Book contents
- Frontmatter
- Contents
- Acknowledgments
- 1 Introduction
- 2 The intact peripheral nerve tree
- 3 Injuries to peripheral nerves
- 4 Addressing nerve regeneration
- 5 Early regenerative events
- 6 Consolidation and maturation of regeneration
- 7 Regeneration and the vasa nervorum
- 8 Delayed reinnervation
- 9 Trophic factors and peripheral nerves
- 10 The nerve microenvironment
- References
- Index
- Plate section
- References
References
- Frontmatter
- Contents
- Acknowledgments
- 1 Introduction
- 2 The intact peripheral nerve tree
- 3 Injuries to peripheral nerves
- 4 Addressing nerve regeneration
- 5 Early regenerative events
- 6 Consolidation and maturation of regeneration
- 7 Regeneration and the vasa nervorum
- 8 Delayed reinnervation
- 9 Trophic factors and peripheral nerves
- 10 The nerve microenvironment
- References
- Index
- Plate section
- References
Summary
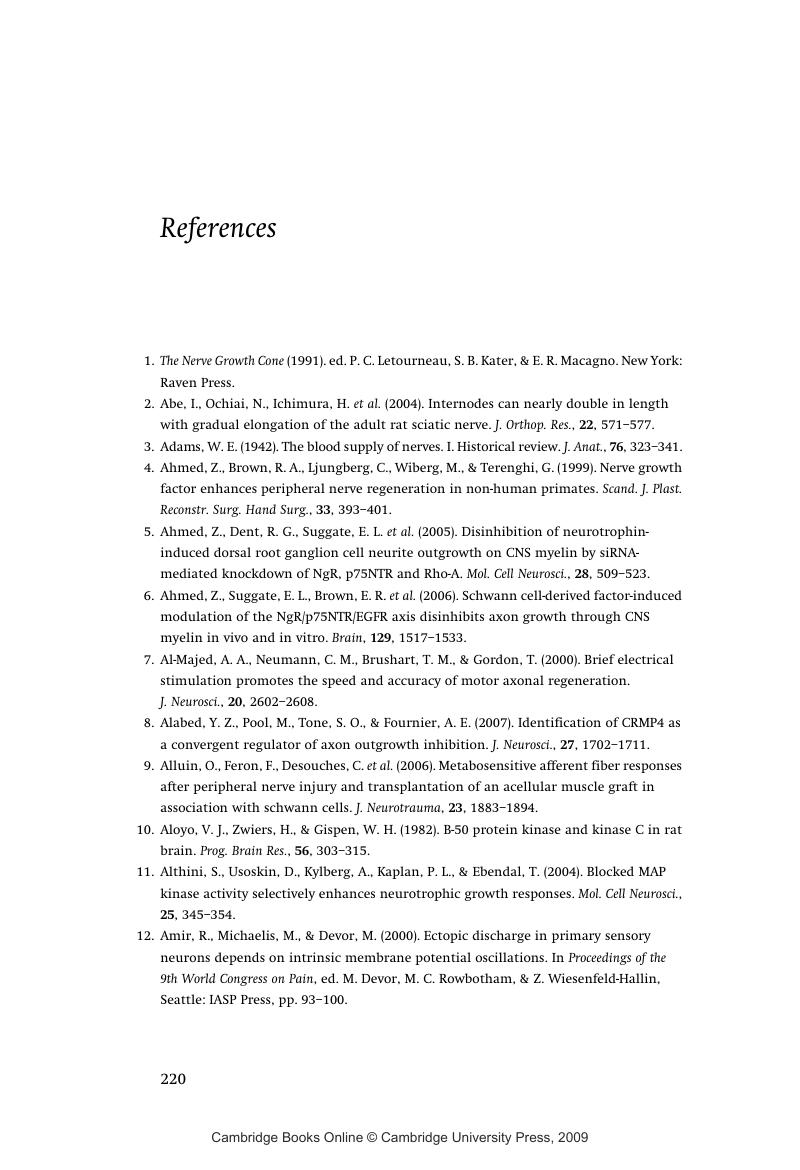
- Type
- Chapter
- Information
- Neurobiology of Peripheral Nerve Regeneration , pp. 220 - 266Publisher: Cambridge University PressPrint publication year: 2008