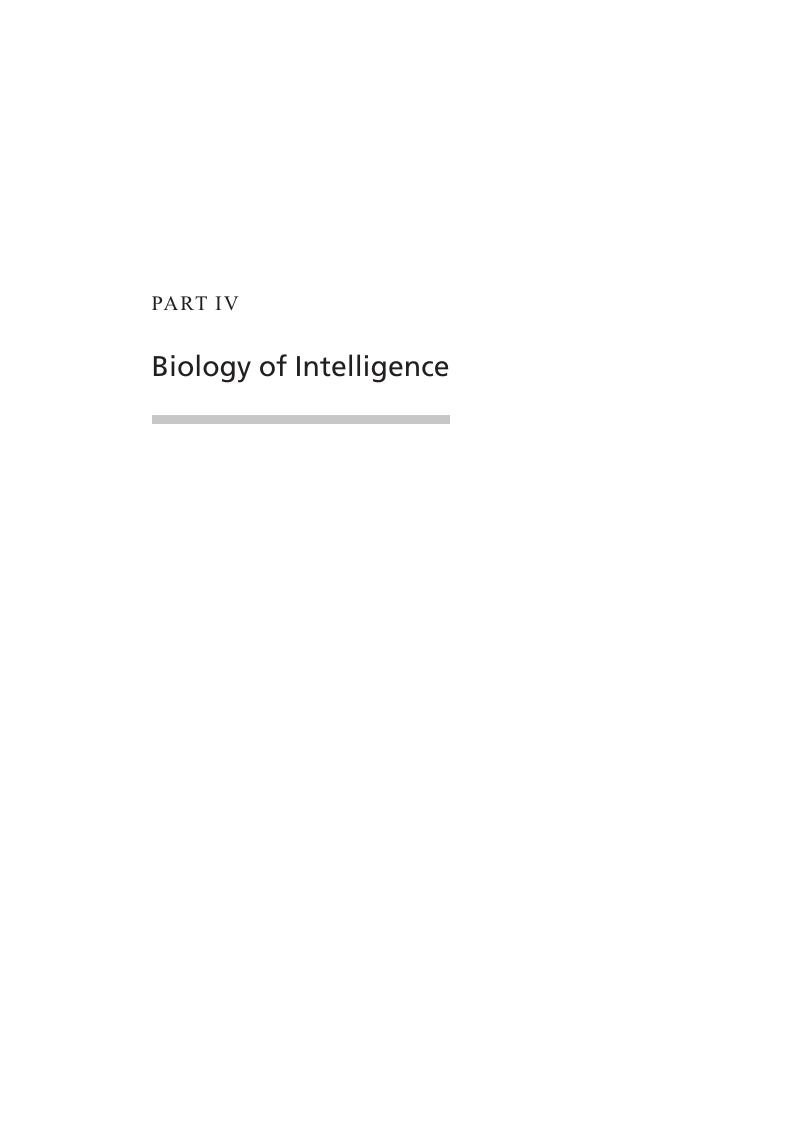
- Type
- Chapter
- Information
- The Cambridge Handbook of Intelligence , pp. 395 - 468Publisher: Cambridge University PressPrint publication year: 2020