Introduction
A total of 690,000 ischemic strokes occur in the USA every year. Reference Lloyd-Jones, Adams and Brown1 Ischemic brain injury can be due to large artery atherosclerosis, cardioembolism, small vessel disease, or cryptogenic. In atherosclerosis, carotid bifurcation plaques are particularly prone to pose a risk for transient ischemic attacks (TIAs), amaurosis fugax, and stroke. Atherosclerotic carotid disease is thought to account for 173,000 (approximately 25%) of all yearly cases of ischemic stroke in the USA. Reference Lloyd-Jones, Adams and Brown1
Consensus guidelines exist concerning the degree of stenosis above which carotid endarterectomy (CEA) or stent placement should be considered in order to prevent future ischemic events. The North American Symptomatic Carotid Endarterectomy Trial (NASCET) demonstrated a benefit from CEA in patients with symptomatic 70%–99% internal carotid artery (ICA) stenosis which can also be found in the latest American Heart Association (AHA)/American Stroke Association Guidelines for the prevention of stroke in patients with stroke and TIA. Reference Hosseini, Kandiyil, Macsweeney, Altaf and Auer2,Reference Kernan, Ovbiagele and Black3 The risk of ipsilateral stroke in the coming 5 years is significantly reduced with a risk reduction of 16% for patients presenting a >70% symptomatic stenosis. Reference Kwee, van Oostenbrugge and Prins4 On the other hand, in symptomatic patients presenting a 50%–69% stenosis, a lower risk reduction of 4.6% makes the necessity of CEA a subject of debate. Reference Kwee, van Oostenbrugge and Prins4 Concerning asymptomatic patients, only 10%–12% of the >50%–60% stenosis subgroup suffer from a symptomatic stroke in the 5 years following the detection of the stenosis. Reference Treiman, McNally, Kim and Parker5 In asymptomatic patients with >50% stenosis, 19 procedures are necessary to prevent a single future stroke. Reference Walker, Marler and Goldstein6 It has thus been questioned if all patients in the 50%–69% stenosis group, in particular asymptomatic patients, should benefit from surgical treatment, which is in itself not exempt from associated morbidity and mortality, and if cost-effectiveness is reasonable in this collective. Reference Altaf, Daniels and Morgan7 No benefit was so far believed to arise from CEA in patients with mild stenoses of <50%. Stroke in this patient cohort is sometimes referred to as “stroke of undetermined etiology” or “cryptogenic.” Reference Adams, Bendixen and Kappelle8 A quest for markers and identifiers of stroke risk other than stenosis degree in this patient group ensued.
Atheromatous plaque composition varies widely and is increasingly recognized to influence a plaque’s behavior, notably its risk of ulceration, appositional thrombus formation, and rupture with subsequent brain embolism. Histologically, eight types of atheromatous plaques have been described in a classification scheme proposed by the AHA with hemorrhagic and other vulnerable plaques falling into category VI. Reference Stary, Chandler and Dinsmore9 Whereas, 70%–80% of symptomatic patients with ≥50% carotid stenosis do not experience a recurrent stroke within 5 years, the stroke risk increases to 66% in patients with a vulnerable plaque. Reference Hosseini, Kandiyil, Macsweeney, Altaf and Auer2 Similarly to stable plaques, vulnerable plaques are typically located on the outer wall of the common carotid bifurcation or the proximal ICA, opposite to the carotid flow divider at a location of maximal oscillatory shear stress. Reference Treiman, McNally, Kim and Parker5 However, unlike stable plaques which are composed essentially of a fibrous or calcified matrix, vulnerable or unstable plaques feature components such as a large lipid or necrotic core, and may show fibrous cap rupture and internal hemorrhage. Reference Narumi, Sasaki and Natori10 Internal hemorrhage is the most straightforward of these three parameters to be estimated by imaging. Intraplaque hemorrhage (IPH) is believed to arise from the rupture of fragile and tortuous neovessels with blood ingress through a fissured plaque surface being an alternative etiological explanation. Reference Virmani, Kolodgie and Burke11 In general, IPH is thought to increase with age. Reference Kwee, van Oostenbrugge and Prins4 Other independent predictors of IPH are male gender and ulceration. Reference Treiman, McNally, Kim and Parker5 Histologically, IPH is associated with an increase in a plaque’s necrotic core size, general plaque progression, and further IPH in the form of ongoing low-grade bleeding. Reference Treiman, McNally, Kim and Parker5
Current treatment algorithms do not consider plaque composition as a criterion to commence treatment. A need has thus been recognized to search for the presence of hemorrhage in low-grade stenotic plaques in order to improve the selection of patients amenable to CEA. Reference Hosseini, Kandiyil, Macsweeney, Altaf and Auer2,Reference Bayer-Karpinska, Schwarz, Wollenweber, Poppert and Saam12,Reference Saba, Yuan and Hatsukami13 In a 2008 study by Altaf et al., Reference Altaf, Daniels and Morgan7 IPH was found to be a good predictor of ipsilateral stroke even in mild to moderate stenosis (30%–69%). The authors found a hazard ratio for ischemic events in this subgroup of 9.8. In the same study, the predictive value of luminal stenosis was much lower (hazard ratio, 1.5). Several other studies followed that compared IPH and stroke risk, Reference Hosseini, Kandiyil, Macsweeney, Altaf and Auer2 with seven cohort studies pooled in a recent meta-analysis from Schindler et al., investigating 1121 patient-years of 696 symptomatic and asymptomatic patients. Reference Schindler, Schinner and Altaf14
The purpose of our study was to use a new 3D TSE T1 fat-saturated black-blood MRI sequence to assess the percentage of hemorrhagic plaques in patients presenting with symptoms of brain ischemia, and to establish a relation between plaque hemorrhage, the degree of stenosis observed, and the prevalence of diffusion-positive infarction in these individuals.
