Several lines of evidence suggest that oxysterols (oxidised cholesterol) are atherogenic and play a role in the pathogenesis of CVD. Plant sterols are structurally related to cholesterol( Reference Katan, Grundy and Jones 1 ), and the presence of one or more unsaturated bonds also makes the plant sterols susceptible to oxidation. Only small amounts of oxidised plant sterols (oxyphytosterols) can be found in the diet( Reference Normen, Johnsson and Andersson 2 , Reference Grandgirard, Martine and Joffre 3 ). Nevertheless, relatively high concentrations of oxyphytosterols are present in the serum of sitosterolaemic patients( Reference Plat, Brzezinka and Lutjohann 4 ) and smaller amounts in the plasma of healthy individuals( Reference Grandgirard, Martine and Demaison 5 , Reference Husche, Weingärtner and Pettersson 6 ). The fact that oxyphytosterol concentrations are high in sitosterolaemic patients, who also have severely elevated plasma plant sterol concentrations, may suggest that higher plasma plant sterol concentrations translate into higher plasma oxyphytosterol concentrations. Indeed, Husche et al. ( Reference Husche, Weingärtner and Pettersson 6 ) have recently shown that consumption of a plant sterol-enriched margarine for 4 weeks increases serum 7β-OH-campesterol concentrations in healthy individuals. Factors that are related to the oxidative behaviour of plant sterols are unknown. For cholesterol, however, it has been suggested that patients characterised by oxidative stress such as type 2 diabetics( Reference Szuchman, Aviram and Musa 7 ) and patients with stable coronary artery disease( Reference Rimner, Al Makdessi and Sweidan 8 ) have increased oxysterol concentrations.
If anything, endogenous oxidation of plant sterols is probably very low. This, in combination with the low serum plant sterol concentrations, makes it difficult to experimentally show the effects of endogenously formed oxyphytosterols on lesion formation. Except for studying the in vitro effects of oxyphytosterols, feeding oxyphytosterols, as has been done for oxysterols( Reference Staprans, Pan and Rapp 9 ), is another approach. Animal models have shown that oxyphytosterols are absorbed from the diet and transported to the lymph and accumulate in the serum, liver and aorta( Reference Tomoyori, Kawata and Higuchi 10 ). Furthermore, oxyphytosterols exhibit cytotoxic effects in cultured macrophages that are similar to those of oxysterols( Reference Adcox, Boyd and Oehrl 11 ). So far, there have been only limited data regarding the effects of an oxyphytosterol-enriched diet on atherogenesis( Reference Tomoyori, Kawata and Higuchi 10 , Reference Liang, Wong and Guan 12 ). However, from these animal studies, it is still unclear whether oxyphytosterols are atherogenic, as has been suggested for oxysterols. We, therefore, decided to investigate the effects of oxysterols and oxyphytosterols simultaneously on serum lipoproteins, inflammatory markers and atherosclerotic lesion development using heterozygous female LDL receptor-deficient (LDLR+/ −) mice.
Methods
Animal study
LDLR+/ − mice were bred in our laboratory by mating LDLR− / − males with C57BL6/J (Charles River Laboratories) females. For the experiment, thirty-six female LDLR+/ − littermates were used. LDLR+/ − mice were chosen to simulate a human model for mild hypercholesterolaemia. Female mice were used because they are more responsive to the development of diet-induced atherosclerosis. At the age of 5 weeks, these mice were divided over twelve cages, with three mice per cage. The mice were housed under standard conditions in wire-topped Macrolon type-I cages with a layer of maize fibres as bedding. All diets and water were given ad libitum. Until the start of the intervention study, the mice were fed a regular mouse diet (Hope Farms). The study was conducted in conformity with the Public Health Service Policy on Humane Care and Use of Laboratory Animals and approved by the Animal Ethics Committee of Maastricht University.
