Book contents
- Frontmatter
- Contents
- Preface
- Acknowledgments
- PART ONE ANATOMY AND PHYSIOLOGY OF THE CEREBELLAR SYSTEM
- PART TWO CEREBELLAR FUNCTIONS
- PART THREE MODELS AND THEORIES
- PART FOUR SUMMARY AND CONCLUSIONS
- APPENDIX A A Hybrid Analogue/Digital Multiplexer/Multiplier-Based Adaptive Signal Processor
- Author's Note
- Bibliography
- Index
- References
Bibliography
Published online by Cambridge University Press: 28 October 2009
- Frontmatter
- Contents
- Preface
- Acknowledgments
- PART ONE ANATOMY AND PHYSIOLOGY OF THE CEREBELLAR SYSTEM
- PART TWO CEREBELLAR FUNCTIONS
- PART THREE MODELS AND THEORIES
- PART FOUR SUMMARY AND CONCLUSIONS
- APPENDIX A A Hybrid Analogue/Digital Multiplexer/Multiplier-Based Adaptive Signal Processor
- Author's Note
- Bibliography
- Index
- References
Summary
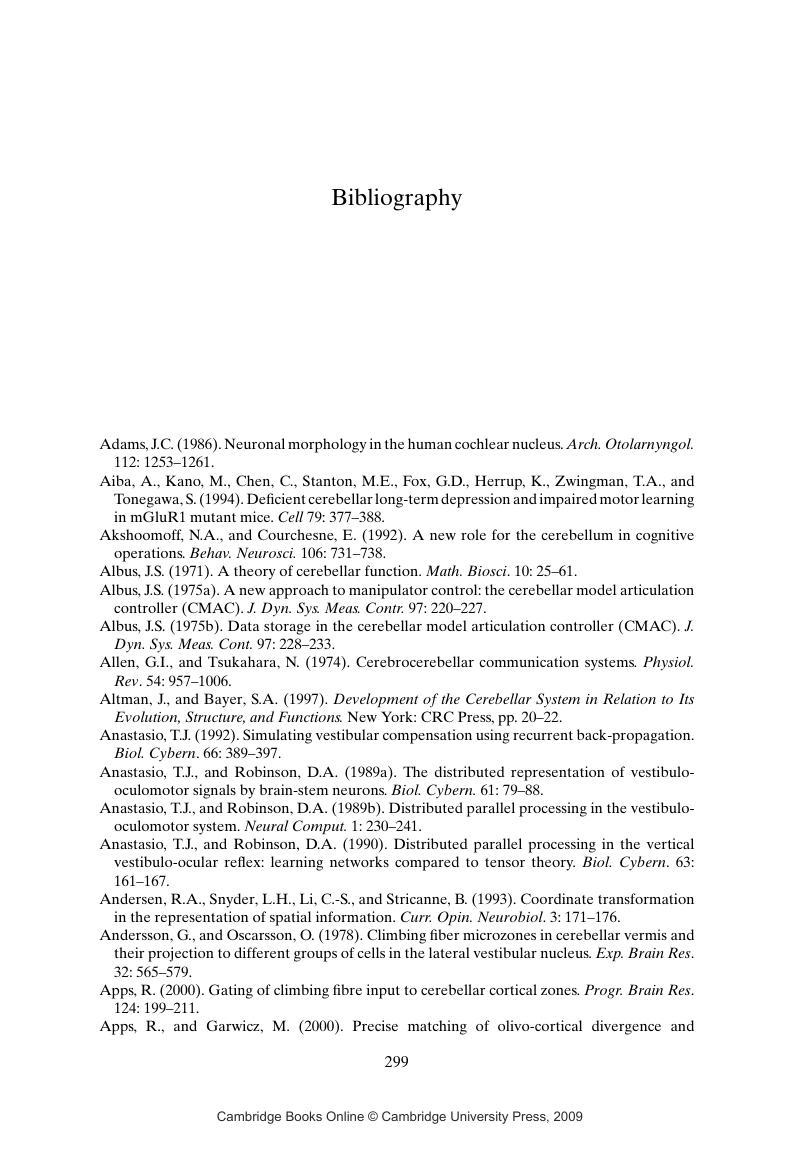
- Type
- Chapter
- Information
- The Cerebellum and Adaptive Control , pp. 299 - 334Publisher: Cambridge University PressPrint publication year: 2002