Material and Methods
Patients
This retrospective study was conducted at a tertiary care university hospital with approval of the competent review board (Commission cantonale d’éthique de la recherche CCER, Geneva, Switzerland), document number 07-125, and in compliance with the principles of the Helsinki Declaration. Informed consent had been given by each patient.
A cohort of 62 symptomatic patients was included between January 2013 and October 2014. The inclusion criterion was admission to our institution with suspicion of TIA, amaurosis fugax, or acute stroke. Patients with possible other etiologies of stroke than embolism due to atherosclerosis, notably individuals where atrial fibrillation was found, were excluded. Almost all patients were initially examined, at the time of admission, with non-contrast computed tomography (NCCT) of the head, followed by a computed tomography angiogram (CTA) of the head and neck vessels, if no hemorrhage was identified. The magnetic resonance (MR) exam was performed up to 1 week after the CTA.
Image Acquisition and Analysis
In order to detect signs of IPH, a coronal 3D TSE fat-saturated black-blood T1 sequence (volume isotropic turbo spin-echo acquisition [VISTA]) was added to the routine MR protocol. The practical applicability of the related T1 spin-echo SPACE sequence had already been shown in the detection of arterial dissection. Reference Cuvinciuc, Viallon and Momjian-Mayor15 Our study differs thus from others having investigated hemorrhagic plaques where a 3D gradient echo T1 sequence or a 2D multi-planar technique were used. The technical parameters of our 3D spin-echo T1 sequence were as follows: repetition time, 350 ms; time to echo, 19.5 ms; field of view, 182 × 120 mm; scan length, 16 cm; acquisition voxel size, 0.5 × 0.5 × 0.8 mm; isotropic reconstruction voxel size, 0.4 × 0.4 × 0.4 mm; SPAIR fat saturation with TI, 120 ms; SENSE factor, 2 × 2; and acquisition time, 6 min 14 s. Axial diffusion-weighted imaging (DWI) was performed with b0 and b1000; slice thickness, 4 mm; in-plane resolution, 1.2 × 2.03 mm; SENSE acceleration factor 2; TR/TE, 3881/69 ms; slice number, 35; and field of view, 230 × 230 mm. The other brain imaging sequences included in our routine protocol were axial T2 fast spin-echo, axial T2 gradient echo, coronal fluid attenuation inversion recovery (FLAIR), and axial 3D time of flight (TOF) MR angiography of the carotid bifurcations. All MR studies were performed on a 3T MR scanner (Ingenuity TF PET/MR, Philips, Amsterdam, The Netherlands). No intravenous contrast medium was used.
The MR exams were read by a neuroradiologist (MS), blinded to patients’ clinical information, who screened for the event of acute infarction on DWI, defined as a region of increased signal on the b1000 image, with concurrent low values on the corresponding apparent diffusion coefficient (ADC) map. Patients with TIA and amaurosis fugax without a correlate on imaging were also included in the statistical analysis. Stenosis degree was determined by the same neuroradiologist, experienced with reconstructions, performing manual measurements according to the NASCET method, using the formula %ICA stenosis = (1 − [narrowest ICA diameter/normal diameter of distal cervical ICA]) × 100. The NASCET method has been validated for the quantification of carotid stenosis with a good correlation between millimeter stenosis assessment on angiography and percentage stenosis measurement on the CTA. Reference Bartlett, Walters, Symons and Fox16 This Bartlett et al. article also addresses the question of possibly fallacious measurements in cases of near-occlusion. Measures were performed on the CTA images acquired prior to the MR exam if available, otherwise on the MR exam itself. Carotid plaques were classified either as VISTA-positive (VISTA [+]) or VISTA-negative (VISTA [−]) based on the presence (VISTA [+]) or absence (VISTA [−]) of plaque hyperintensity on the 3D T1 sequence.
