The majority of apple dietary fibre and polyphenols (flavonoids and phenolic acids) accumulate in the fruit peel, which after the manufacture of fruit juices and beverages remains as pomace(Reference Kosmala, Kolodziejczyk and Markowski1). The technology used to obtain apple concentrate is highly efficient; however, from 12 to 20 % of processed raw material becomes a by-product and, after production, is treated as waste mass. The most frequently applied ways of recycling apple wastes are for animal feed and composting or by disposal in dumps, the latter being a great waste of beneficial compounds present in the pomace (i.e. fibres and polyphenols). The need to exploit fibre and fibre–phenolic by-products from apple processing for the manufacture of functional additives to food is therefore substantiated(Reference Sudha, Baskaran and Leelavathi2).
It has been speculated that the beneficial activity of polyphenolic compounds present in unrefined diets is linked, to a great extent, to the physiological effects of dietary fibre constituting the fibre–polyphenol complexes, also referred to as ‘antioxidant dietary fibre’(Reference Juśkiewicz, Żary-Sikorska and Zduńczyk3, Reference Lopez-Oliva, Agis-Torres and Goni4). Esposito et al. (Reference Esposito, Arlotti and Bonifati5) conclude results of their study with a statement that the fibre–polyphenol complex seems to be more beneficial to the consumer's health than the highly-purified medicinal preparation of dietary fibre or antioxidants. The fibre–antioxidant complex seems to be a natural route of delivering components with antioxidative properties to large gut microbiota, thus protecting antioxidants against degradation in the stomach(Reference Lopez-Oliva, Agis-Torres and Goni4). This points to potential advantages of diet supplementation with natural preparations containing both functional polysaccharides and polyphenolic compounds, which enables utilising the physiological properties of both groups of these compounds locally in the gut as well as in the internal tissues. Our recent study showed that the applied addition of the polyphenolic fraction from chicory root to diets containing prebiotic fructans did not diminish the positive effect of the prebiotic on the gut ecosystem and triggered positive changes in the lipid profile of blood serum as well as deceleration of pro-oxidative processes in selected tissues(Reference Juśkiewicz, Zduńczyk and Żary-Sikorska6). Polyphenols are also potential modulators of digestive fermentation processes because, in part, they escape absorption in the small intestine and reach the large intestine, where they themselves or their metabolites affect colonic/caecal bacteria(Reference Juśkiewicz, Milala and Jurgoński7). Some reports have addressed the negative effect of relatively high amounts of dietary polyphenols on the direction and rate of large gut fermentation processes(Reference Frejnagel and Juśkiewicz8); with reference to that, our previous study demonstrated that the presence of grapefruit polyphenols in diets for rats did not restrict the positive effects of dietary fibre in the caecum, whereas the presence of fibre was proved to be successful in eliminating the undesirable effects of the former on metabolism parameters of the caecum, including, e.g. increases in pH value and excess accumulation of digesta, inhibition of the glycolytic activity of microbiota and diminished production of SCFA(Reference Zduńczyk, Juśkiewicz and Estrella9).
The objective of the present study on Wistar rats was to identify the physiological effects of the coupled action of metabolites of endogenous digestion of a dietary fibre fraction and polyphenols from apple pomace. It was hypothesised that removing, through ethanolic extraction, a large part of the polyphenolic fraction of dietary apple pomace would further affect the physiology of the gastrointestinal tract (mainly caecal fermentation) and the systemic response of the body (antioxidative status and blood lipoprotein). To assess whether the polyphenolic component of pomace is largely responsible for the assumed beneficial effect in rats, experimental diets were supplemented with two different apple preparations: native, unprocessed apple pomace abundant in polyphenols and a processed product deprived of most of these compounds via ethanol extraction.
Material and methods
Apple pomaces
Commercial apple pomace from the manufacture of clarified juices (dessert apple cultivar, with enzyme-assisted pressing) was obtained from the ALPEX Company (Łęczeszyce, Poland). Fresh pomace from the Bücher-type press was dried in a convection oven at a temperature ≤ 70°C until the moisture content was lower than 5 %, and then the dried material was passed through sieves with 5- and 3-mm mesh. Fractions with particle sizes lower than 3 mm and higher than 5 mm and devoid of seed were ground in a disc mill to sizes of < 0·6 mm. The preparation thus obtained constituted the natural, unprocessed apple pomace. The processed apple pomace was subsequently prepared when phenolic compounds were removed from pomace using ethanol extraction. The residue obtained after drying in the air oven constituted the processed apple pomace.