At the age of 8 weeks, a run-in period of 2 weeks started, during which all the mice received an atherogenic control diet. This diet contained, per 100 g, 17·2 g fat with a Western diet-like fatty acid profile (4·5 g palm oil, 1·7 g coconut oil, 4·0 g soya oil, 2·2 g olive oil and 4·8 g cacao oil), 47·1 g carbohydrate (37·1 g sucrose and 10·0 g maize starch), 20·0 g protein (casein), 0·25 g cholesterol, 0·25 g cholic acid, 0·20 g methionine, 4·85 g mineral mixture and 0·25 g vitamin mixture. After the run-in period, the mice in the twelve cages were randomly allocated to one of the three semi-synthetic test diet groups. For the next 35 weeks, the first group (n 12) was fed the atherogenic control diet. From this group, three mice died in weeks 2 (hydrocephalus), 16 (overgrown teeth) and 17 (unknown reasons). The second group (n 12) received the same atherogenic control diet except that 10 % of the added cholesterol was replaced by oxysterols (0·025 g/100 g diet). The third group (n 12) was given the same atherogenic diet, but now with 0·025 g oxyphytosterols (replacing cholesterol). For both oxysterols and oxyphytosterols, this means an approximate intake of 0·03 mg oxysterol or oxyphytosterol/g body weight per d. Oxysterols and oxyphytosterols were prepared by heating cholesterol or plant sterols at 180°C for 3 h (Raisio Group; Benecol Limited). In the oxysterol mixture and the oxyphytosterol mixture, 4·1 and 6·4 % of the sterols identified were still present in the non-oxidised form, respectively (Table 1). As has been indicated, there is a difference in the composition of the oxysterol and oxyphytosterol mixtures, which may in theory – if there is a difference in the potential atherogenicity of different oxy(phyto)sterol forms – have had a slight influence on the results of the study.
Table 1 Composition of the oxysterol and oxyphytosterol mixtures

Sampling of blood and tissues and determination of body weight
Fasting blood was sampled by retro-orbital bleeding under halothane anaesthesia at weeks 2, 7, 17, 27 and 37. Before sampling, the mice were weighed. Blood was collected into plastic tubes (Eppendorf AG) filled with glass beads to prepare serum. After the last blood sampling (week 37), all the mice were euthanised.
The entire heart (with aorta) of each mouse was dissected, sectioned perpendicularly to the heart axis just below the atrial tips and directly put into aluminium cryotubes (Omnilabo International) placed in dry ice-cooled isopentane. The cryotubes were stored at − 80°C until use.
Analysis of serum cholesterol and lipoprotein profiles
Directly after sampling, blood samples were analysed for serum total cholesterol concentrations using a commercially available enzymatic kit (CHOD/PAP method; Roche Diagnostics). The AUC was calculated for each mouse as an indicator of overall cholesterol exposure of the aorta during the 37-week experiment( Reference Rimner, Al Makdessi and Sweidan 8 ).
Lipoproteins were separated by means of fast protein liquid chromatography, and cholesterol concentration was quantified using an in-line detection system as described by Plat et al. ( Reference Plat, Beugels and Gijbels 13 ). Before the analysis, the sera of mice housed in the same cage were pooled.
Quantification of inflammatory marker concentrations
The concentrations of a panel of inflammatory markers (interferon-γ, IL-6, IL-10, IL-12, monocyte chemoattractant protein-1 (MCP-1) and TNF-α) were determined using the mouse inflammation cytometric bead array (Becton Dickinson Biosciences) according to the manufacturer's protocol. Briefly, 5 μl of the sample or the cytokine standard mixture were mixed with 5 μl of the mixed capture beads and 5 μl of the detection antibody-phycoerythrin reagent and incubated at room temperature for 2 h in the dark. Then, a two-colour flow cytometric analysis was performed using a FACScan® flow cytometer (Becton Dickinson Immunocytometry Systems). Before the analysis, equal volumes of sera from mice housed in the same cage were pooled. Data were acquired and analysed using the Becton Dickinson Cytometric Bead Array software (Becton Dickinson Immunocytometry Systems).
