Introduction
Clay minerals are a class of inorganic material and are used widely in traditional medicine (Reference BourgeoisBourgeois, 2006; Reference CarreteroCarretero, 2002; Reference Carretero, Gomes, Tateo, Bergaya, Theng and LagalyCarretero et al., 2006; Reference Iborra and GonzálezIborra & González, 2006) with several industrial applications (Reference Anirudhan and RamachandranAnirudhan & Ramachandran, 2007; Reference Bergaya, Theng, Lagaly, Bergaya, Theng and LagalyBergaya et al., 2006; Reference Baltar, Luz, Baltar, Oliveira and BezerraBaltar et al., 2009; Reference Liu, Huang and WangLiu et al., 2008; Reference Madyan, Fan and HuangMadyan et al., 2017; Reference Xavier, Santos, Osajima, Luz, Fonseca and Silva FilhoXavier et al., 2016;). Among their physicochemical characteristics are significant surface reactivity, ion exchange, adsorption, colloidal and dispersion capabilities, rheological properties, and low toxicity. Such features make them suitable for use as biomaterials, including excipients or active ingredients in pharmaceutical formulations, cosmetics (Reference CarreteroCarretero, 2002), drug carriers (Reference Kim, Choi, Elzatahry, Vinu, Choy and ChoyKim et al., 2016; Reference López-Galindo, Viseras and CerezoLópez-Galindo et al., 2007), regenerative medicine (Reference Chrzanowski, Kim and NeelChrzanowski et al., 2013; Reference Ghadiri, Chrzanowski and RohanizadehGhadiri et al., 2015), and development of advanced materials, such as nanocomposites (Reference Alcântara, Darder, Aranda, Ayral and Ruiz-HitzkyAlcântara et al., 2015; Reference Ruiz-Hitzky, Van Meerbeek, Bergaya, Theng and LagalyRuiz-Hitzky & Van Meerbeek 2006; Reference Ruiz-Hitzky, Darder, Fernandes, Wicklein, Alcântara and ArandaRuiz-Hitzky et al., 2013).
Palygorskite (Plg) is a raw 2:1 phyllosilicate with the approximate structural formula Si8O20(Al2Mg2)(OH)2(OH2)4.4H2O (Reference García-Romero, Barrios and RevueltaGarcía-Romero et al., 2004) belonging to a nanofibrous group of clay minerals. The fiber-forming nature, resulting from the 180° inversion that occurs at every four tetrahedra of silicon, leads to the formation of a structure in open channels parallel to its central octahedral axis. This conformation is responsible for its adsorptive properties and large specific surface area, making it a suitable candidate to host bioactive molecules (Reference Carazo, Borrego-Sánchez, García-Villén, Sánchez-Espejo, Viseras, Cerezo and AguzziCarazo et al., 2018; Lopéz-Galindo et al., 2007; Reference Romero and BarriosRomero & Barrios, 2008; Reference Tajeu, Ymele, Jiokeng and TonleTajeu et al., 2018). One of the advantages of fibrous clay minerals, when compared to layered ones, is the silanol groups located on the outer surface and their negative charges which act as centers of interaction with polysaccharides such as chitosan, proteins, lipids, nucleic acids, enzymes, and amino acids (Reference Alcântara, Darder, Aranda and Ruiz-HitzkyAlcântara et al., 2012; Reference Alcântara, Darder, Aranda and Ruiz-HitzkyAlcântara et al., 2014, 2015An et al., 2015; Benteoli et al., 2007; Reference Castro-Smirnov, Ayache, Bertrand, Dardillac, Le Cam, Aranda and O., Pilar, A., Ruiz-Hitzky, E., & Lopez, B. S.Castro-Smirnov et al., 2017; Reference Huang, Liu and WangHuang et al., 2009; Reference Mbouguen, Ngameni and WalcariusMbouguen et al., 2006, Reference Mbouguen, Ngameni and Walcarius2007; Reference Ruiz-Hitzky, Darder, Fernandes, Wicklein, Alcântara and ArandaRuiz-Hitzky et al., 2013; Reference You, Yin, Gu, Xu and SangYou et al., 2011; Reference ZaiaZaia, 2004; ).
Numerous factors justify the use of Plg in devices. As Reference Chen and JinChen and Jin (2010) reported, Plg has a moderate absorptive capacity, good biological affinity, and a role in enhancing the accessibility of bioactive sites. Plg is a suitable immobilization matrix to prevent the protein structures (e.g. enzymes) from unfolding, resulting in high detective sensitivity during electrochemical sensing (Reference Wu, Cai, Chen, Zhang and CaiWu et al., 2011). However, despite the potential of Plg associated with biomolecules in the design of nanostructured systems, no study involving antimicrobial peptides (AMPs) has been reported.
