The monoamines serotonin (5-hydroxytryptamine; 5-HT) and dopamine (DA) are important neurotransmitters/modulators involved in integration of the behavioural and neuroendocrine responses to stress(Reference Chaouloff1–Reference Höglund, Kolm and Winberg4). Comparative models suggest common mechanisms for dietary modulation of release and transmission by these signal substances in vertebrates. For instance, it has been demonstrated that dietary supplementation with the essential amino acid l-tryptophan (Trp), the immediate precursor of 5-HT, enhances brain serotonergic activity in teleosts(Reference Höglund, Bakke and Øverli5–Reference Winberg, Overli and Lepage8) and mammals, including man(Reference Markus, Verschoor and Firk9). In general, the synthesis of central 5-HT depends largely on the plasma concentration ratio between Trp and other large neutral amino acids (LNAA), since they all enter the brain through the same carrier transporter located on the blood–brain barrier(Reference Curzon, Sandler and Silverstone10, Reference Fernstrom11). Once inside the neuron, Trp is transformed by tryptophan-5-hydroxylase (EC 1.14.16.4) into 5-hydroxytryptophan, which subsequently is decarboxylated to 5-HT by aromatic l-amino acid decarboxylase (EC 4.1.1·28)(Reference Leathwood12, Reference Fernstrom and Wurtman13). Furthermore, among the LNAA competing with Trp for access into the brain is tyrosine. This amino acid is the precursor of the catecholamine DA, and it has been suggested that Trp may influence central DA activity through this mechanism(Reference Lepage, Vilchez and Pottinger7).
Dietary manipulations of the ratio between Trp and other LNAA have been used to investigate the involvement of central 5-HT in the regulation of appetite, aggressive behaviour, and the neuroendocrine stress response in mammals, including human subjects as well as in comparative vertebrate models, such as fish(Reference Höglund, Bakke and Øverli5, Reference Markus, Olivier and Panhuysen14, Reference Höglund, Sørensen and Bakke15). Studies in rainbow trout (Oncorhynchus mykiss) have shown that dietary Trp enrichment suppresses aggressive behaviour as well as the neuroendocrine stress response(Reference Lepage, Tottmar and Winberg6, Reference Winberg, Overli and Lepage8). Furthermore, Lepage et al. (Reference Lepage, Vilchez and Pottinger7) observed that the attenuation in stress response appeared to be time-specific; rainbow trout displayed lower plasma cortisol concentrations after 7 d of treatment with Trp-enriched feed, effects which were not evident when the experimental diet regimen lasted for 3 or 28 d(Reference Lepage, Tottmar and Winberg6, Reference Lepage, Vilchez and Pottinger7). Compensatory mechanisms, such as up-regulation of Trp-catabolising enzymes, have been suggested to prevent a long-term effect, when dietary treatment with Trp is extended beyond 7 d(Reference Lepage, Vilchez and Pottinger7). For instance, tryptophan 2,3 dioxygenase (EC 1.13.11.11; TDO) plays an important role as a house-keeping enzyme in the regulation of circulating levels of Trp(Reference Smith and Pogson16). Still, it is currently unknown for how long the stress-reducing effect of a 7 d regimen with Trp supplementation lasts if the fish are reintroduced to a diet with standard amino acid composition.