Quantification of atherosclerotic lesion size and severity and oxyphytosterol concentrations
After placing the frozen hearts on an object holder in the microtome/cryostat with the sectioned plane facing up, they were embedded in Tissue Tek (Sakura Finetek Europe BV). Subsequently, the frozen hearts were sectioned towards the aortic axis. Upon reaching the aortic root, serial cross-sections (7 μm thick) were made until the aortic valves disappeared. These sections were mounted onto microscope slides and air-dried on silica for 24 h. The slides were kept frozen ( − 80°C) until staining. Atherosclerotic lesion size was analysed for each mouse using four serial sections with a 42 μm interval. The collected sections were stained with toluidine blue and digitally photographed (Nikon DXM1200) and quantified using the digital image software (Adobe Photoshop CS3). Atherosclerotic lesion severity was quantified using the same four sections used for size determination. The following three types of lesions were discerned: (1) mild lesions – fatty streaks containing only foam cells; (2) moderate lesions – characterised by the additional presence of a collagenous cap; (3) severe lesions – involvement of the media and increased fibrosis, cholesterol clefts and/or necrosis of the plaque. After scoring each mouse individually, the percentages of lesions classified as mild, moderate and severe were calculated for each diet group.
The quantification of oxyphytosterol concentrations in aortic lesions was performed essentially following a protocol the same as that described for the analysis of those in the serum( Reference Husche, Weingärtner and Pettersson 6 ). For this purpose, three to five aortic sections were combined, weighed and extracted. The measured amounts of oxyphytosterols were normalised to the amount of aortic tissue.
Quantification of collagen content and apoptosis in atherosclerotic lesions
Sirius red staining was performed to visualise the collagen content per lesion area. For each mouse, one section was used. All the stained sections were photographed under the same conditions with a digital microscope camera (Nikon DXM1200). To quantify the proportion of collagen within the lesions, the lesion area was selected manually and a colour range selection was applied to measure the collagen content (Adobe Photoshop CS3). The same colour settings were used for all the sections. For the quantification of macrophages, lesions from the aortic root were fixed in acetone and incubated with antibodies against macrophages (MOMA-2, a gift from G. Kraal) as described by Goossens et al. ( Reference Goossens, Gijbels and Zernecke 14 ).
The number of apoptotic cells per lesion area was quantified using the terminal deoxynucleotidyl transferase-mediated dUTP nick end labelling assay (TUNEL) technique. For each mouse, one section was used. Only those TUNEL-positive nuclei that displayed morphological features of apoptosis, including cell shrinkage, aggregation of chromatin into dense mass and nuclear formation, were counted.
Statistical analyses
Differences between the three diet groups were tested for statistical significance using the non-parametric Kruskal–Wallis test. When a significant diet effect was found, pairwise comparisons were made using the non-parametric Mann–Whitney test. Values are presented as medians (with ranges). Differences in atherosclerotic lesion severity were tested using the χ2 test. Differences between the groups were considered as statistically significant at P< 0·017. Statistical analyses were performed using SPSS 11.0 (version 11.0.3; SPSS, Inc.) for MacIntosh OS X (version 10.3.9; Apple Inc.).
Results
Effects of oxysterol and oxyphytosterol consumption in the LDL receptor-deficient+/−mice
Body weight and cholesterol and lipoprotein profiles
At the end of the experiment, body weight (P= 0·867; Table 2) and serum cholesterol concentrations (P= 0·843) of the LDLR+/ − mice were similar in the three diet groups. In addition, no differences were found in the cholesterol AUC values (P= 0·163), indicating that the overall cholesterol exposure of the aorta was similar for all the diet groups. Lipoprotein profiles also did not differ (data not shown).
Table 2 Effects of oxysterols and oxyphytosterols on body weight, serum cholesterol and inflammatory marker concentrations, cholesterol exposure, and collagen and apoptotic cell contents at the end of the study (Median values and ranges)
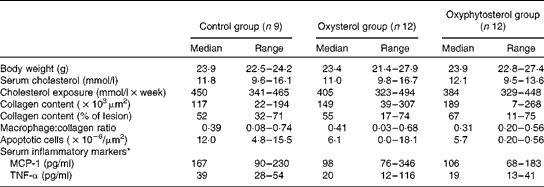
* Before the analyses, equal volumes of sera from mice housed in the same cage were pooled.
During the experiment, changes in serum total cholesterol concentrations did not differ between the three diet groups, except at weeks 7 and 17. Compared with the control diet, the oxyphytosterol diet significantly lowered serum total cholesterol levels at week 7 by 13 % (P= 0·006) and at week 17 by 12 % (P= 0·001). Serum total cholesterol concentrations in the oxysterol group followed the same pattern, but no significant differences were found when compared with the control group.