AMPs correspond to a class of biomolecules isolated from various living organisms as part of the innate immune system. Their potential as new antibiotic molecules is firmly established in the literature as drug candidates (Reference Chu, Yip, Chen, Yu, Chih, Cheng, Chou and ChengChu et al., 2015; Reference Ntwasa and AbdelmohsenNtwasa, 2012). Research involving AMPs has demonstrated their high activity in the micromolar concentration with a broad spectrum against bacterial pathogens (Reference Mor, Hani and NicolasMor et al., 1994), fungi (Reference Leite, Brand, Silva, Kückelhaus, Bento, Araújo, Martins, Lazzari and BlochLeite et al., 2008), viruses (Reference Belaid, Aouni, Khelifa, Trabelsi, Jemmali and HaniBelaid et al., 2002), protozoa (Reference Brogden and BrogdenBrogden & Brogden, 2011; Reference Calderon, Silva-Jardim and ZulianiCalderon et al., 2009; Reference Moraes, Nascimento, Miura, Leite, Nakano and KawanoMoraes et al., 2011a, Reference de Moraes, Nascimento, Miura, Leite, Nakano and Kawano2011b), and cancer cells (Buri et al., 2011; Reference Chu, Yip, Chen, Yu, Chih, Cheng, Chou and ChengChu et al., 2015; Reference Gaspar, Veiga and CastanhoGaspar et al., 2013).
Dermaseptin 01 (DRS 01) (Reference Amiche, Ladram and NicolasAmiche et al., 2008) is a peptide isolated from the cutaneous secretion of South American amphibians of the genus Pithecopus (Cope, 1866). DRS 01 has been the subject of many studies since its discovery, including its association with nanostructures (Reference Bittencourt, de Oliveira Farias, Bezerra, Véras, Silva, Costa, Bemquerer, Silva, de Almeida, Leite and EirasBittencourt et al., 2016; Reference Brand, Leite, Silva, Albuquerque, Prates, Azevedo, Carregaro, Silva, Sá, Brandão and BlochBrand et al., 2002, Reference Brand, Leite, de Sá Mandel, Mesquita, Silva, Prates, Barbosa, Vinecky, Martins, Galasso, Kuckelhaus, Sampaio, Furtado, Andrade and Bloch2006, 2013; Reference Castiglione-Morelli, Cristinziano, Pepe and TemussiCastiglione-Morelli et al., 2005; Reference Eaton, Bittencourt, Costa Silva, Véras, Costa, Feio and LeiteEaton et al., 2014; Reference Leite, Brand, Silva, Kückelhaus, Bento, Araújo, Martins, Lazzari and BlochLeite et al., 2008; Reference Moraes, Nascimento, Miura, Leite, Nakano and KawanoMoraes et al., 2011a, Reference de Moraes, Nascimento, Miura, Leite, Nakano and Kawano2011b, 2013; Reference Salay, Nobre, Colhone, Zaniquelli, Ciancaglini, Stabeli, Leite and ZucolottoSalay et al., 2011; Reference Silva, Leite, Brand, Regis, Tedesco, Azevedo, Freitas and BlochSilva et al., 2008; Reference Zampa, Araújo, Costa, Nery Costa, Santos, Zucolotto, Eiras and LeiteZampa et al., 2009). Recent research has focused on strategies for the modification of AMPs, including hybrid, stabilized, or immobilized AMPs using various materials, allowing innovations in the applications due to increased stability or even the enhancement of previously existing properties (Reference Brogden and BrogdenBrogden & Brogden, 2011; Reference Costa, Carvalho, Montelaro, Gomes and MartinsCosta et al., 2011; Reference Onaizi and LeongOnaizi & Leong, 2011). Because of their biological activity, AMPs can be used as recognition elements when interacting with target substances through intermolecular reactions in sensor devices or as coating agents Reference Lillehoj, Kaplan, He, Shi and Ho(Lillehoj et al., 2014; Reference Plácido, de Oliveira Farias, Marani, Vasconcelos, Mafud, Mascarenhas, Eiras, Leite and Delerue-MatosPlácido et al., 2016; Reference da Silva, Fortes, Oliveira, Freitas, Soares, Sobrino and Leiteda Silva et al., 2014).