Generally, during stressful situations, there is a positive correlation between brain 5-HT activity and circulating glucocorticoids, the endproduct in the hypothalamic–pituitary–adrenocortical (HPA; mammals) or hypothalamic–pituitary–interrenal (HPI; teleosts) axis(Reference Chaouloff1, Reference Winberg and Lepage17). Thus, the observation that Trp supplementation stimulates 5-HT production and simultaneously suppresses HPA or HPI axis responsiveness may seem contradictory in this regard. However, as mentioned above, the suppression in the stress-induced release of cortisol became apparent only after 7 d of dietary Trp pre-treatment, suggesting an indirect effect of increased 5-HT signalling(Reference Lepage, Vilchez and Pottinger7). Interestingly, repeated daily administration with selective 5-HT reuptake inhibitors have been reported to exert similar effects as Trp supplementation on HPA or HPI axis reactivity among rodents and fish, implying common mechanisms such as changes in 5-HTergic receptor expression and/or sensitivity for both treatments(Reference Lepage, Larson and Mayer18, Reference Jensen, Jessop and Harbuz19). Studies in rainbow trout have also shown that Trp supplementation attenuates the stress-induced elevation of plasma adrenocorticotropic hormone levels, indicating that the stress-reducing effects of Trp may be mediated by mechanisms upstream in the HPI axis(Reference Lepage, Vilchez and Pottinger7). In teleosts, the nucleus lateralis tuberis (NLT) in the hypothalamus is innervated by DA and 5-HT fibres, containing corticotrophin-releasing factor-, DA- and 5-HT-producing cells that project down to the pituitary(Reference Corio, Peute and Steinbusch20–Reference Rao, Job and Schreibman22). However, the extent to which the stress-reducing effects of Trp act on this level of the HPI axis has still not been thoroughly described. Moreover, in mammals the limbic system in the forebrain is involved in the control of HPA axis activity (for more information, see reviews by Feldman et al. (Reference Feldman, Conforti and Weidenfeld23) and Jacobson & Sapolsky(Reference Jacobson and Sapolsky24)). Although different developmental patterns in the forebrain early in ontogeny restrict comparative studies between teleosts and mammals, recent findings indicate that the lateral pallial regions (Dl) of the telencephalon may correspond to the mammalian hippocampus(Reference Portavella and Vargas25). Furthermore, studies in rainbow trout suggest that these telencephalic regions elicit an inhibitory action on the neuroendocrine stress response(Reference Øverli, Korzan and Larson26). However, it remains to be determined whether the stress-reducing effects of Trp are reflected in changes in DA and 5-HT neurochemistry in these brain areas.
In view of the above, the aims of the present study were to: (1) investigate if the stress-reducing effect, as measured by plasma cortisol levels, of dietary Trp supplementation persists after switching back to standard feed with normal amino acid composition; and (2) evaluate whether DA and 5-HT activity in the NLT and Dl are involved in mediating these endocrine effects in juvenile Atlantic cod (Gadus morhua). A putative dampening effect of dietary Trp on cortisol responses to stress may potentially be put to practical use in fish-farming conditions (for example, during transport, vaccination and sorting).
Materials and methods
Experimental animals
The experimental animals used in the present study were 1-year-old juvenile farmed Atlantic cod. During spring 2007, approximately 1200 cod were purchased from the commercial aquaculture company Marine Harvest AS, and transported to the aquarium facility at Solbergstrand Marine Research Station. At the beginning of the experiment the fish weight varied between 51 and 149 g (mean 91·8 (sem 0·9) g; n 182). Before the experiment, the fish were kept in two 1 m3 indoor holding tanks at a density of approximately 45 kg/m3 for approximately 1 month. The tanks were continuously supplied (30 litres/min) with ambient seawater obtained from a depth of 60 m (Oslo fjord). The temperature varied between 7 and 12 °C (mean 8·6 (sem 0·1) °C), while the salinity varied between 33·6 and 35·3 ‰ (mean 34·3 (sem 0·03) ‰) during the experimental period, and the pH remained stable at about 8·0. The light–dark (L:D) regimen was automatically controlled and adjusted to conditions at latitude 59°N (Oslo, Norway). While the fish were in the holding tanks, they were manually fed with commercial cod pellets (BioMar, 4 mm) at approximately 1 % of their body mass per d. The experiment was conducted in accordance with the Norwegian animal welfare act, and approved by the Norwegian experimental animals committee (FDU).
Experimental conditions
The experimental environment used in the present study consisted of thirty-six identical glass aquaria (40 litres), each divided into two compartments, continuously supplied (0·5 litres/min) with ambient sea water and equipped with tightly fitted transparent lids. The lids were equipped with small holes (0·5 cm in diameter) through which food could be added to the aquaria. The water outlet was connected to pipes running outside the aquaria, which allowed for regulation of the water level (Fig. 1). A reduction in water level to 7 cm served as an acute confinement stressor for the individual fish. The L:D regimen was adjusted to the same conditions as for the period preceding the experiments.