Chemical analyses of plant material
The chemical compositions of both apple pomaces are detailed in Table 1. Each pomace was analysed in duplicate for DM, crude protein, fat, ash, total dietary fibre and insoluble dietary fibre using Association of Official Analytic Chemists(10) 934.01, 920.152, 930.09, 940.26, 985.29 and 993.19 methods, respectively. HPLC analysis of phenolic compounds in unprocessed and processed apple pomaces was also performed(Reference Juśkiewicz, Milala and Jurgoński7).
Table 1 Composition of apple preparations used as dietary components
(Mean values and standard deviations, n 3)
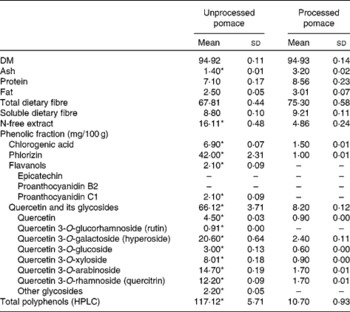
* Mean values within a row were significantly different (P ≤ 0·05).
Extraction of polyphenolic compounds from apple pomaces was performed as follows: 0·5 g of each freeze-dried sample was mixed with 4 ml of solvent (70 % ethanol, v/v) and sonicated for 15 min. After centrifugation at 4800 g for 5 min, the supernatant was collected and the residue was re-extracted twice with 3 ml of the same solvent. The pooled extracts were made up to 10 ml. The ethanolic extracts, containing most of the soluble polyphenols, were analysed by HPLC (a Dionex system with diode array detection (Germering, Germany) coupled with a Gemini 5-μm C18 110A column (250 × 4·6 mm; Phenomenex Synergi, Torrance, CA, USA)). The elution solvents were A (0·05 % phosphoric acid in water) and B (0·05 % phosphoric acid in acetonitrile). A flow rate of 1·25 ml/min and a temperature of 25°C were set as the operating conditions. For the linear gradient method, the program started with 4 % B from 0 to 5 min as gradient stabilisation, 4–15 % B from 5 to 12·5 min, 15–40 % B from 12·5 to 42·4 min, 40–50 % B from 42·4 to 51·8 min, 50 % B from 51·8 to 53·4 min, 4 % B from 53·4 to 55 min and a post-run with 4 % B for 10 min to equilibrate the column for the next injection. The standards of chlorogenic acid, phlorizin, epicatechin and rutin (quercetin 3-O-glucorhamnoside) were acquired from Sigma-Aldrich (Poznań, Poland), and procyanidin B2, quercetin, hyperoside (quercetin 3-O-galactoside), quercetin 3-O-glucoside and quercitrin (quercetin 3-O-rhamnoside) were supplied from Extrasynthese Company (Genay, France). The identification of procyanidin C1 was based on literature data of the UV spectrum, and the identification of quercetin 3-O-xyloside and quercetin 3-O-arabinoside was based partly on the literature and our data that had been acquired previously with HPLC/MS(Reference Juśkiewicz, Milala and Jurgoński7). The results were quoted as the sum of polyphenols, and detailed as chlorogenic acid, phlorizin, flavanols and the sum of quercetin and its glycosides, which are considered to be particularly biologically active groups among all apple polyphenols(Reference Lu and Foo11).
Animal study
The animal protocol used in the present study was approved by the local Institutional Animal Care and Use Committee. The assessment was conducted on twenty-four male Wistar rats aged 35 d and weighing 120·1 (sem 1·72) g, and randomly divided into three groups of eight animals each. All animals were housed individually over 4 weeks in metabolic cages with free access to water and semi-purified casein diets (Table 2). The selection of the animals and their maintenance over the 28 d experiment followed common regulations. The environment was controlled with a 12 h light–12 h dark cycle, a temperature of 21 ± 1°C, relative humidity of 50 (sem 5) % and twenty air changes/h. All experimental diets were similar in terms of dietary ingredients except for the phenolic fraction. The control diet, containing α-cellulose as the fibre source, contained no phenolic substances (experimental group C). The diet supplemented with 14 % of processed apple pomace contained 0·002 % of dietary polyphenols and the diet supplemented with 15 % of unprocessed apple pomace contained 0·018 % of apple phenolics (groups A and AP, respectively). In both experimental diets, the apple preparations were added at the expense of cellulose and a part of maize starch.
