Book contents
- Frontmatter
- Dedication
- Contents
- Preface
- Acknowledgements
- 1 A Trinity of Duplexities
- 2 A Trinity of Duplexities
- 3 From Elements of Lie Symmetries to Lorentz Algebra
- 4 Representations of Lorentz
- 5 Discrete Symmetries: Part 1 (Parity)
- 6 Discrete Symmetries: Part 2 (Charge Conjugation)
- 7 Eigenspinors of Charge Conjugation Operator, Elko
- 8 Construction of Elko
- 9 A Hint for Mass Dimension One Fermions
- 10 CPT for Elko
- 11 Elko in Shirokov-Trautman, Wigner and Lounesto Classifications
- 12 Rotation-Induced Effects on Elko
- 13 Elko-Dirac Interplay: A Temptation and a Departure
- 14 An Ab Initio Journey into Duals
- 15 Mass Dimension One Fermions
- 16 Mass Dimension One Fermions as a First Principle Dark Matter
- 17 Continuing the Story
- Appendix Further Reading
- References
- Index
- References
- Frontmatter
- Dedication
- Contents
- Preface
- Acknowledgements
- 1 A Trinity of Duplexities
- 2 A Trinity of Duplexities
- 3 From Elements of Lie Symmetries to Lorentz Algebra
- 4 Representations of Lorentz
- 5 Discrete Symmetries: Part 1 (Parity)
- 6 Discrete Symmetries: Part 2 (Charge Conjugation)
- 7 Eigenspinors of Charge Conjugation Operator, Elko
- 8 Construction of Elko
- 9 A Hint for Mass Dimension One Fermions
- 10 CPT for Elko
- 11 Elko in Shirokov-Trautman, Wigner and Lounesto Classifications
- 12 Rotation-Induced Effects on Elko
- 13 Elko-Dirac Interplay: A Temptation and a Departure
- 14 An Ab Initio Journey into Duals
- 15 Mass Dimension One Fermions
- 16 Mass Dimension One Fermions as a First Principle Dark Matter
- 17 Continuing the Story
- Appendix Further Reading
- References
- Index
- References
Summary
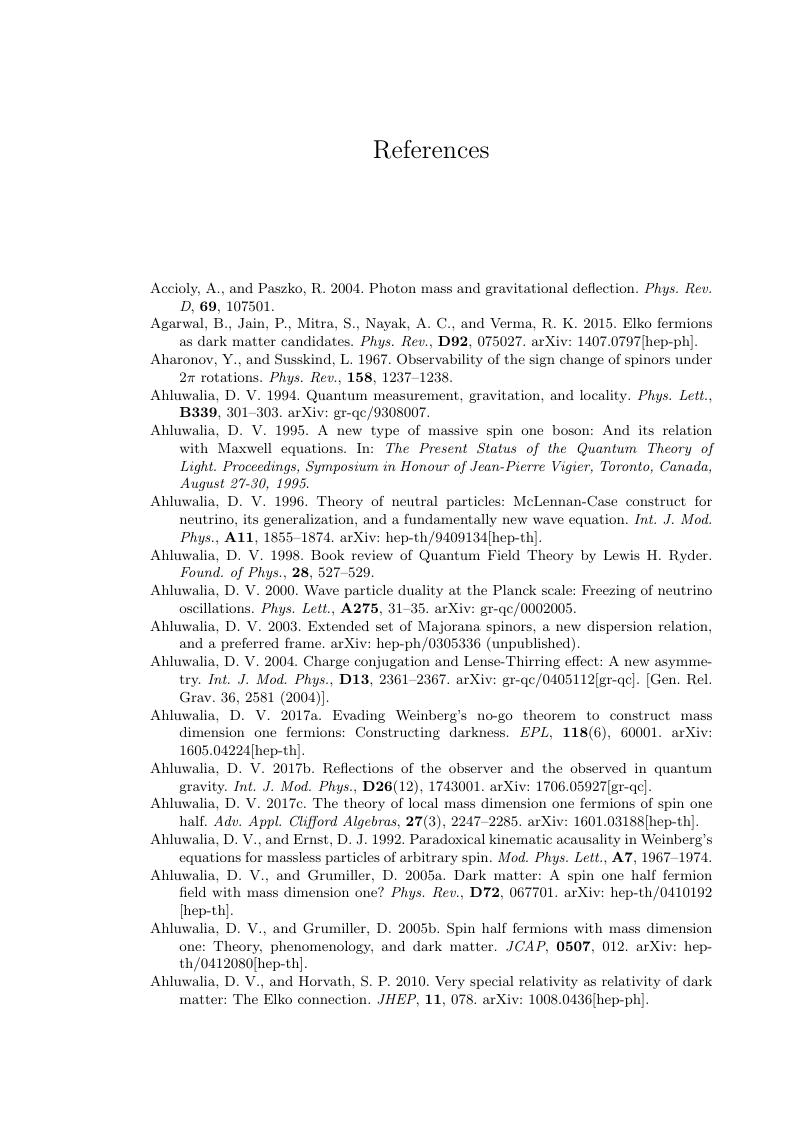
- Type
- Chapter
- Information
- Mass Dimension One Fermions , pp. 104 - 112Publisher: Cambridge University PressPrint publication year: 2019