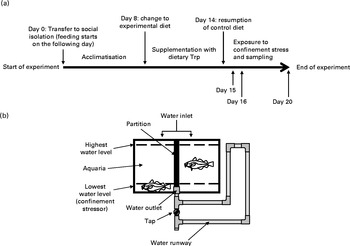
Fig. 1 (a) Overview of experiment design. Trp, l-tryptophan. (b) Illustration of the housing conditions; the water level indicates whether or not the fish is subjected to confinement.
A total of four different experimental feeds were prepared by the aquafeed company BioMar AS in Brande, Denmark, which had different levels of Trp in relation to the total amount of other LNAA. The four different feeds, specifically developed for cod, were identical in energetic value and differed only in Trp content: the control feed contained 0·47 % Trp (1xTrp) and the experimental feeds contained 0·82 % Trp (2xTrp), 1·14 % Trp (3xTrp) and 1·65 % Trp (4xTrp).
Experimental protocol
During July 2007, at the start of the experiment, fish (of approximate equal size) were randomly selected, weighed and quickly transferred to each of the seventy-two compartments within the thirty-six aquaria to obtain social isolation. In order to facilitate acclimatisation to their new environment, all fish were fed control feed for a period of 7 d. Subsequently fish were either fed 1xTrp, 2xTrp, 3xTrp or 4xTrp (n 4–19, see Figs. 4–6 for specific details regarding sample size) feed for 7 d, after which the fish were then again fed control feed (1xTrp) for 0, 1 or 5 d (see Fig. 1 for an overview of the set-up). Individual food intake was quantified once per d by counting the number of pellets consumed. For quantification of food intake, individual fish were fed with one pellet at a time until the fish rejected three pellets in a row. To avoid possible bias induced by a insufficient treatment time with Trp(Reference Lepage, Vilchez and Pottinger7), fish that ate for less than 4 d during the 7 d period with experimental diet were omitted from the dataset. The fish were starved for 24 h during the phase comprising the reintroduction of control feed before sampling was initiated. Subsequently within this timeframe the fish were then sampled either directly (undisturbed groups) or after 30 min of confinement stress (lowering of water level; see Fig. 1) on the morning following the last day of feeding (1, 2 or 6 d post-experimental diet).
Sampling procedure
At sampling, individual fish were quickly anaesthetised (ethyl-m-aminobenzoate methanesulfonate (Benzocain); 500 mg/l) and a blood sample (approximately 0·6 ml) was collected from the caudal vessel using a syringe pre-treated with EDTA. Blood samples collected were immediately centrifuged (13 000 rpm; 4 °C; 2 min) and the plasma fractions were transferred to 1·5 ml Eppendorf tubes, and subsequently snap-frozen on dry ice. In the sampling sessions conducted at 1 d following the Trp treatment brains were also collected from the fish sculls, and embedded in Tissue-Tec (O.C.T. Compound, Sakura Finetek AS). Plasma samples and whole brains were stored at − 70°C for later analyses of cortisol levels and monoaminergic activity.
Assays
The concentration of plasma cortisol in the samples was measured using a commercial ELISA kit (product no. 402710; Neogen Corp.). Plasma samples were diluted 1:100 in assay buffer and the samples processed according to the kit protocol. The enzyme reaction was terminated by adding 1 m-HCl, and subsequently the plates were read in a conventional plate reader at 450 nm (Vmax Microplate Reader; Molecular Devices). The percentage bound for each standard solution was used to generate a logarithmic curve. This curve was used to calculate the concentrations of plasma cortisol.