Table 2 Composition of the diets

C, control; A, diet supplemented with 14 % of processed apple pomace; AP, diet supplemented with 15 % unprocessed apple pomace.
* Casein preparation containing 89·7 % protein, 0·3 % fat, 2·0 % ash and 8·0 % water (Lacpol Company, Murowana Goslina, Poland).
† SPI Procon 2100 IP (Solae Company, St Louis, MO, USA).
‡ Kruszwica SA Company, Kruszwica, Poland.
§ Sigma-Aldrich (Poznań, Poland).
∥ AIN-93G-MX (per kg mix): 357 g calcium carbonate anhydrous (40·04 % Ca), 196 g potassium phosphate monobasic (22·76 % P and 28·73 % K), 70·78 g potassium citrate, tripotassium monohydrate (36·16 % K), 74 g NaCl (39·34 % Na and 60·66 % Cl), 46·6 g potassium sulphate (44·87 % K and 18·39 % S), 24 g magnesium oxide (60·32 % Mg), 6·06 g ferric citrate (16·5 % Fe), 1·65 g zinc carbonate (52·14 % Zn), 1·45 g sodium meta-silicate 9H2O (9·88 % Si), 0·63 g manganous carbonate (47·79 % Mn), 0·3 g cupric carbonate (57·47 % Cu), 0·275 g chromium potassium sulphate 12H2O (10·42 % Cr), 81·5 mg boric acid (17·5 % B), 63·5 mg sodium fluoride (45·24 % F), 31·8 mg nickel carbonate (45 % Ni), 17·4 mg lithium chloride (16·38 % Li), 10·25 mg sodium selenate anhydrous (41·79 % Se), 10 mg potassium iodate (59·3 % I), 7·95 mg ammonium paramolybdate 4H2O (54·34 % Mo), 6·6 mg ammonium vanadate (43·55 % V) and 221·026 g powdered sucrose.
¶ AIN-93G-VM (g/kg mix): 3·0 nicotinic acid, 1·6 calcium pantothenate, 0·7 pyridoxine-HCl, 0·6 thiamin-HCl, 0·6 riboflavin, 0·2 folic acid, 0·02 biotin, 2·5 vitamin B12 (cyanocobalamin, 0·1 % in mannitol), 15·0 vitamin E (all-rac-α-tocopheryl acetate, 500 mg/g), 0·8 vitamin A (all-trans-retinyl palmitate, 275 mg/g), 0·25 vitamin D3 (cholecalciferol, 10 mg/g), 0·075 vitamin K1 (phylloquinone), 974 655 powdered sucrose.
** AVEBE, Veendam, Holland.
During the study, the rats were subjected to N balance that was preceded by a 10 d preliminary period. In the 5 d period of the proper experiment, faeces and urine were thoroughly collected from all rats that were kept in balance cages (Tecniplast Spa, Buguggiate, Italy). The content of total N in diets, faeces and urine collected in the balance period was assayed using the Association of Official Analytic Chemists(10) 920.152 method.
Experimental groups were additionally monitored for body weight gains (body weight was recorded at the beginning and on termination of the study) and diet intake (daily monitoring), which enabled the calculation of the feed conversion ratio. Faecal pH was measured using a microelectrode and a pH/ion meter (model 301; Hanna Instruments, Vila do Conde, Portugal) after the 1st, 2nd, 3rd and 4th week of experimental feeding. At the termination of the experiment, the rats were anaesthetised with sodium pentobarbital according to the recommendations for euthanasia of experimental animals. After laparotomy, blood samples were taken from the caudal vena cava, and then small intestine, caecum, colon, liver, heart, kidneys and lungs were removed and weighed. The small intestine was divided into four equal parts, and the second part (jejunum) from the stomach side was rinsed with ice-cold physiological saline and cut open. The mucosal samples were collected by scraping with glass slides on an iced glass plate, weighed and subsequently stored at − 40°C. Disaccharidase activities (sucrase, maltase and lactase) were assayed by the method of Messer & Dahlqvist(Reference Messer and Dahlqvist12) with modifications. The amount of liberated glucose was measured spectrophotometrically and the enzyme activity was expressed as micromoles of disaccharide hydrolysed/min per g of protein. Samples of caecal contents were used for the immediate analysis of ammonia, DM and SCFA, whereas the rest of the caecal digesta was transferred to tubes and stored at − 70°C. From the fresh caecal digesta, ammonia was extracted, trapped in a solution of boric acid in Conway's dishes and determined by direct titration with sulphuric acid. The DM of the digesta was determined at 105°C.
