References
Published online by Cambridge University Press: 04 September 2009
Summary
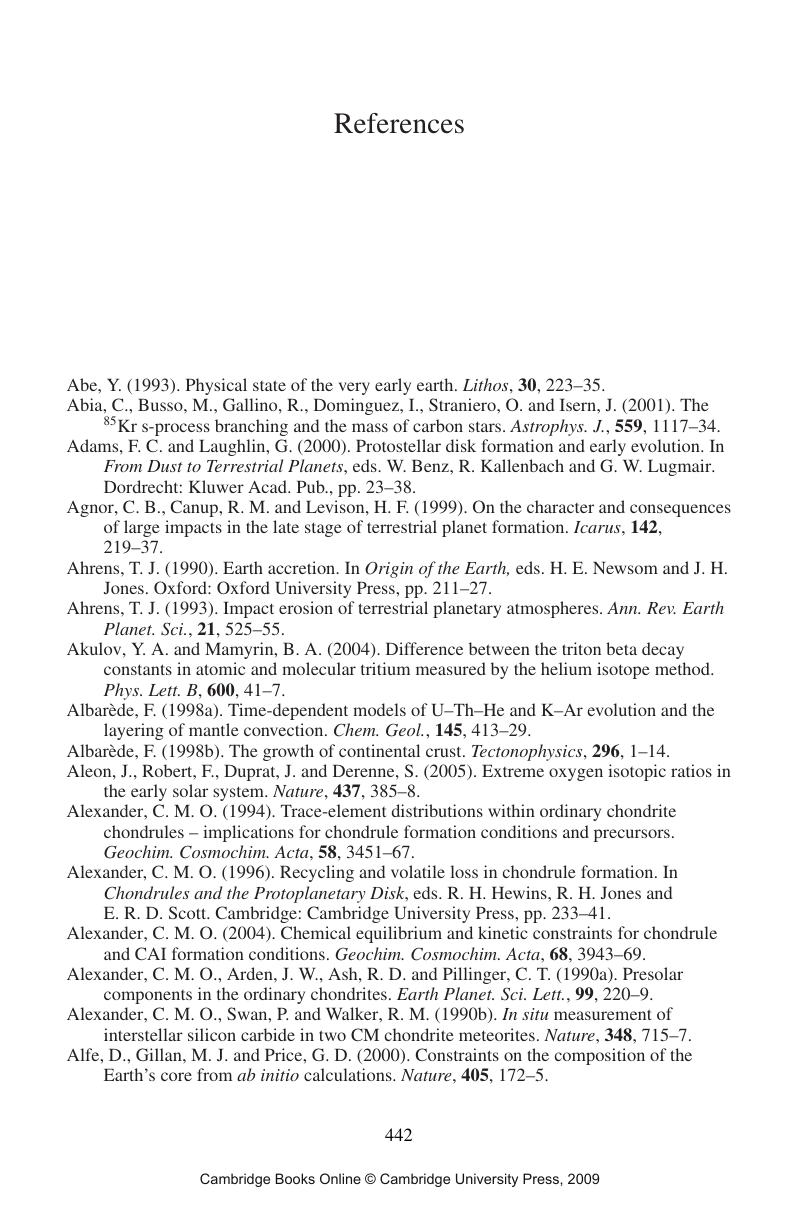
- Type
- Chapter
- Information
- The Evolution of MatterFrom the Big Bang to the Present Day, pp. 442 - 488Publisher: Cambridge University PressPrint publication year: 2008