Before brain monoamine analysis, the brains were sectioned into 300 μm thick slices using a cryostat (PMV 450 MP; Palmstierna Mekaniska Verkstad) at − 21°C. The slices were mounted on glass plates, and sections containing the lateral pallial parts of the telencephalon (Dl) as well as the NLT were micro-dissected using the sharpened end of a level-cut syringe (0·5 mm in inner diameter) (Fig. 2). These two tissues were then each added to a 4 % perchloric acid solution (200 μl) containing 0·26 g/l EDTA and 19·4 ng/ml epinin (deoxyepinin) as internal standard and mechanically homogenised using an Eppendorf pestle. Subsequently, the samples were centrifuged for 2 min at 30 000 g and 4°C. The supernatant fraction (100 μl) was used immediately to quantify concentrations of 5-HT, DA, 5-hydroxyindoleacetic acid (5-HIAA) and 3,4-dihydroxyphenylacetic acid (DOPAC), a major DA metabolite, by HPLC with electrochemical detection as described by Øverli et al. (Reference Øverli, Harris and Winberg27). Samples were quantified by comparison with standard solutions of known concentrations and corrected for recovery of the internal standard using HPLC software (CSW; DataApex Ltd). Analysis of actual levels, expressed as catabolite or monoamine content/total protein, was not possible due to the samples containing remaining proteins after supernatant fraction were lost. Instead, we used the ratio of the catabolite to its parent monoamine as an estimation of central monoaminergic activity. This is commonly used as an index of monoaminergic activity especially when studying behaviour and stress(Reference Summers28, Reference Shannon, Gunnet and Moore29). Neurotransmitter ratios (5-HIAA:5-HT or DOPAC:DA) were calculated from the concentrations in each sample and presented as either serotonergic or dopaminergic activity.
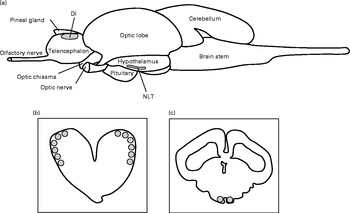
Fig. 2 Lateral view of the teleost brain (a) and cross-sections of regions analysed for monoaminergic activity: lateral pallial parts of telencephalon (Dl) (b) and nucleus lateralis tuberis (NLT) (c), highlighted in grey.
Data treatment and statistics
All data were subjected to tabular and graphical examination and analysis in Microsoft Excel®, before being transferred to Stata SE/11 for Windows (StataCorp LP) for further statistical evaluation. The large variation in the results and variances between treatments made it difficult to find a suitable statistical approach to evaluate all observations in one statistical analysis. To overcome these problems, the analyses were therefore performed on data within each sampling time (i.e. days after Trp treatment), and for cortisol within unstressed and stressed fish. Due to lack of normality, the tests were run on log-transformed data for the cortisol results. For the monoamine data, log-transformation was not sufficient to achieve normal distribution, thus non-parametric methods were used to analyse these results. Effects on plasma cortisol were investigated using one-way ANOVA, whereas brain monoaminergic ratios were evaluated employing Kruskal–Wallis tests and quantile regression. A Spearman rank correlation test was used to assess correlations between the ratios of 5-HIAA:5-HT and DOPAC:DA, for each brain region. Results regarding plasma cortisol levels and monoamine ratios are presented as mean values with their standard errors and medians, respectively.
Results
Appetite
Feed intake increased steadily in all groups during the 7 d acclimatisation period preceding treatment with elevated dietary Trp. On average the feed intake increased from 0·07 % to 0·37 % of their body weight during this period. After the acclimatisation period, feed intake continued to increase in all groups during both the experimental and post-experimental diet periods. Fish receiving the Trp-supplemented diets did not appear to differ in feed intake during the treatment period or after the reintroduction of commercial feed when compared with the control group (Fig. 3).
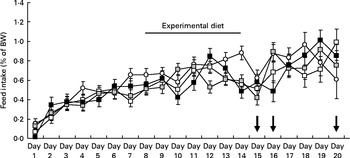
Fig. 3 Daily feed intake in juvenile cod (Gadus morhua) over the course of the experiment: control feed containing 0·47 % l-tryptophan (Trp) (1xTrp; ); experimental feed containing 0·82 % Trp (2xTrp;
); experimental feed containing 1·14 % Trp (3xTrp;
); experimental feed containing 1·65 % Trp (4xTrp;
). Data are means, with standard errors represented by vertical bars. The arrows on days 15, 16 and 20 highlight when the confinement stressor was introduced. BW, body weight.