Caecal digesta samples were subjected to SCFA analysis using GC (Shimadzu GC-2010, Kyoto, Japan). The samples (0·2 g) were mixed with 0·2 ml formic acid, diluted with deionised water and centrifuged at 7211 g for 10 min. The supernatant was loaded onto a capillary column (SGE BP21, 30 m × 0·53 mm; SGE Europe Ltd, Milton Keynes, UK) using an on-column injector. The initial oven temperature was 85°C and was raised to 180°C by 8°C/min increase and held there for 3 min. The temperatures of the flame ionisation detector and the injection port were 180 and 85°C, respectively. The sample volume for GC analysis was 1 μl. The caecal SCFA pool size was calculated as the sum of SCFA concentration in the digesta and caecal digesta mass.
The activity of bacterial enzymes (β-glucosidase and β-glucuronidase) released into the environment was measured by the rate of p- or o-nitrophenol release from their nitrophenylglucosides, according to the method described elsewhere(Reference Juśkiewicz and Zduńczyk13), in fresh faeces, which were taken after each week of experiment, and in the caecal digesta on termination of the experiment (α- and β-glucosidase, α- and β-galactosidase and β-glucuronidase). The following substrates were used: ρ-nitrophenyl-α-d-glucopyranoside (for α-glucosidase); ρ-nitrophenyl-β-d-glucopyranoside (for β-glucosidase); ρ-nitrophenyl-α-d-galactopyranoside (α-galactosidase); ο-nitrophenyl-β-d-galactopyranoside (β-galactosidase); ρ-nitrophenyl-β-d-glucuronide (for β-glucuronidase). The reaction mixture contained 0·3 ml of substrate solution (5 mm) and 0·2 ml of 1:10 (v/v) dilution of the caecal:faecal sample in 100 mm phosphate buffer (pH 7·0) after centrifugation at 7211 g for 15 min. Incubation was carried out at 37°C and ρ-nitrophenol was quantified at 400 and 420 nm (ο-nitrophenol concentration) after addition of 2·5 ml of 0·25 m cold sodium carbonate. The enzymatic activity (α- and β-glucosidase, α- and β-galactosidase and β-glucuronidase) was expressed as micromol quantity of the product formed/h per g of digesta.
The extent of lipid peroxidation in selected tissues (kidneys, lungs, liver and heart) and in the serum was measured by quantifying malondialdehyde formed in terms of thiobarbituric acid-reactive substances (TBARS)(Reference Uchiyama and Mihara14). Serum concentrations of TAG, total cholesterol and its HDL fraction were estimated with reagents from Alpha Diagnostics Limited (Warsaw, Poland). The activity of glutathione peroxidase in the heparinised blood and superoxide dismutase in erythrocyte lysate were determined using reagents from Randox Laboratories Limited (Crumlin, Antrim, UK). The serum antioxidant capacity of water-soluble and the antioxidant capacity of lipid-soluble substances (Analytik Jena AG, Jena, Germany) were determined by a photochemiluminescence detection method, using a Photochem (Analytik Jena AG). In the photochemiluminescence assay, the generation of free radicals was partially eliminated by the reaction with antioxidants present in the serum samples, and the remaining radicals were quantified by luminescence generation. Ascorbate and Trolox calibration curves were used in order to evaluate antioxidant capacity of water-soluble and lipid-soluble substances, respectively, and the results were expressed as micromole concentration of ascorbate or Trolox equivalents per ml of serum.
Statistical analyses
Results were analysed statistically using one-way ANOVA and the significance of differences between groups was determined using Duncan's multiple-range test at a significance level of P ≤ 0·05. Data are expressed as mean values and standard errors of the mean. Calculations were done using STATISTICA 8.0 (StatSoft Corporation, Kraków, Poland).
