Book contents
- Frontmatter
- Contents
- Preface
- Acknowledgments
- 1 Introduction to Fed-Batch Cultures
- 2 Idealized Reactors and Fed-Batch Reactors
- 3 Maximization of Reaction Rates and Fed-Batch Operation
- 4 Phenomena That Favor Fed-Batch Operations
- 5 Classification and Characteristics of Fed-Batch Cultures
- 6 Models Based on Mass Balance Equations
- 7 Non–Equation-Based Models
- 8 Specific Rate Determination
- 9 Optimization by Pontryagin's Maximum Principle
- 10 Computational Techniques
- 11 Optimization of Single and Multiple Reactions
- 12 Optimization for Cell Mass Production
- 13 Optimization for Metabolite Production
- 14 Simple Adaptive Optimization
- 15 Measurements, Estimation, and Control
- 16 Feasibility Assessment and Implementable Feed Rates
- Index
- References
13 - Optimization for Metabolite Production
Published online by Cambridge University Press: 05 April 2013
- Frontmatter
- Contents
- Preface
- Acknowledgments
- 1 Introduction to Fed-Batch Cultures
- 2 Idealized Reactors and Fed-Batch Reactors
- 3 Maximization of Reaction Rates and Fed-Batch Operation
- 4 Phenomena That Favor Fed-Batch Operations
- 5 Classification and Characteristics of Fed-Batch Cultures
- 6 Models Based on Mass Balance Equations
- 7 Non–Equation-Based Models
- 8 Specific Rate Determination
- 9 Optimization by Pontryagin's Maximum Principle
- 10 Computational Techniques
- 11 Optimization of Single and Multiple Reactions
- 12 Optimization for Cell Mass Production
- 13 Optimization for Metabolite Production
- 14 Simple Adaptive Optimization
- 15 Measurements, Estimation, and Control
- 16 Feasibility Assessment and Implementable Feed Rates
- Index
- References
Summary
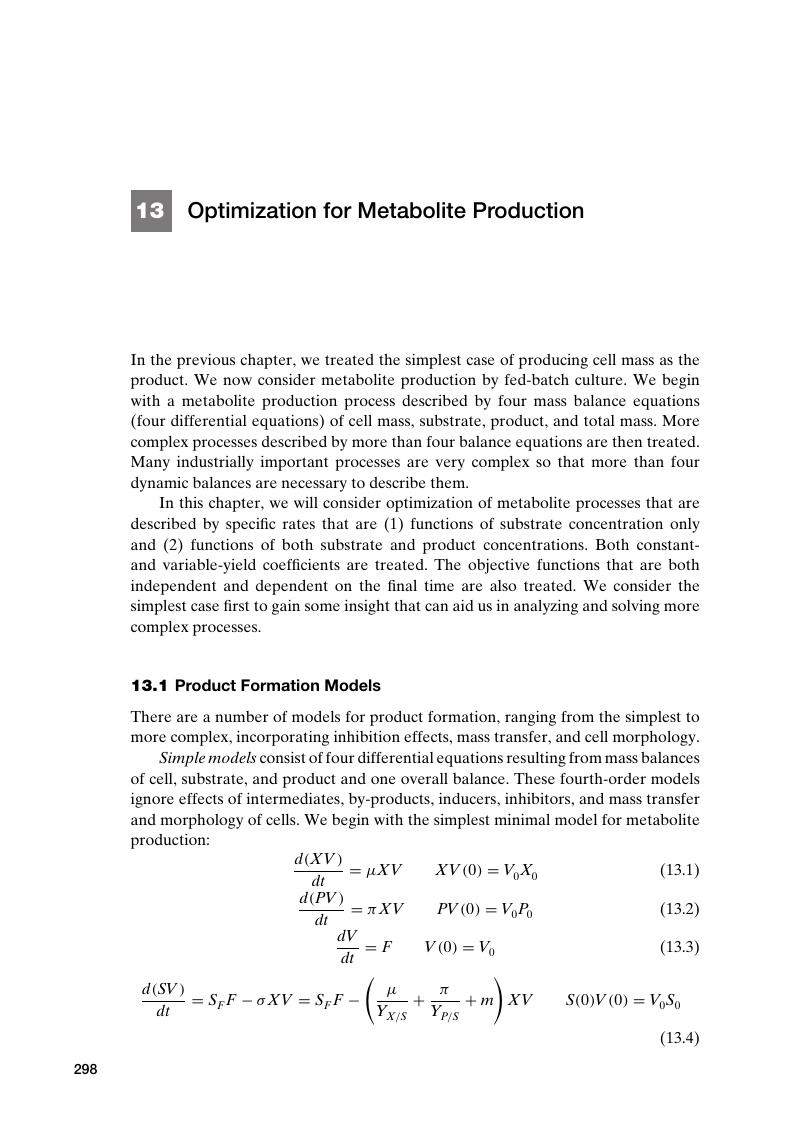
- Type
- Chapter
- Information
- Fed-Batch CulturesPrinciples and Applications of Semi-Batch Bioreactors, pp. 298 - 392Publisher: Cambridge University PressPrint publication year: 2013