Stress response
As shown in Fig. 4, a dose-dependent decline in plasma cortisol levels was observed among stressed fish on day 1 after dietary Trp treatment was terminated (ANOVA; P< 0·056). The effect was no longer evident on day 2 (P< 0·84) and day 6 (P< 0·11). Unstressed fish showed low cortisol levels at all three sampling times and did not seem to be affected by Trp supplementation on day 1 (P< 0·80) or day 2 (P< 0·19), but some changes were observed on day 6 (P< 0·007) during which fish fed 4xTrp displayed a somewhat higher cortisol response than the group on the control diet.
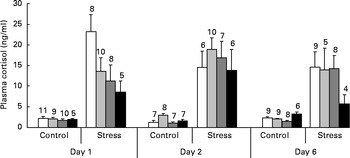
Fig. 4 Plasma cortisol levels in juvenile cod (Gadus morhua) following the reintroduction of control diet after 7 d on l-tryptophan (Trp) supplementation: control feed containing 0·47 % Trp (1xTrp; □); experimental feed containing 0·82 % Trp (2xTrp; ); experimental feed containing 1·14 % Trp (3xTrp;
); experimental feed containing 1·65 % Trp (4xTrp; ■). Data are means, with standard errors represented by vertical bars. The values above the bars indicate the number of individual fish. ANOVA on stressed fish: day 1, P< 0·056; day 2, P< 0·84; day 6, P< 0·11. ANOVA on undisturbed fish: day 1, P< 0·80; day 2, P< 0·19; day 6, P< 0·007.
Brain monoamine activity in nucleus lateralis tuberis and lateral pallial region of telencephalon
Initial graphical examination of regional brain monoamine activity, as indicated by the metabolite:neurotransmitter ratio, showed no visible effect of stress in either the NLT or Dl on day 1 after termination of Trp treatment. Thus, stressed and undisturbed fish were pooled before subsequent statistical analysis was performed. The results are shown in Figs. 5 and 6 and reveal a curvilinear relationship on both monoamine ratios. Supplementation with Trp revealed an effect on the 5-HIAA:5-HT ratio in the Dl (Kruskal–Wallis test: P< 0·015) and further analysis showed that this monoamine ratio was higher at moderate doses of dietary Trp supplementation (quantile regression: 2xTrp: P< 0·032; 3xTrp: P< 0·047; 4xTrp: P< 0·132) when compared against control feed. A similar pattern for the 5-HIAA:5-HT ratio was also observed in the NLT (Kruskal–Wallis test: P< 0·01) in which the effects seemed most pronounced at 3xTrp (quantile regression: 2xTrp: P< 0·08; 3xTrp: P< 0·05; 4xTrp: P< 0·31). While no clear effects of Trp supplementation were found on the DOPAC:DA ratio in the Dl (Kruskal–Wallis test: P< 0·21; quantile regression: 2xTrp: P< 0·08; 3xTrp: P< 0·48; 4xTrp: P< 0·28), there was a significant elevation of this monoamine ratio in the NLT (Kruskal–Wallis test: P< 0·04). Further analysis revealed that supplementation with 2xTrp resulted in an increased DOPAC:DA ratio, an effect which was no longer apparent at higher doses of the amino acid (quantile regression: 2xTrp: P< 0·02; 3xTrp: P< 0·17; 4xTrp: P< 0·27).
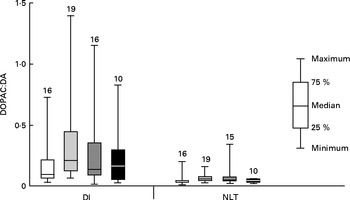
Fig. 5 Dopaminergic activity assessed by 3,4-dihydroxyphenylacetic acid:dopamine (DOPAC:DA) ratio in the lateral pallial telencephalon (Dl) and nucleus lateralis tuberis (NLT) in juvenile cod (Gadus morhua) on day 1 after dietary l-tryptophan (Trp) supplementation was terminated: control feed containing 0·47 % Trp (1xTrp; □); experimental feed containing 0·82 % Trp (2xTrp; ); experimental feed containing 1·14 % Trp (3xTrp;
); experimental feed containing 1·65 % Trp (4xTrp; ■). Results are presented as boxplots by pooling stressed and undisturbed fish. The values above the bars indicate the number of individual fish. Kruskal–Wallis test for Dl: P< 0·21, quantile regression with comparison against 1xTrp; 2xTrp, P< 0·08; 3xTrp, P< 0·48; 4xTrp, P< 0·28. Kruskal–Wallis test for NLT: P< 0·04, quantile regression with comparison against 1xTrp; 2xTrp, P< 0·02; 3xTrp, P< 0·17; 4xTrp, P< 0·27.