Results
After the 1st and 3rd week of the experiment, the faecal pH in group C was significantly (P ≤ 0·05) higher than in group AP as well as in group A on days 21 and 28 of the study (Table 3). The activity of faecal β-glucosidase released into the environment did not differ among the groups. Diet supplementation with both apple pomaces examined resulted in significant decreases in faecal β-glucuronidase activity on days 21 and 28 of experimental feeding (P ≤ 0·05 v. C group).
Table 3 Faecal pH value and bacterial β-glucosidase and β-glucuronidase activity in rats fed with diets supplemented with apple pomaces*
(Mean values with their standard errors)
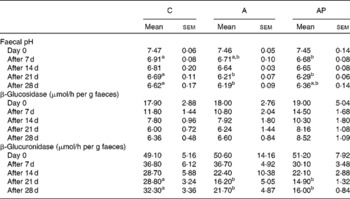
C, control; A, diet supplemented with 14 % of processed apple pomace; AP, diet supplemented with 15 % unprocessed apple pomace.
a,b Mean values within a row with unlike superscript letters were significantly different (P ≤ 0·05).
* The dietary content of dietary polyphenols in the experimental groups was as follows: C, 0 %; A, 0·002 %; AP, 0·018 %.
The apple products applied in the study had no significant effect on either the extent of body weight gains or on diet intake (Table 4). The addition of both test apple pomaces increased faecal N excretion significantly, thereby the highest value of the apparent digestibility index of N was noted in the C group (P ≤ 0·05 v. the other groups). The quantity of N excreted with urine was significantly lower in the AP group than in the C group. The retention index, considering loss of N both with faeces and urine, did not differ statistically between the groups. The dietary supplementation with the analysed apple pomaces had no significant effect on either the pH value of ileal digesta or on the mass of the small intestine along with digesta. In the AP group, the mucosal sucrase activity was found to be significantly lower compared to controls (P ≤ 0·05). The 4-week administration of the A and AP diets to rats evoked a significant decrease in the pH of the caecal and colonic digesta compared with the C diet. In addition, the lowest colonic pH value was observed for the AP dietary treatment (P ≤ 0·05 v. C and A). After administration, both the processed and unprocessed apple pomace increased the relative caecal mass (tissue and digesta) and decreased the caecal ammonia and DM concentration, as compared to the C group (P ≤ 0·05).
Table 4 Body weight (BW), diet intake, nitrogen excretion patterns and gastrointestinal tract parameters in rats fed with the experimental diets
(Mean values with their standard errors)
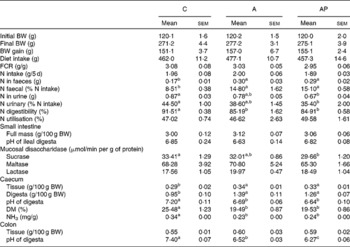
C, control; A, diet supplemented with 14 % of processed apple pomace; AP, diet supplemented with 15 % unprocessed apple pomace; FCR, feed conversion ratio.
a,b,c Mean values within a row with unlike superscript letters were significantly different (P ≤ 0·05).
The activity of bacterial β-glucuronidase in the caecal digesta of control rats was found to be higher than in other groups (P ≤ 0·05), in addition to there being a significantly higher activity of bacterial β-glucosidase compared to group A (Table 5). The dietary supplementation from apples did not significantly affect the activity of bacterial α-, β-galactosidase or α-glucosidase in the caecum. The total caecal concentration of SCFA in rats fed with the A and AP diets was significantly higher than in the C group, this resulting mainly from the increased concentration of acetic and butyric acids (P ≤ 0·05 v. control group). The highest concentration of propionic acid was found in group AP (P ≤ 0·05 v. group C). The dietary A and AP treatments also significantly decreased the concentration of branched-chain fatty acids, i.e. iso-butyric and iso-valeric acids, in comparison to group C, whereas for valeric acid, a significant difference was confirmed between groups C and A. The profile analysis of three major fatty acids acetic acid:propionic acid:butyric acid revealed that both dietary treatments with the apple pomaces were characterised by a significantly lower propionic acid:total SCFA ratio, compared to the controls. The addition of processed apple pomace in diet A increased the proportion of butyric acid (P ≤ 0·05 v. C) significantly. The size of the total SCFA pool (as well as acetic, propionic and butyric acid pools) in the A and AP groups was significantly higher than in the C group. The pools of iso-butyric and iso-valeric acids in the C group turned out to be significantly higher than in group A.