Electrochemical sensors are among the most widely used chemical sensors due to their selectivity, sensitivity, simplicity, and low cost. In this field, organic molecules immobilized on clay minerals may play an essential role in developing electrochemical sensors and biosensors as matrices for the adsorption of electroactive ions (Reference Gianfreda, Rao, Saccomandi and ViolanteGianfreda et al., 2002; Reference MoustyMousty, 2004; Reference Navrátilová and KulaNavrátilová & Kula, 2003). Aluminosilicates are materials of interest because of their hydrophilicity, porosity, and mechanical and thermal stability (Reference Chen and JinChen & Jin, 2010; Reference MoustyMousty, 2004; Reference Navrátilová and KulaNavrátilová & Kula, 2003).
The production of functional films based on clay minerals is possible from nanometric dispersions in aqueous media (Reference Chrzanowski, Kim and NeelChrzanowski et al., 2013; Reference Ghadiri, Chrzanowski and RohanizadehGhadiri et al., 2015; Reference Huang, Liu and WangHuang et al., 2008; Reference Ruiz-Hitzky, Darder, Fernandes, Wicklein, Alcântara and ArandaRuiz-Hitzky et al., 2013). Thus, clay minerals represent suitable materials for preparing nanostructured composites associated with AMPs in the search for new materials for use in biotechnology. Thus, the layer-by-layer (LbL) self-assembly technique enables the formation of thin films where the properties of Plg and DRS 01, for example, can be combined and explored at a molecular level. These films can be used in several areas, e.g. as electrochemical sensors, as biomolecule-delivery systems, and as functional coatings (Reference DecherDecher, 1997; Reference Decher and SchlenoffDecher & Schlenoff, 2012; Gentile et al., 2015; Kim et al., 2015; Reference LehnLehn, 2007; Picart et al., 1998, 2015; Reference Rawtani and AgrawalRawtani & Agrawal, 2014; Reference Ruiz-Hitzky, Darder, Fernandes, Wicklein, Alcântara and ArandaRuiz-Hitzky et al., 2013; Reference Skorb, Volkova and AndreevaSkorb et al., 2014).
Thus, the objective of the present study was to prepare nanostructured films where the properties of Plg are combined at the molecular level with DRS 01, serving as a support platform for immobilizing this peptide and improving its adoption and availability. The strategy proposed in this study led to the development of a new nanomaterial the applications of which can be explored in the vast biotechnology field.
Experimental
Preparation of Materials
Antimicrobial Peptide
DRS 01 (GLWSTIKQKGKEAAIAAA-KAAGQAALGAL-NH2) was synthesized by the 9-fluorenylmethyloxycarbonyl (Fmoc) solid-phase strategy (Reference Chan and WhiteChan & White, 2000) as reported previously (Reference BrogdenBrogden, 2005). Reagents used for peptide synthesis were acquired from Peptides International (Louisville, Kentucky, USA). Purification was performed on a preparative reversed-phase column (RP) (Vydac 218 TP 510, Hesperia, California, USA) coupled to the HPLC system model LC-10VP (Shimadzu Corp., Kyoto City, Japan). The molecular weight (2793.39 Da) and sample purity were confirmed using MALDI-TOF/TOF mass spectrometry, as described by Reference Bittencourt, de Oliveira Farias, Bezerra, Véras, Silva, Costa, Bemquerer, Silva, de Almeida, Leite and EirasBittencourt et al. (2016).
Palygorskite
Palygorskite (Plg) was collected from a deposit located in the Coimbra, Cardoso company mine (coordinates: 23 M064043 9,249,510; elevation: 185.7 m) in the municipality of Guadalupe, state of Piauí (northeast region of Brazil). The raw Plg was submitted to the beneficiation process, involving comminution and magnetic separation steps in a high-intensity field (14,000 Gauss) to remove iron (oxyhydr)oxides. The next stage was wet sieving in a 635# sieve (20 μm). The <20 μm fraction was purified by washing several times in distilled water and centrifuging. The precipitate was dried and treated with hydrogen peroxide (H2O2) in sodium acetate and acetic acid buffer under stirring at 45°C for 72 h; all the chemicals were obtained from Sigma Aldrich (St. Louis, Missouri, USA). Following further washings, the fine fraction was stored in a desiccator and used to produce LbL films. All the chemicals were of analytical quality and were used as supplied without further purification.