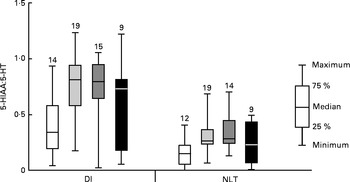
Fig. 6 Serotonergic activity assessed by 5-hydroxyindoleacetic acid:5-hydroxytryptamine (5-HIAA:5-HT) ratio in the lateral pallial telencephalon (Dl) and nucleus lateralis tuberis (NLT) in juvenile cod (Gadus morhua) on day 1 after dietary l-tryptophan (Trp) supplementation was terminated: control feed containing 0·47 % Trp (1xTrp; □); experimental feed containing 0·82 % Trp (2xTrp; ); experimental feed containing 1·14 % Trp (3xTrp;
); experimental feed containing 1·65 % Trp (4xTrp; ■). Results are presented as boxplots by pooling stressed and undisturbed fish. The values above the bars indicate the number of individual fish. Kruskal–Wallis test for Dl: P< 0·015, quantile regression with comparison against 1xTrp; 2xTrp, P< 0·032; 3xTrp, P< 0·047; 4xTrp, P< 0·13. Kruskal–Wallis test for NLT: P< 0·01, quantile regression with comparison against 1xTrp; 2xTrp, P< 0·08; 3xTrp, P< 0·05; 4xTrp, P< 0·31.
A significant moderate correlation (R 2s 0·33; P< 0·015) was found between the 5-HIAA:5-HT and DOPAC:DA ratios in the NLT, while there was only a trend (R 2s 0·22; P< 0·099) in the Dl.
Discussion
The present study showed a clear dose-dependent relationship between plasma cortisol and dietary levels of Trp at 1 d after termination of the experimental feed regimen, amongst fish subjected to confinement stress. However, the suppression in stress-induced cortisol response was no longer apparent on days 2 and 6 after the dietary treatment period, indicating that the effects of Trp supplementation are rather temporary. The observation that Trp treatment for 7 d resulted in decreased plasma cortisol levels suggests that this amino acid acts on the HPI axis stress reactivity in a similar way in Atlantic cod as previously reported in rainbow trout(Reference Lepage, Tottmar and Winberg6). In that study, undisturbed trout also responded to Trp-enriched feed by a slight increase in basal plasma cortisol levels. In the present study, a similar effect was only seen at 6 d following termination of Trp supplementation, although the significance of this finding is difficult to explain and further studies are needed to verify whether it is an artifact or not.
The absence of changes in monoamine activity, estimated by the 5-HIAA:5-HT and DOPAC:DA ratios, stemming from confinement stress alone in the present results seems somewhat contradictory to the general consensus that an increase in central 5-HT activity is related to an activation of the neuroendocrine stress response(Reference Lepage, Tottmar and Winberg6, Reference Winberg and Lepage17, Reference Øverli, Harris and Winberg27, Reference Höglund, Balm and Winberg30, Reference Winberg and Nilsson31). However, the 5-HTergic response to stress has been shown to differ both temporally and regionally in a comparative animal model, the lizard Anolis carolinensis (Reference Summers, Larson and Summers32). This has also been demonstrated in rainbow trout where social stress induced different patterns in 5-HTergic activity between the Dl and dorsomedial parts of the telencephalon(Reference Øverli, Korzan and Larson26). In the latter study, the authors suggested that the Dl, which is functionally similar to the mammalian hippocampus, exerts an inhibitory effect on the cortisol stress response. However, the role of 5-HT in this brain region is relatively unknown in teleosts, and it is possible that the findings by Øverli et al. (Reference Øverli, Korzan and Larson26) specifically reflect social interaction (with regards to 5-HT activity in the Dl) rather than just a general stress response.