Table 5 Bacterial enzyme activity, as well as concentration, pool and profile of SCFA in the caecal digesta
(Mean values with their standard errors)
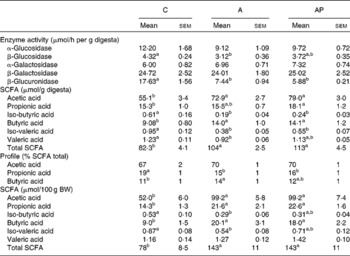
C, control; A, diet supplemented with 14 % of processed apple pomace; AP, diet supplemented with 15 % unprocessed apple pomace; BW, body weight.
a,b Mean values within a row with unlike superscript letters were significantly different (P ≤ 0·05).
Table 6 Indices of antioxidant status and lipid metabolism in rats fed diets supplemented with apple pomaces
(Mean values with their standard errors)
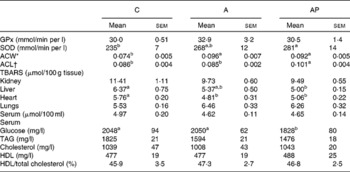
C, control; A, diet supplemented with 14 % of processed apple pomace; AP, diet supplemented with 15 % unprocessed apple pomace; GPx, glutathione peroxidase; SOD, superoxide dismutase; ACW, antioxidant capacity of water-soluble substances in the serum; ACL, antioxidant capacity of lipid-soluble substances in the serum; TBARS, thiobarbituric acid-reactive substances.
a,b Mean values within a row with unlike superscript letters were significantly different (P ≤ 0·05).
* μmol ascorbic acid equivalents/ml of serum.
† μmol Trolox equivalents/ml of serum.
Out of all groups assayed, the AP group was characterised by the highest activity of superoxide dismutase in a haemolysate of erythrocytes (P ≤ 0·05 v. group C) (Table 6). The serum antioxidant capacity of water-soluble substance value in rats fed with the A and AP diets was significantly increased compared to group C (P ≤ 0·05). The rats of group AP fed with a diet containing unprocessed apple pomace were characterised by a significantly higher serum antioxidant capacity of lipid-soluble substance value compared to groups C and A. The concentration of TBARS was significantly diminished in the livers of rats fed with the AP diet compared to the controls, whereas in the heart muscle it was significantly lower in groups A and AP v. group C. The control group and group fed with the diet containing processed apple pomace were characterised by significantly higher blood serum glucose levels compared to the rats fed with a diet with a higher contribution of the polyphenolic fraction from unprocessed apple pomace.
Discussion
Results obtained in the present experiment demonstrate that animal growth and feed utilisation in the groups fed on diets containing both processed and unprocessed apple pomace were similar to those of the control group. A change in the route of N excretion was however observed in groups fed on diets containing both apple preparations, typical of when dietary fibre is present and fermented in the large intestine. The difference lies in a significant increase in the bulk of N excreted with faeces and diminished bulk of N excreted with urine. In relation to the hypothesis, the dietary content of 0·018 % polyphenolic fraction in the unprocessed apple pomace did not affect the utilisation of dietary protein. Other reported studies have similarly not shown any negative effects either on diet intake or on body weight gains of experimental rats when 10 % lyophilised polyphenolic-rich cider apple preparations were added to the diet, resulting in 0·07 % dietary content of polyphenolic (mainly procyanidins and catechin) fraction(Reference Aprikian, Duclos and Guyot15). A 3-month-long toxicological study demonstrated that, in rat tissue, there were no clinical, haematological or histopathological changes on administration of 2 g/kg dose (equivalent to the consumption of over 270 apples a day by an average adult) of a polyphenolic extract (63·8 % of procyanidins) from unripe apples(Reference Shoji, Akazome and Kanda16). Some authors have however indicated the negative effect of administering high doses of polyphenols (i.e. epicatechins from green tea, anthocyanins from chokeberry and honeysuckle) in diets on experimental animals, young ones in particular(Reference Frejnagel and Juśkiewicz8).
