Book contents
- Genome Editing and Engineering
- Genome Editing and Engineering
- Copyright page
- Dedication
- Contents
- Contributors
- Foreword
- Preface
- Part I Biology of Endonucleases (Zinc-Finger Nuclease, TALENs and CRISPRs) and Regulatory Networks
- Part II Genome Editing in Model Organisms
- Part III Technology Development and Screening
- Part IV Genome Editing in Stem Cells and Regenerative Biology
- Part V Genome Editing in Disease Biology
- 21 CRISPR/Cas9-based In Vivo Models of Cancer
- 22 Inducible CRISPR-based Genome Editing for the Characterization of Cancer Genes
- 23 Genome Editing for Retinal Diseases
- 24 Manipulation of Long Non-coding RNAs in Cardiovascular Disease Using Genome Editing Technology
- 25 Gene Silencing, Disruption and Latency Reactivation with RNA-based and Gene Editing CRISPR/Cas, ZFN and TALEN Technologies for HIV-1/AIDS Therapies
- 26 Use of the CRISPR/Cas9 System for Genome Editing of Immune System Cells, Defense Against HIV-1 and Cancer Therapies
- 27 Harnessing the Therapeutic Potential of Long Non-coding RNAs in Immunity
- Part VI Legal (Intellectual Property) and Bioethical Issues of Genome Editing
- Index
- Plate Section (PDF Only)
- References
21 - CRISPR/Cas9-based In Vivo Models of Cancer
from Part V - Genome Editing in Disease Biology
Published online by Cambridge University Press: 30 July 2018
- Genome Editing and Engineering
- Genome Editing and Engineering
- Copyright page
- Dedication
- Contents
- Contributors
- Foreword
- Preface
- Part I Biology of Endonucleases (Zinc-Finger Nuclease, TALENs and CRISPRs) and Regulatory Networks
- Part II Genome Editing in Model Organisms
- Part III Technology Development and Screening
- Part IV Genome Editing in Stem Cells and Regenerative Biology
- Part V Genome Editing in Disease Biology
- 21 CRISPR/Cas9-based In Vivo Models of Cancer
- 22 Inducible CRISPR-based Genome Editing for the Characterization of Cancer Genes
- 23 Genome Editing for Retinal Diseases
- 24 Manipulation of Long Non-coding RNAs in Cardiovascular Disease Using Genome Editing Technology
- 25 Gene Silencing, Disruption and Latency Reactivation with RNA-based and Gene Editing CRISPR/Cas, ZFN and TALEN Technologies for HIV-1/AIDS Therapies
- 26 Use of the CRISPR/Cas9 System for Genome Editing of Immune System Cells, Defense Against HIV-1 and Cancer Therapies
- 27 Harnessing the Therapeutic Potential of Long Non-coding RNAs in Immunity
- Part VI Legal (Intellectual Property) and Bioethical Issues of Genome Editing
- Index
- Plate Section (PDF Only)
- References
Summary
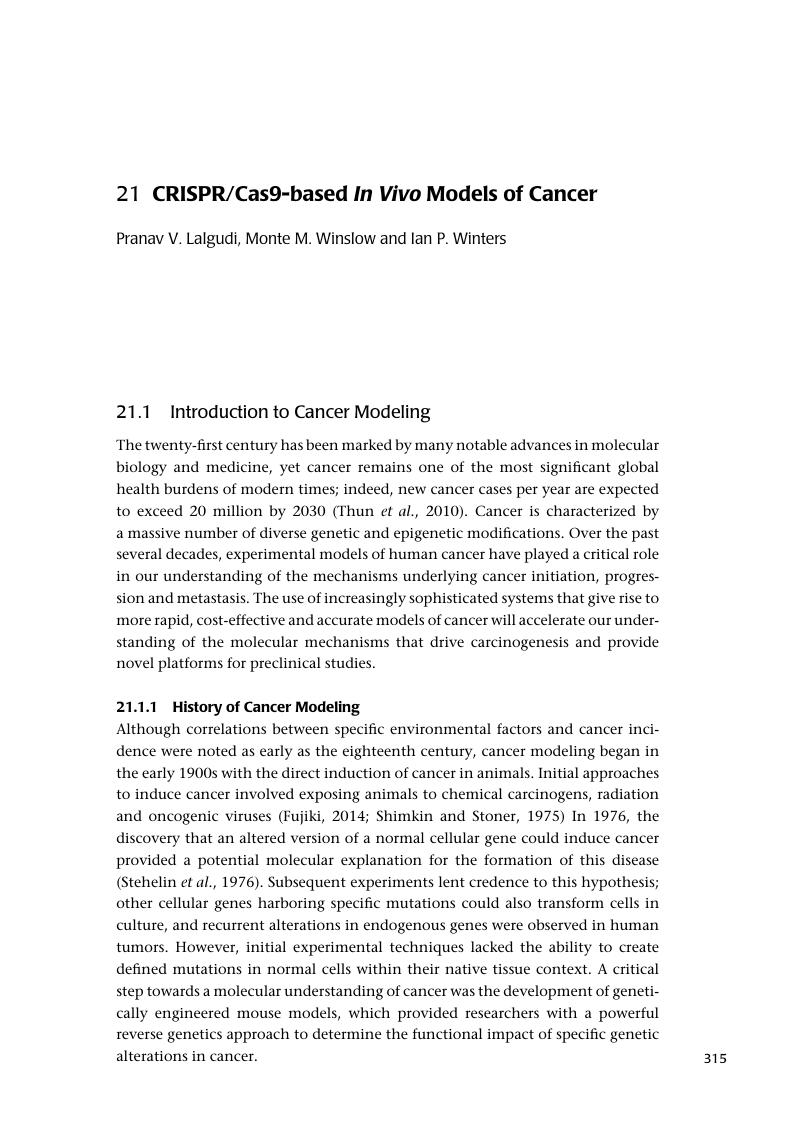
- Type
- Chapter
- Information
- Genome Editing and EngineeringFrom TALENs, ZFNs and CRISPRs to Molecular Surgery, pp. 315 - 336Publisher: Cambridge University PressPrint publication year: 2018
References
- 1
- Cited by