Statistical Analysis
Relationships between categorical variables were evaluated by the Chi-square test of independence. When more than 20% of cells had an expected value of less than 5, Fisher’s exact test was used instead. T-test was used to compare means of continuous variables. Levene’s test for equality of variances was performed and the significance level for the T-test was chosen accordingly depending on whether equality of variances between the two groups could be assumed or not. The effect of NASCET stenosis on VISTA status of a plaque and on ipsilateral core infarct was evaluated by logistic regression. In these two cases, a receiver operator characteristic (ROC) curve was derived. Logistic regression was also used to evaluate the effect of VISTA status of a plaque on the likelihood of ipsilateral core infarct. All statistical analyses were carried out on SPSS Version 22.0.0.0 (IBM Corporation, Armonk, NY, USA). A 0.05 significance level was used for all tests.
Results
Demographic data of the study population, including age, gender, and cardiovascular risk factors are shown in Table 1. Of note, age ranged from 37 to 89 years, and the mean age was 74 years. Twenty-six patients were female and 36 were male. The entire cohort was considered as a whole (n = 62) and also separately by VISTA (–) plaques bilaterally (n = 36) and at least one VISTA (+) plaque (n = 26). There was generally a similar distribution of all the variables across the two groups considered except for gender, with men being relatively more represented in the VISTA (+) group than women (73.1% vs. 47.2%, p = 0.042).
Table 1: Demographic data of the entire cohort of patients (n = 62) and separately by patients with VISTA (−) (n = 36) and VISTA (+) (n = 26) plaques
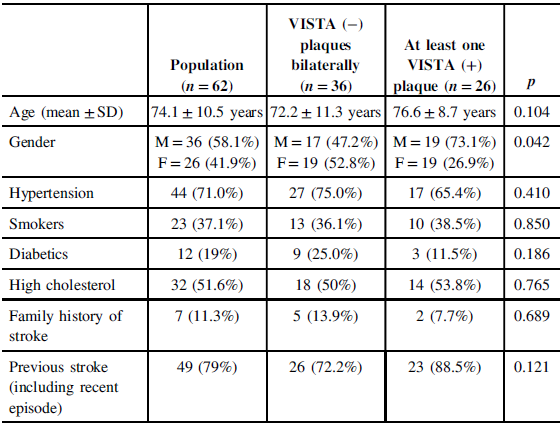
SD = standard deviation.
In the plaque-based analysis, a total of 114 plaques were found in the cohort of 62 symptomatic patients, 26 VISTA (+), and 88 VISTA (−) (Table 2). As there were no bilateral hemorrhagic plaques, patient-based prevalence of VISTA (+) plaques was thus of 42%. The overall plaque prevalence was 100% if ipsilateral plaques were counted. NASCET stenosis was significantly higher in the VISTA (+) than in the VISTA (−) groups (47.4% vs. 33.7%, p = 0.009).
Table 2: Cross-tabulation of recent ipsilateral DWI-positive core infarcts with VISTA (−) and VISTA (+) plaques status. VISTA (+) plaques correspond most likely to IPH and are significantly more associated with ipsilateral stroke than VISTA (−) plaques (p = 0.003). As an example, assuming independence between the two variables, the expected proportion of recent ipsilateral stroke caused by a VISTA (+) plaque should have been 6.0%, when the observed value was in fact 13.2%.

Chi-square = 8.993; p = 0.003.
Furthermore, logistic regression showed that when the degree of stenosis was used to predict the VISTA (+) status of a plaque, there was a significant relationship with odds ratio (OR) = 1.018 (95% CI [1.001, 1.034], p = 0.033). In other words, a 1% increase in NASCET stenosis implied an increase in the probability of VISTA (+) status by 0.018 or 1.8%. This was further demonstrated by the receiver operator characteristic (ROC) curve, shown in Figure 1, with area under the curve (AUC) significantly higher than 0.500 (p = 0.024).