Concerning the accepted hypothesis of the work, the higher content of dietary polyphenols in unprocessed apple pomace was found to decrease the activity of mucosal sucrase in the small intestine. However, these differences were within normal physiological ranges, without any negative effects on the growth of the animals. It is common knowledge that polyphenolic compounds may contribute to reducing the activity of endogenous enzymes in the small intestine, as has been reported in other studies, in which different classes of polyphenols (flavonols and phenolic acids) were used(Reference Juśkiewicz, Zduńczyk and Żary-Sikorska6, Reference Ramachandra, Shetty and Salimath17). This inhibitory effect of dietary polyphenols on mucosal disaccharidases activity is especially desirable if the organism carries a risk of diabetes, in which the reduced absorption of monosaccharides may influence the regulation of blood glucose levels(Reference Juśkiewicz, Zduńczyk and Jurgoński18). The tested apple pomaces were found to beneficially reduce the caecal, colonic and faecal pH values, wherein this should be linked with the physiological action of the preparations' fibre fraction during the course of the gut fermentation. With reference to the proposed hypothesis, the increased content of dietary polyphenols did not make the digesta or faeces alkaline, as was observed in our previous studies with grapefruit and blue-berried honeysuckle polyphenolic extracts(Reference Frejnagel and Juśkiewicz8, Reference Zduńczyk, Juśkiewicz and Estrella9); this indicated that the fermentation is indeed proceeding on dietary unprocessed apple pomace supplementation, as it should in the distal segments of the gastrointestinal tract. In the present study, both dietary apple preparations significantly suppressed bacterial β-glucuronidase activity in faeces and caecal digesta. On the addition of the processed apple pomace, a small inhibition of caecal β-glucosidase activity was observed, as compared to the control dietary treatment. Both dietary fibre and polyphenols present in the apple preparations may play positive roles in minimising the risk of colonic diseases through inhibiting the enzymic activity of undesirable intestinal microbiota(Reference Ohkami, Tazawa and Yamashita19, Reference Krupa-Kozak, Juśkiewicz and Wronkowska20). Studies performed on rats with azoxymethane-induced colon cancer revealed that pectins, constituting approximately 50 % of the dietary fibre fraction of apples, suppressed the activity of bacterial tryptophanase, β-glucuronidase and β-glucosidase to a considerable extent(Reference Ohkami, Tazawa and Yamashita19). The effect of polyphenolics on the glycolytic activity of intestinal microbiota may however vary, ranging from enhancing through having no effect to inhibiting, and the mechanism and dependence on other factors have not yet been properly recognised(Reference Frejnagel and Juśkiewicz8, Reference Biedrzycka and Amarowicz21).
The presence of apple pomaces in the experimental diets exerted trophic effects shown by the increased mass of the wall and digesta of the caecum; in relation to the hypothesis, additional trophic effects of apple polyphenols in the caecum were however not observed. It has been reported that simultaneous intake of high amounts of flavonoids and fibre with prebiotic properties normalises the course of fermentation in the large intestine, which may be disrupted by overdosing with the polyphenolic fraction in a given diet(Reference Zduńczyk, Juśkiewicz and Estrella9). Our study demonstrated that diets containing preparations from apples beneficially decreased the concentration of caecal ammonia; with reference to the proposed hypothesis, the actual content of polyphenolics in the diet was not a decisive factor influencing the level of this marker. The balance between ammonia production and absorption by bacteria and intestinal cells is vital for the ammonia concentration, which may change the metabolism and morphology of the intestinal epithelium, thus leading to carcinogenesis(Reference Juśkiewicz, Zduńczyk and Matusevicius22). It is assumed that the concentration and, in some cases, the pool of SCFA are reliable markers of the extent of fermentation occurring in large intestines by colonising microbiota(Reference Juśkiewicz, Zduńczyk and Jankowski23). In our study, the parameters of individual SCFA depicted an almost identical susceptibility to fermentation for both unprocessed and processed pomaces. Both apple pomaces used in the present experiment significantly increased the concentration and pool of butyric acid, which has an impact on the health and on the cancerous cells of the colon epithelium, and are currently under intensive study(Reference O'Keefe, Ou and Aufreiter24). It is speculated that one of the properties of butyric acid and some of its derivatives is the inhibition of histone deacetylation, which is closely related to suppression of carcinogenesis in the colon, whereas in this respect, acetic acid appeared to be inactive and propionic acid revealed only a medium inhibition of histone deacetylase activity(Reference Waldecker, Kautenburger and Daumann25).