Atherosclerotic lesion size and severity
Although at the end of the experiment, lesion size seemed to be more pronounced in the oxysterol and oxyphytosterol groups than in the control group, differences between the three diet groups did not reach statistical significance (P= 0·420; Fig. 1). The proportion of severe atherosclerotic lesions, however, was significantly higher after oxysterol (P= 0·004) and oxyphytosterol (P= 0·011) diet consumption than after control diet consumption (Fig. 2). Proportions between the oxysterol and oxyphytosterol groups did not differ significantly (P= 0·125).

Fig. 1 Effects of oxysterol- and oxyphytosterol-enriched diets on atherosclerotic lesion size in female LDL receptor+/ − mice. Values are medians and ranges.
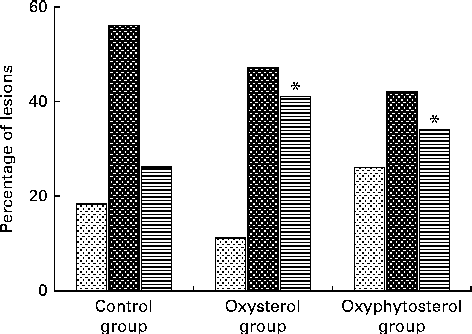
Fig. 2 Effects of oxysterol- and oxyphytosterol-enriched diets on atherosclerotic lesion severity in female LDL receptor+/ − mice. Values are presented as medians. Lesions were categorised as mild (), moderate (
) and severe (
). Differences in atherosclerotic lesion severity were tested using the χ2 test. * Median values were significantly different from those of the control group (P< 0·017).
Oxyphytosterol amounts within the atherosclerotic lesions
Within the atherosclerotic lesions, the amounts of oxyphytosterols in the mice fed the oxyphytosterol diet were higher (P< 0·017) than those in both the oxysterol and control groups (Fig. 3). All oxyphytosterols examined (7β-OH-sitosterol and 7β-OH-campesterol, 7-keto-sitosterol and 7-keto-campesterol, and 7α-OH-sitosterol and 7α-OH-campesterol) exhibited essentially the same pattern.

Fig. 3 Effects of oxysterol- and oxyphytosterol-enriched diets on oxyphytosterol concentrations (ng) in the atherosclerotic lesions of female LDL receptor+/ − mice. * Median values were significantly different from those of the control and oxysterol groups (P< 0·017). Amounts are reported in ng/7 μm-thick section. □, Control group; , oxysterol group; ■, oxyphytosterol group.
Collagen content and macrophages and apoptotic cell number in the atherosclerotic lesions
Collagen content and relative collagen content (expressed as percentage of lesion size) were comparable (P= 0·675 and 0·377, respectively) between the three diet groups (Table 2). No significant differences were found in the macrophage:collagen ratio and in the number of apoptotic cells in the atherosclerotic lesions between the diet groups (P= 0·301).
Inflammatory marker concentrations
At the end of the experimental period, serum concentrations of MCP-1 (P= 0·472) and TNF-α (P= 0·232) were similar between the diet groups (Table 2). Those of interferon-γ, IL-6, IL-10 and IL-12 were below the detection limits, and therefore they are not reported.
Discussion
Oxysterols are present in human plasma and are increased in type 2 diabetics( Reference Szuchman, Aviram and Musa 7 ) and patients with stable coronary artery disease( Reference Rimner, Al Makdessi and Sweidan 8 ). Likewise, oxyphytosterols are found in human plasma( Reference Husche, Weingärtner and Pettersson 6 ), although at much lower concentrations. Less is known, however, about the potential adverse health effects of oxyphytosterols. We found that consumption of oxyphytosterols – similar to that of oxysterols – increased the proportion of severe atherosclerotic lesions in female LDLR+/ − mice. Interestingly, without claiming causality, the concentrations of oxyphytosterols in the lesions of the oxyphytosterol diet-fed group were higher than those in the lesions of the oxysterol diet- and control diet-fed groups.