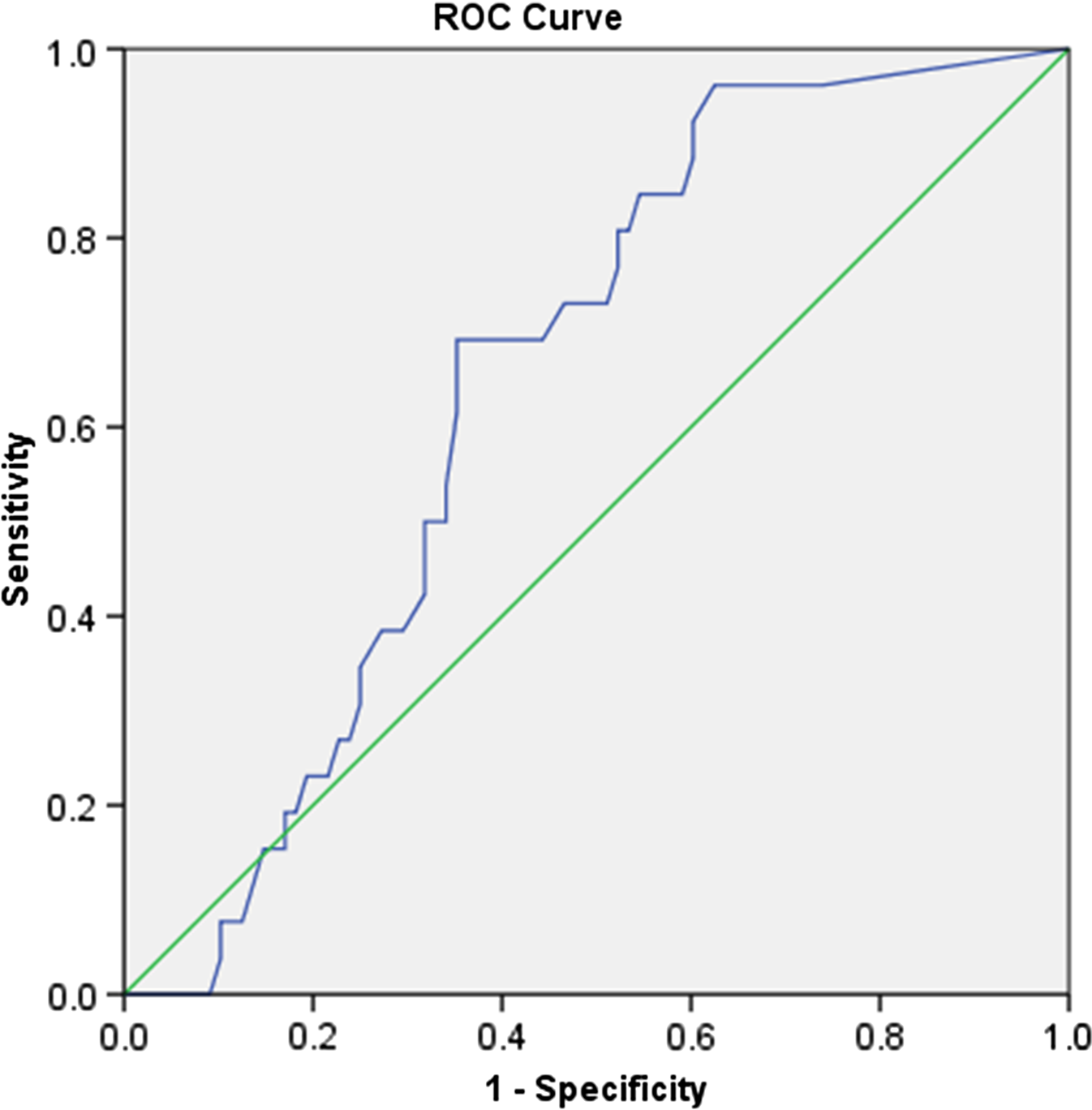
Figure 1: The ROC curve of plaque NASCET stenosis as a predictor of intra-plaque hemorrhage [VISTA (+) plaque]. AUC = 0.646, 95% CI [0.542, 0.750], p = 0.024.
VISTA (+) plaques were significantly more associated with ipsilateral stroke than VISTA (−) plaques (p = 0.003) as demonstrated in Table 2. As an example, assuming independence between the two variables, the expected proportion of recent ipsilateral stroke caused by a VISTA (+) plaque should have been 6.0%, when the observed value was in fact 13.2%.
The association between VISTA (+) plaques and ipsilateral stroke was further explored by logistic regression, which showed a statistically significant positive relationship with OR = 3.854 (95% CI [1.548, 9.592], p = 0.004). A similar logistic regression model also taking into account the degree of NASCET stenosis of the plaque still showed a significant result for VISTA (+) and ipsilateral stroke with OR = 3.275 (95% CI [1.290, 8.313], p = 0.035), confirming that a VISTA (+) plaque is more likely to cause ipsilateral stroke, independently of the degree of NASCET stenosis. It should be noted that an increase in the degree of NASCET stenosis is also associated with an increased likelihood of ipsilateral stroke with OR = 1.019 (95% CI [1.004, 1.034], p = 0.011) as confirmed by an ROC curve with AUC significantly higher than 0.500 (p = 0.009) (Figure 2). This was the case even when the effect of VISTA status of the plaque was taken into account with OR = 1.016 (95% CI [1.001, 1.032], p = 0.035), confirming that larger plaques are more likely to cause stroke, independently of their VISTA status.