In teleosts it has been shown that brain monoamines innervate the NLT(Reference Corio, Peute and Steinbusch20–Reference Rao, Job and Schreibman22) and that there are corticotrophin-releasing factor-producing neurons within this nucleus that project down into the pituitary. Still, the actual function of these serotonergic fibres within this hypothalamic region has not been well described(Reference Lillesaar33). Both the NLT and the pre-optic area have been suggested to be involved in the regulation of adrenocorticotropic hormone(Reference Fryer and Peter34). Furthermore, exposure to chronic stress has been shown to affect the corticotrophin-releasing factor cells in the NLT(Reference Norris, Felt and Woodling21), but it is possible that the endocrine response to acute stressors, such as confinement, is controlled by alternate pathways in other brain regions.
Independent of confinement stress, dietary supplementation of Trp affected 5-HT activity in both the Dl and NLT in the present study. This seems to be in agreement with the findings of Höglund et al. (Reference Höglund, Bakke and Øverli5), who reported a general increase in brain 5-HT activity after 7 d of Trp treatment in cod. According to the present data, the largest changes in 5-HT activity were attributed to moderate doses of Trp, in which 5-HIAA:5-HT ratios were elevated compared with fish fed standard feed continuously. However, 5-HT activity appeared to regress towards control levels in both brain regions in those fish receiving the highest dose of dietary Trp (corresponding to four times the Trp content of the standard feed). Interestingly, the present results showed that DA activity in the NLT also appeared to be stimulated by Trp administration, albeit to a lesser extent than 5-HT activity, as judged by the trend in increased DOPAC:DA ratio. Although the general consensus is that Trp increases 5-HT activity, supplementation with this LNAA has previously been found to have no clear effects on either the 5-HIAA:5-HT or DOPAC:DA ratios, and only elevated 5-HIAA levels in the hypothalamus and optic tectum after 7 d on experimental diet in rainbow trout(Reference Lepage, Tottmar and Winberg6, Reference Lepage, Vilchez and Pottinger7). In contrast, Winberg et al. (Reference Winberg, Overli and Lepage8) reported increased levels of 5-HIAA as well as 5-HIAA:5-HT ratios in both the hypothalamus and brainstem, but not in the telencephalon in an experiment examining the effects on aggressive behaviour in rainbow trout receiving the same dietary Trp treatment. A possible explanation for the conflicting data between these two studies could be that the observed effects of Trp enrichment on brain 5-HT signalling are more pronounced when combined with other treatments, such as exposure to an acute stressor(Reference Lepage, Tottmar and Winberg6, Reference Lepage, Vilchez and Pottinger7) or social interactions(Reference Winberg, Overli and Lepage8). Such differences in context may activate alternate neural pathways contributing to the inconsistencies in 5-HT signalling between the studies mentioned above. Nonetheless, our data indicate that 5-HTergic activity regresses towards control levels in fish receiving the highest dose of dietary Trp supplementation. Similar dietary levels of this amino acid have previously been shown to induce an inhibition of behavioural responses to social challenges, such as aggression towards an intruder(Reference Winberg, Overli and Lepage8) and attenuation of the anorectic response to novelty(Reference Höglund, Sørensen and Bakke15) in salmonid fish. The observation that 5-HT activity approached control levels in both the Dl and NLT in fish receiving the highest Trp dose makes it tempting to interpret that this reflects such suppressive effects on responses to acute challenges. However, the functional roles of these brain regions, with regards to monoaminergic activity, in regulating behavioural and endocrine responses to acute challenges still remain largely unknown in teleosts. It should also be noted that in the present study we were only able to quantify monoaminergic activity as the catabolite:parent monoamine ratio, and it is possible that actual levels of monoamines and their catabolites could have revealed other effects on monoaminergic signalling within the same context.