So far, only a few animal studies have examined the atherosclerotic potential of oxysterols or oxyphytosterols. Staprans et al. ( Reference Staprans, Pan and Rapp 9 ) found significant increases in lesion size after 4 months in apoE− / − mice fed an oxysterol-enriched diet when compared with those fed a cholesterol-enriched diet. These effects were not strain dependent, as in the LDLR− / − mice, an oxysterol diet also significantly increased the lesion size after a 7-month intervention. On the other hand, Ando et al. ( Reference Ando, Tomoyori and Imaizumi 15 ) observed no significant differences in lesion size after 8 weeks of feeding a control, cholesterol or oxysterol diet to apoE− / − mice, which may have been related to the relatively short duration of the study. So far, only two studies have examined the potential atherogenicity of oxyphytosterols( Reference Tomoyori, Kawata and Higuchi 10 , Reference Liang, Wong and Guan 12 ). Tomoyori et al. ( Reference Tomoyori, Kawata and Higuchi 10 ) found no significant differences in lesion size after 9 weeks of feeding a plant sterol or an oxyphytosterol diet to apoE− / − mice. It should be noted, however, that the effects of oxyphytosterols were compared with those of phytosterols and not with those of an atherogenic control diet, making it difficult to compare these results with our findings. In addition, the duration of that study was substantially shorter than that of the present study. Recently, Liang et al. ( Reference Liang, Wong and Guan 12 ) have shown in hamsters that diets enriched with the oxidation products of sitosterol or stigmasterol did not lower serum total cholesterol and LDL-cholesterol concentrations when compared with their non-oxidised counterparts. The oxidised products also did not affect atherosclerotic plaque size and cholesterol accumulation in the aorta when compared with those of the group that received the non-oxidised plant sterols.
There are several potential mechanisms by which oxy(phyto)sterols may promote the development of atherosclerosis. Among others, these components may modulate lipid homeostasis, trigger cell death or activate oxidation and inflammation. Concerning the effects on lipid homeostasis, this cannot explain the present results, since cholesterol exposure time did not differ between the three groups. In vitro experiments have shown that oxysterols trigger cell death in many different cell types, such as endothelial cells( Reference Sevanian, Hodis and Hwang 16 ), macrophages( Reference Clare, Hardwick and Carpenter 17 ), smooth muscle cells( Reference Peng, Tham and Taylor 18 ) and lymphocytes( Reference Christ, Luu and Mejia 19 ). Oxyphytosterols have also been shown to exhibit cytotoxic effects in cultured macrophages( Reference Adcox, Boyd and Oehrl 11 ). Besides apoptosis, oxysterols can also induce necrosis in various cell types, especially at higher concentrations( Reference Vejux and Lizard 20 ). Furthermore, it has been suggested that the cytotoxic characteristics of an oxysterol mixture depend on the oxysterols in the mixture, their levels and their relative proportions( Reference Vejux and Lizard 20 ). Since it is unknown how these in vitro findings translate to the in vivo situation, this may explain the lack of the effect on the apoptotic cell content in the present animal study. Furthermore, both in vitro and animal studies have shown that oxysterols activate oxidative processes and inflammation. In our cell experiments using HCAEC also, oxyphytosterols increased the production of MCP-1, a marker of endothelial activation. However, serum levels of TNF-α and MCP-1 were not significantly changed in the present study. Clearly, more research is needed to elucidate the potential atherogenic mechanism of oxy(phyto)sterols in vivo.
In the present study, the increased proportion of severe atherosclerotic lesions did not parallel the differences in serum total cholesterol levels, total cholesterol exposure or lipoprotein profiles. Consumption of oxyphytosterols, however, resulted in a significant reduction in serum total cholesterol concentrations at weeks 7 and 17. Although oxysterol consumption resulted in the same pattern, differences did not reach statistical significance. Staprans et al. ( Reference Staprans, Pan and Rapp 9 ) also observed no significant differences in serum cholesterol concentrations between the apoE− / − mice fed a control diet and those fed an oxysterol diet. However, in the LDLR− / − mice, serum total cholesterol concentrations were significantly reduced by the oxysterol diet when compared with the control diet. The study of Ando et al. ( Reference Ando, Tomoyori and Imaizumi 15 ) also suggested that dietary oxysterols and cholesterol had comparable effects on serum total cholesterol concentrations. Furthermore, Tomoyori et al. ( Reference Tomoyori, Kawata and Higuchi 10 ) found no significant differences in serum total cholesterol concentrations after 9 weeks of feeding a phytosterol or an oxyphytosterol diet. Thus, it is not clear whether oxysterols and oxyphytosterols affect serum total cholesterol concentrations.