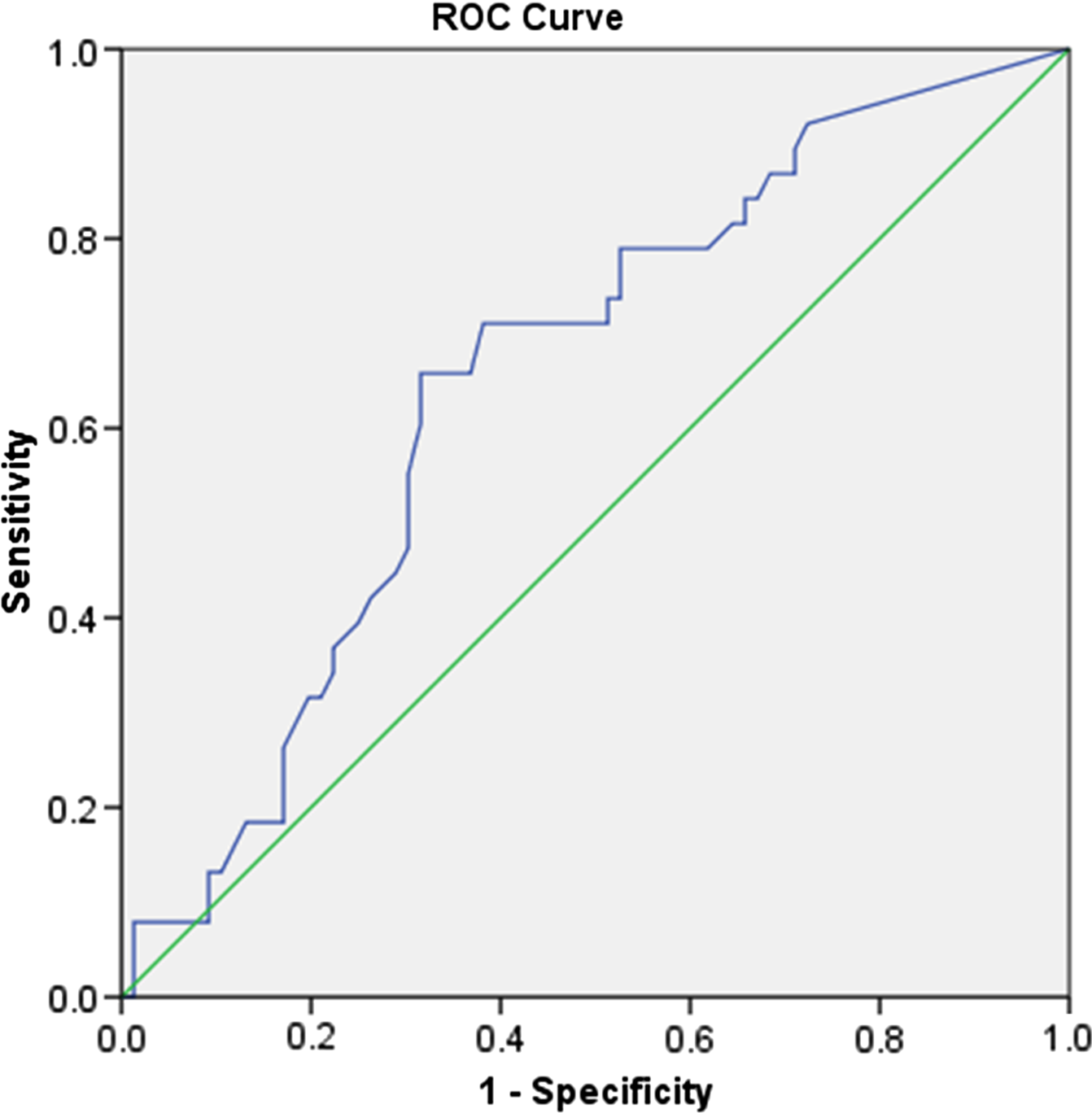
Figure 2: The ROC curve of plaque NASCET stenosis as a predictor of ipsilateral stroke. AUC = 0.650, 95% CI [0.546, 0.754], p = 0.009.