In humans, reducing the synthesis of central 5-HT (by depriving the brain of Trp) can induce depression and anxiety-related disorders(Reference Delgado, Charney and Price35, Reference Neumeister, Turner and Matthews36). Despite this, the potency of Trp supplementation as an antidepressant has a rather narrow therapeutic window (for a review, see Shaw et al. (Reference Shaw, Turner and Del Mar37)). It is well known that the endogenous amount of Trp, available for 5-HT synthesis, is regulated by Trp-catabolising enzymes such as TDO. This enzyme is activated not only by its substrate Trp, but can also be triggered by glucocorticoids(Reference Gál and Sherman38, Reference Rubin39). Providing excess exogenous Trp stimulates the activity of TDO, enhancing conversion of this amino acid into kynurenin; thus leaving less Trp available for 5-HT synthesis and subsequent neurotransmission. While extensively studied in mammals, there is less information in the literature when it comes to TDO regulation of Trp in teleost fish. It is possible that the lack of effects on the endocrine stress response in the present study (i.e. at days 2 and 6 after dietary Trp supplementation) may somehow be reflected by changes in the catabolic activity of TDO. However, Walton et al. (Reference Walton, Coloso and Cowey40) observed no alterations in the activity of this enzyme in rainbow trout when given different levels of dietary Trp. A similar lack in effect has also been reported in channel catfish (Ictalurus punctatus), in which repeated doses of either dietary Trp or adrenocorticotropic hormone or three doses of glucocorticoids failed to induce hepatic TDO(Reference Brown and Dodgen41). Clearly, this topic needs to be addressed in future studies, taking into account other Trp-catabolising enzymes involved in the kynurenin pathway as well.
In conclusion, pretreatment with Trp-enriched feed for 7 d resulted in a dose-dependent reduction on post-stress plasma cortisol levels, confirming the observation that dietary Trp supplementation can suppress the endocrine response in Atlantic cod, as previously documented in rainbow trout(Reference Lepage, Tottmar and Winberg6, Reference Lepage, Vilchez and Pottinger7). The attenuation in stress response was no longer evident at 2 or 6 d following the termination of Trp administration, indicating a rather short time window for the stress-reducing effect of this amino acid. Concomitant with the temporary decrease in endocrine response, moderate dietary doses of Trp, containing two to three times the levels of commercial feed, acted stimulatory in general on both brain serotonergic and dopaminergic (to lesser extent) activity. However, this effect appeared to return towards control levels, as determined from monoamine ratios, at a dietary Trp dose corresponding to four times the content in commercial feed. In light of a previous study which reported a suppression in aggressive behaviour in rainbow trout at similar Trp doses(Reference Winberg, Overli and Lepage8), the present data may indicate that the brain regions NLT and Dl potentially elicit an inhibitory effect on the behavioural responses to social challenges. However, the finding that monoaminergic activity was unaffected by acute stress might also reflect alternative roles for these two brain regions, such as partial participation in suppression on the endocrine stress response. On an applied level, further studies are required to assess whether dietary Trp can be used as a potential nutritional strategy in aquaculture conditions.
Acknowledgements
The present study was supported and funded by BioMar AS and the Norwegian Research Council. We would like to thank the staff at Solbergstrand Station for providing us with the facilities for this experiment. D. B. conducted the study, carried out the cortisol analysis, was involved in analysis/interpretation of the data and wrote substantial parts of the manuscript. J. S. was involved in planning/designing of the study, interpretation of data and in writing the manuscript, built the experimental set-up and conducted the study. S. W. was involved in planning/designing of the study, interpretation of data and in writing the manuscript.Å. K. was involved in analysis/interpretation of data and the manuscript review. E. S. evaluated the statistical methods and performed the data analysis, was involved in interpretation of data and manuscript review. K. v. K. carried out the monoamine analysis and was involved in the manuscript review. M. H. was involved in planning/designing of the study, interpretation of data and the manuscript review. I. M. was involved in planning/designing of the study and the manuscript review. E. H. was involved in planning/designing of the study, analysis/interpretation of data and in writing the manuscript. The authors declare no conflict of interest.