The antioxidant capacity of apples arises from the presence of a number of compounds possessing antioxidant properties, including polyphenols and ascorbic acid(Reference Maffei, Tarozzi and Carbone26). In apple peel, which contains about five times more polyphenols than pulp, the main antioxidant compounds include flavonols, flavanols and procyanidins, which may together contribute to about 90 % of the total antioxidant capacity of the apple(Reference Biedrzycka and Amarowicz21). Unrefined dietary fibre may also affect the biological properties of polyphenolic fractions, this being, amongst others, a factor preventing their digestion in the gastrointestinal tract(Reference Lopez-Oliva, Agis-Torres and Goni4), thus providing the basis for studies on the antioxidant properties of fibre–phenolic complexes of apples(Reference Maffei, Tarozzi and Carbone26). The present study shows that both apple preparations increased the activity of superoxide dismutase in blood and a significant difference was noted for the diet containing 0·018 % of polyphenols. Similar changes were recorded in the case of the antioxidant capacity of the water fraction of serum. A significant increase in the antioxidant capacity of the lipid fraction of serum was recorded after diet supplementation with AP, which had introduced the highest amount of polyphenolics to the diet. This effect was not observed for diet A. The above-mentioned results support the accepted hypothesis that removing, through ethanolic extraction, a large part of the polyphenolic fraction of dietary apple pomace may further affect the systemic response of the body, e.g. the antioxidant status. Other studies have demonstrated that supplementation with a polyphenolic fraction at a 0·01 % level of a diet corresponds to a daily intake of four 200 g apples with a low polyphenol content or of one apple with a high content of this fraction by an adult person with a body mass of 60 kg(Reference Vrhovsek, Rigo and Tonon27). In the present study, the addition of both apple pomaces to the diet decreased the concentration of TBARS in the heart muscle, whereas the dietary addition of the unprocessed preparation significantly reduced the level of TBARS in the liver. These results only partly support the accepted hypothesis and indicate the beneficial physiological effect of both dietary fibre and polyphenols from apples, which may confirm suggestions of other authors on the beneficial effect of fibre–polyphenolic complexes consumed with diet(Reference Zduńczyk, Juśkiewicz and Estrella9, Reference Aprikian, Duclos and Guyot15). The administration of apples in the diet for rats with induced diabetes caused increased protection against lipid oxidation and consequently lowered the concentration of malondialdehyde in their urine and heart muscle(Reference Heo and Lee28). A lowered TBARS level in blood serum and urine was also noted for healthy animals as a result of apple ingestion(Reference Pajk, Rezar and Levart29).
In relation to the hypothesis, our study has also shown that, different from the processed apple pomace, a dietary addition of unprocessed pomace contributing 0·018 % of the polyphenolic fraction to the diet decreased the concentration of serum glucose in rats. This is indicative of the hypoglycaemic properties of apple polyphenols, and one of the potential causes of such an effect may be the lower activity of mucosal sucrase in the small intestine determined in the AP group. Furthermore, other research indicates that the inhibitory activity of endogenous disaccharidases forms one of the most important pathways of the hypoglycaemic effect of dietary quercetin and its glycosides in diabetic rats(Reference Ramachandra, Shetty and Salimath17). The lowering of blood glucose levels as a result of apple consumption has also been confirmed in human studies(Reference De Oliviera, Sichieri and Moura30).
Conclusions
It can be concluded that apple products added in diets for rats (i.e. as unprocessed and processed apple pomaces) exerted beneficial effects on the antioxidant status of the rat bodies, as well as on the blood lipid profile and fermentation in distal sections of their gastrointestinal tract. Concerning the accepted hypothesis of the work, the relatively high presence of the polyphenolic fraction in the unprocessed preparation did not affect the growth of animals or the extent of caecal fermentation, and more favourably modified the parameters of the organism's antioxidant status and blood glucose level compared to apple pomace extracted with ethanol.
Acknowledgements
J. Ju., E. Ż.-S. and Z. Z. were involved in the conception of the study; B. K. prepared the apple preparations and their chemical characteristics; J. Ju., E. Ż.-S., J. Ja. and A. J. were involved in the collection and assembly of data and in the analysis of physiological response; J. Ju. and E. Ż.-S. prepared the manuscript and performed the statistical analyses. Critical revision of the text was completed by all the authors. The authors extend their deepest gratitude to Feed for Health, COST Action FA 0802 (http://www.feedforhealth.org). The present study received no specific grant from any funding agency in the public, commercial or not-for-profit sectors. The authors declare no conflicts of interest.