Thirty-six out of the 62 patients showed areas of restricted diffusion on the DWI imaging sequence, 2 of them both-sided, corresponding to areas of acute brain infarcts in 58% of the study population (Table 2, Figures 3c and 4c). All 36 patients with acute infarcts had a carotid bifurcation or proximal carotid artery plaque ipsilateral to the ischemic brain lesion (Figures 3a and 3b, 4a and 4b). Out of the 36 patients with evidence of an ischemic core infarct, 15 (42%) showed ipsilateral VISTA (+) carotid plaques on the fat-saturated 3D T1WI sequence of the neck vessels, either at the carotid bifurcation or at the proximal ICA (C1 segment) (Table 2). No ipsilateral carotid VISTA T1 hypersignal was detected in the remaining 21 patients (58%) with brain core infarct (Table 2). In the supposedly hemorrhagic plaque group of stroke patients, degrees of stenoses (ipsilateral to the infarct) were ≥70% in 13%, ≥60% < 70% in 27%, ≥50% < 60% in 13%, ≥40% < 50% in 27%, ≥30% < 40% in 0%, and <30% in 20% of patients. In 21 patients with acute brain infarcts but VISTA (−) ipsilateral carotid plaques, degrees of stenosis of 23 ipsilateral plaques were ≥70% in 26%, ≥60% < 70% in 9%, ≥50% < 60% in 13%, ≥40% < 50% in 9%, ≥30% < 40% in 9%, and <30% in 35% (Table 3, numbers rounded). In the entire VISTA (−) plaque group, including patients without core infarcts (88 plaques in total), the mean degree of stenosis was 34%. In both groups presenting acute infarction with an associated ipsilateral plaque, the mean degree of stenosis was not significantly different (p = 0.294), with 50% in the group with VISTA (+) plaques (n = 15), and 44% in the group with VISTA (−) plaques (n = 24). The mean degree of stenosis was thus not superior to 50% in both groups with close to half of patients falling into the group of mild or low-grade stenosis (<50%). In the remaining 26 patients without a DWI-positive lesion on MRI, 8 presented hemorrhagic plaques with a mean degree of stenosis of 38% (Figure 5). A total of 39 nonhemorrhagic plaques were identified in the 26 DWI-negative patients with a mean degree of stenosis of 37%.

Figure 3: CTA and VISTA MRI images illustrate stenosis of 60% measured according to NASCET criteria (A, B) of the left ICA with supposed IPH (B, asterisk) and ipsilateral frontal infarct on diffusion MRI image (C, arrow).

Figure 4: MRI images show stenosis of 40% of the right ICA (A, B) with supposed IPH on VISTA sequence (A, asterisk) as well as a small ipsilateral paraventricular infarct on diffusion MRI image (C, arrow).
Table 3: Table showing the relation of stenosis degree to VISTA (+) status of atheromatous plaques in stroke patients (numbers are rounded)


Figure 5: MRI images illustrate an 85% stenosis of the left ICA (A, B) with supposed IPH (B, asterisk). No core infarct was observed on the corresponding diffusion-weighted (C) and T2-weighted (D) MRI images.
Discussion
Similarly to classical risk factors (smoking, male gender, and hypertension), IPH has been recognized to be a strong predictor of cardiovascular events. Reference Hosseini, Kandiyil, Macsweeney, Altaf and Auer2 , Reference Gupta, Baradaran and Schweitzer17–Reference Deng, Mu and Yang21 It carries a higher predictive value for recurrence of ischemic events than any other clinical risk factor identified in the NASCET trial with a minimal hazard ratio of 4.6–4.7 in a meta-analysis from 2013. Reference Gupta, Baradaran and Schweitzer17 This was confirmed by the meta-analyses from Schindler et al. and Deng et al. from 2020 with a hazard ratio of 7.14 (95% CI [4.32, 11.82]) in the latter. Reference Schindler, Schinner and Altaf14,Reference Deng, Mu and Yang21
IPH can be detected by ultrasound with hemorrhagic plaques having a heterogeneous pattern containing anechoic components. Reference Bluth, Kay and Merritt22 However, although widely used, ultrasound is limited by subjectivity and may be challenging in the presence of calcifications. Reference Narumi, Sasaki and Natori10 In a study by Bluth et al., the sensitivity, specificity, and accuracy for the detection of IPH by ultrasound were 94%, 88 %, and 90%, respectively. Reference Bluth, Kay and Merritt22 Additionally, ultrasound has limited power in accurately identifying patients at low risk. Reference Hosseini, Kandiyil, Macsweeney, Altaf and Auer2 Computed tomography (CT) also did not prove so far to be sufficiently reliable for density-based detection of IPH. Reference U-King-Im, Fox and Aviv23