It is well known that sterols, in general, are present in their oxidised form in Western diets. Various food products, such as dairy products, eggs, meat and fish, may contain some oxysterols( Reference Brown and Jessup 21 ). Food processing, especially heat treatment, drying and storage, can also induce oxidation. Oxyphytosterols have been identified in coffee beans( Reference Turchetto, Lercker and Bortolomeazzi 22 ), French fries( Reference Dutta 23 ), heated vegetable oils( Reference Dutta 23 ), infant milk formulas( Reference Zunin, Calcagno and Evangelisti 24 ), parenteral nutrition( Reference Plat, Brzezinka and Lutjohann 4 ) and potato chips( Reference Dutta 25 ). The dietary intake of oxyphytosterols from phytosterol-enriched foods or supplements is estimated to be relatively low ( ≤ 1·7 mg/d). Results from animal studies have suggested that an increased dietary intake of oxyphytosterols elevates their serum concentrations. Data regarding the relative absorption of oxyphytosterols are limited. Tomoyori et al. ( Reference Tomoyori, Kawata and Higuchi 10 ) observed a greater total lymph recovery of both oxidised campesterol (15·9 %) and β-sitosterol (9·1 %) than that of campesterol (5·5 %) and β-sitosterol (2·2 %) in lymph duct-cannulated rats fed diets containing cholesterol, plant sterols or their oxidised derivatives. There are, however, no data from human intervention studies that have quantified the absorption of dietary oxyphytosterols. Except from dietary sources, oxysterols can also be formed endogenously( Reference Breuer and Björkhem 26 ), and this may also be true for oxyphytosterols. An important question is whether in vivo-formed oxysterols and oxyphytosterols have atherogenic potential the same as that of their diet-derived counterparts that we used in the present study. To what extent oxyphytosterols are formed, metabolised and excreted in the human body has not been studied.
In the present study, oxysterol and oxyphytosterol mixtures were used. Whether all isoforms have similar effects is not known, but this certainly needs to be addressed in future studies. In this respect, recent initiatives to specifically synthesise individual oxyphytosterols from sitosterol and campesterol( Reference O'Callaghan, Foley and O'Connell 27 ) are a major step forward.
In summary, the present results indicate that not only dietary oxysterols but also dietary oxyphytosterols increase the proportion of severe atherosclerotic lesions. This suggests that plant sterols when oxidised may increase atherosclerotic lesion severity instead of lowering the size and severity of lesions when fed in their non-oxidised form. Therefore, this novel finding might give an indication as to where to find the answer in the current hot debate about the potential atherogenicity of plant sterols. However, to what extent these results can be extrapolated to the human situation warrants further investigation.
Acknowledgements
We thank the technical staff from our departments for their support. We also thank the Raisio Group, Benecol Limited for kindly providing the oxy(phyto)sterols to prepare the mouse diets. The present study was financially supported by the Netherlands Organization for Scientific Research (Program Nutrition: Health, Safety and Sustainability; grant 014–12-010). The authors' contributions are as follows: E. T. performed the study, analysed and interpreted the data, and wrote the manuscript; C. H. and D. L. contributed to the sterol analyses and the interpretation of the data, revised the previous manuscript versions, and revised and agreed upon the final manuscript; M. J. J. G., M. J., R. S.-S. and I. v. d. M. contributed to the lesion analysis, revised the previous manuscript versions, and revised and agreed upon the final manuscript; R. P. M. and J. P. contributed to the design of the study, the data analysis and the interpretation of the data, revised the previous manuscript versions, and revised and agreed upon the final manuscript. None of the authors has any conflicts of interest with regard to the organisation that had sponsored the research.