An emerging imaging alternative is heavily T1WI black-blood MR techniques. Black-blood plaque imaging can be performed using either 2D or 3D T1W1 sequences where hemorrhagic areas, enclosed by a thin fibrous cap, appear as increased T1 signal intensity, often crescent-shaped, as shown by several radiologic-histopathologic studies. Reference Treiman, McNally, Kim and Parker5 T1WI MR with fat saturation has been histologically proven to detect IPH with a sensitivity of 90%–100% and a specificity of 74%–80%. Reference Hosseini, Kandiyil, Macsweeney, Altaf and Auer2,Reference Chu, Kampschulte and Ferguson24 As opposed to 2D multi-planar sequences, 3D sequences have several advantages such as better contrast and spatial resolution, fewer partial volume and motion artifacts, and shorter acquisition time. They also have higher sensitivity and specificity for plaque characterization than 2D techniques, as shown in the histologic–radiologic study by Narumi et al. Reference Narumi, Sasaki and Natori10 with 34 patients. An advantage of the gradient echo-based Magnetization-Prepared – RApid Gradient Echo (MPRAGE) sequence over fast spin-echo and TOF T1 sequences was postulated in a 2010 publication by Ota et al. Reference Ota, Yarnykh and Ferguson25 The group of Sato et al., however, tested four different T1 sequences for the detection of hemorrhagic plaque and found a spin-echo sequence to be the most sensitive one. Reference Sato, Ogasawara and Narumi26
Clinically, IPH is related to previous cardiovascular events, and exposes the patient to a higher risk of future ischemic events, even in the context of a moderate overall degree of stenosis. Reference Hosseini, Kandiyil, Macsweeney, Altaf and Auer2,Reference Narumi, Sasaki and Natori10,Reference Chu, Kampschulte and Ferguson24,Reference Zavodni, Wasserman and McClelland27,Reference Qiao, Steinman and Qin28 As early as in 1979, and then in 1982, IPH had been linked to symptomatic behavior of carotid atheromatosis. Reference Lusby, Ferrel, Ehrenfeld, Stoney and Wylie29,Reference Sadoshima, Fukushima and Tanaka30 In the Hosseini et al. Reference Hosseini, Kandiyil, Macsweeney, Altaf and Auer2 study with individuals presenting ≥50% carotid stenosis, patients with IPH suffered 92% of all recurrent ipsilateral ischemic events (57/62 events in a total of 179 patients) and 25 out of 26 future strokes. At the same time, that group performed a meta-analysis of 335 patients where IPH was associated with a significant increase in the risk for recurrent ipsilateral ischemic events (OR = 12.2; 95% CI [5.5–27.1]). Similarly, in a 2008 study by Altaf et al., Reference Altaf, Daniels and Morgan7 13 out of 14 ischemic neurological events during the follow-up of 64 patients occurred in the patient group with IPH. Treiman et al. Reference Treiman, McNally, Kim and Parker5 suggested that 18% of individuals with IPH and ≥50% stenosis would develop symptomatic cerebral infarction, and 43% of individuals either symptomatic or asymptomatic cerebral infarction in the 48 months following the discovery of the plaque. The increased stroke risk associated with a hemorrhagic plaque has been estimated to last for up to 5 years. Reference Treiman, McNally, Kim and Parker5 The hazard ratio of stroke based on the presence of IPH on MRI was estimated at 5.2 by Treiman et al., and at 10.2 and 7.9 for symptomatic and asymptomatic patients, respectively, by Schindler et al. Reference Treiman, McNally, Kim and Parker5,Reference Schindler, Schinner and Altaf14 In the 2004 study by Chu et al., Reference Chu, Kampschulte and Ferguson24 IPH was detected in 77% of symptomatic plaques scheduled for CEA. Finally, in a 2012 study by Turc et al., Reference Turc, Oppenheim and Naggara31 the overall prevalence of recent IPH was 33% in symptomatic patients. Of note, the risk of further cerebral embolization is also higher during the CEA procedure itself with plaques featuring IPH. Reference Altaf, Beech and Goode32
In a comparison of specimens of patients having undergone CEA, symptomatic patients had a strong association with plaques containing IPH (71%), whereas the association proved to be weak in asymptomatic patients (12%) as shown by Spagnoli et al. Reference Spagnoli, Mauriello and Sangiorgi33 This difference seems to confirm the assumption that individuals with hemorrhagic plaques are at a higher risk of ischemic events, which is in agreement with the results from our study. Concerning the volume of IPH, no significant difference (p > 0.99) was found in a study of 43 symptomatic and asymptomatic patients having undergone CEA. Reference Hatsukami, Ferguson and Beach34 Accordingly, in the Lusby et al. Reference Lusby, Ferrel, Ehrenfeld, Stoney and Wylie29 study, IPH was found in a higher proportion of CEA specimens from symptomatic than from asymptomatic individuals. In the Polak et al. ultrasound study, there was an increased prevalence of supposed IPH in patients with carotid stenosis when patients were studied in proximity to the onset of symptoms. Reference Polak, O’Leary and Kronmal20
In our study of a collective of symptomatic patients, 42% had intraplaque T1 hyperintensity on high-resolution black-blood VISTA MR, suggestive of IPH. The prevalence of hemorrhagic plaques in the general asymptomatic population has been estimated at 11.5%. Reference Van Lammeren, Den Hartog and Pasterkamp35 Considering that a higher prevalence of hemorrhage is usually found in symptomatic plaques in cases of more severe stenosis, Reference Kwee, van Oostenbrugge and Prins4 the 42% prevalence value in our study is considerable. It lies between the 31% prevalence of IPH found in symptomatic patients with mild stenosis and the 46% prevalence in patients with moderate stenosis of 50%–69%, in the Kwee et al. Reference Kwee, van Oostenbrugge and Prins4 study. The Schindler et al. meta-analysis from 2020 found a 51.6% prevalence of IPH in patients with symptomatic carotid stenosis and a 29.4% prevalence in patients with asymptomatic carotid stenosis. Reference Schindler, Schinner and Altaf14 A 37% prevalence of ipsilateral IPH has been described in patients with symptomatic low-grade stenosis in the Bayer–Karpinska study started in 2013. Reference Bayer-Karpinska, Schwarz, Wollenweber, Poppert and Saam12 These patients suffered from a stroke which was initially classified as cryptogenic. Also of note is that, in this latter study, only 3% of contralateral non-symptomatic plaques were hemorrhagic. The group of Altaf et al. Reference Altaf, Daniels and Morgan7 found 61% of IPH in symptomatic patients with 30%–69% stenosis. In the 2013 study by Hosseini et al., Reference Hosseini, Kandiyil, Macsweeney, Altaf and Auer2 which included patients with intermediate and high-grade stenosis, 64% of carotid plaques were hemorrhagic. Other studies reported IPH prevalence of 28% and 37% in 50%–79% and 50%–70% (moderate) stenoses groups, respectively. Reference Hosseini, Kandiyil, Macsweeney, Altaf and Auer2 This is in agreement with our results, which showed a positive relationship between NASCET degree of stenosis and presence of IPH.
If only 36 patients with DWI-positive infarcts in our study are considered, as many as 42% of them had a VISTA (+) plaque ipsilateral to the infarct. The mean stenosis degree of these plaques was only 50%. Our findings are in keeping with previous studies such as that conducted by Yoshida et al. Reference Yoshida, Sadamasa, Narumi, Chin, Yamagata and Miyamoto36 which found an association between symptomatic low-grade carotid stenosis with a vulnerable plaque and a high rate of stroke recurrence that was refractory to aggressive medical treatment. In those patients, CEA was a safe and effective alternative. Our study’s findings feed the discussion concerning a paradigm change in treatment recommendations for carotid plaques that goes beyond the mere degree of stenosis. A patient with a stable plaque with <70% stenosis might benefit from medical statin therapy alone whereas a hemorrhagic plaque should be considered at high risk for future events and might necessitate more aggressive, or even invasive, treatment, even if the degree of stenosis is lower than 50%.
The role of plaque imaging in the surveillance of medical therapy, particularly the influence of statins on plaque composition, needs to be elucidated in further studies. Reference Zavodni, Wasserman and McClelland27,Reference Liu, Zhao, Cao, Lu and Xu37 Plaque imaging should be evaluated in larger controlled clinical trials, including patients with mild stenosis carotid plaques, to reassess and possibly modify currently applied algorithms for invasive treatment. It will need to be clarified to which extent the thickness and percent stenosis degree of a hemorrhagic plaque influence its propensity to embolize, and further attention will be drawn on morphological aspects like IPH volume and intensity. Reference Yang, Liu and Han38 It also seems necessary to determine to which degree a hemorrhagic plaque develops inflammatory changes, e.g. through iron deposition, and following endothelial dysfunction. Reference Treiman, McNally, Kim and Parker5
A limitation of this study lies in the absence of histological correlation, not allowing exclusion of the hypothesis that some of the focal T1 hyperintensities observed do not represent plaque hemorrhage but rather fat or calcium deposits. It is also limited by the fact that only carotid bifurcation atheromatosis has been taken into account and not aortic arch disease.
In summary, 3D TSE T1 fat-saturated black-blood MRI provides information on plaque composition that goes beyond mere luminal stenosis measurement, showing other potentially independent risk factors and particularly probable IPH as a marker of vulnerability. Our study demonstrates that a high percentage (42%) of ischemic events in patients with a mean low- to moderate-grade of stenosis (50%) are associated with supposedly hemorrhagic plaques, an easily detectable MRI imaging marker. Plaque characterization imaging should be performed in situations of uncertainty concerning the etiology of an ischemic stroke and allows for more accurate risk stratification and management of patients presenting carotid plaques with <70% stenosis. It also noninvasively identifies high-risk patients who could benefit from surgical treatment.
Disclosures
None of the authors has any conflict of interest to report.
Statement of Authorship
MS performed the main writing of the article, with contributions and critiques from JB, IB, AP, RS, and KOL. JB performed the statistical analyses and provided tables and graphs. MIV conceived the study, oversaw the work, and assisted in writing and editing. AP prepared the images. BMD and JR provided technical support and feedback.
Funding
No grant or other funding has supported this study.