Preparation and Characterization of LbL Films
The ITO slides (A ≈ 0.40 cm2) were previously cleaned in detergent and deionized water (1:6, v:v) under heating until 75°C and, after that, sonicated in acetone (purchased from Merck, São Paulo, Brazil) for 10 min. Subsequently, the RCA (Radio Corporation of America) protocol was used to hydrophilize the substrates in an alkaline medium (Reference Kim, Granstrom, Amigo, Johansson, Salaneck, Daik, Festa and CacialliKim et al., 1998) by adding deionized water, NH4OH, and H2O2 (purchased from Merck) (5:1:1, v:v). The substrates were dipped in the RCA solution at 75–80°C for 30 min and then rinsed twice in deionized water. All substrates were then dried with a gentle N2 flow. For the deposition of films, DRS 01 and Plg were first stored individually in 1.5 mL microtubes and dispersed in deionized water (pH 5.6, 25°C, resistivity of 18 MΩ cm). Both dispersions were used at the concentration of 1.0 mg mL-1 and sonicated for 10 min to improve the dispersion of the material (Reference Fernandes, Ruiz, Darder, Aranda and Ruiz-HitzkyFernandes et al., 2009). In the case of Plg, this step was fundamental for obtaining nanoscale particles and improving their adsorption capacity (Reference Wang and WangWang & Wang, 2016). The immobilization was performed by alternating exposure of the ITO substrate to Plg and DRS 01, respectively, for 5 min, according to previous studies (Reference Bittencourt, de Oliveira Farias, Bezerra, Véras, Silva, Costa, Bemquerer, Silva, de Almeida, Leite and EirasBittencourt et al., 2016; Reference Zampa, Araújo, Costa, Nery Costa, Santos, Zucolotto, Eiras and LeiteZampa et al., 2009). Between immersions, the substrates were washed in deionized water to remove non-adsorbed molecules and dried with a gentle N2 flow.
Palygorskite Characterization
XRD
X-ray diffraction of Plg was carried out using the powder method and the results collected using a Bruker D-4 Endeavor instrument (Billerica, Massachusetts, USA) with CuKα (40 kV/40 mA). The scans were conducted from 4 to 80º2θ using a step size of 0.04º2θ and a counting time of 2 s, using a Lynx Eye position-sensitive detector. Qualitative interpretations of the XRD patterns were compared using the standards in the database PDF02 (ICDD, 2006) in the Bruker software, Diffrac Plus .
FTIR
Infrared spectra were obtained using KBr pellets in the region between 4000 and 400 cm–1 with a Bruker Vector 3.3 FTIR spectrometer. A total of 96 scans was collected for each sample at 4 cm–1 resolution.
XRF
The chemical composition of the Plg sample was determined using an Axios X-ray fluorescence spectrometer (Panalytical). The purified Plg sample was prepared in a Vaneox automatic press (20 mm template, P = 20 ton and t = 30 s), using boric acid (H3BO3) as the binder in the ratio of 1:0.3–0.6 g of acid and 2.0 g of the dried sample at 100°C. Semi-quantitative results (standardless) were expressed as %, calculated as oxides normalized to 100%.
SEM-EDS
Scanning electron microscopy and energy dispersive X-ray spectroscopy were performed using an FEI-Quanta FEG 250 SEM instrument (Thermo Fischer Scientific, Hillsboro, Oregon, USA). The samples were sputter coated with Au and imaged using both the secondary electron and backscattered electron modes.
Transmission Electron Microscopy (TEM)
The micrographs were obtained using a Philips CM 200 Super Twin Transmission Electron Microscope. The samples were prepared by dispersing the material in 2-propanol in microtubes submitted to an ultrasonic bath for 10 min. After fiber dispersion, a drop was deposited on a microscopy grid and dried before examination.
Film Characterizations
Cyclic Voltammetry (CV)
All measurements were performed in triplicate to ensure their reproducibility. The Plg/DRS 01 LbL films were characterized by CV in a Dropsens μStat 400 bipotentiostat/galvanostat instrument (Dropsens, Llanera, Spain) and an electrochemical cell with a capacity of 50 mL containing three electrodes. The reference electrode was a saturated calomel electrode (SCE) [Hg/HgCl/KCl (sat.)], while a platinum plate (A = 1.0 cm2) was used as an auxiliary electrode. The LbL films deposited on the ITO electrode for ITO/Plg/DRS 01 sequence were used as the working electrodes. Potassium phosphate buffer (KH2PO4/K2HPO4), 0.1 M, pH 7.2 at 25°C, was used as an electrolytic solution. The potential scanning rate was 50 mV s–1.
Spectroscopy in the Ultraviolet-Visible Region (UV-Vis)
LbL films were characterized by UV-Vis spectroscopy employing an Agilent Cary 60 spectrophotometer (Santa Clara, California, USA) between 200 and 800 nm scanning. For the UV-Vis measurements, the Plg/DRS 01 film was adsorbed on a quartz substrate to probe peptide absorption in the ultraviolet region (216 and 280 nm). Successive spectra were recorded for each adsorbed bilayer until the stable growth of the LbL film was observed.
AFM
AFM was used to evaluate the surface roughness of the ITO/Plg and ITO/Plg/DRS 01 films and the ITO clean substrate. Images were collected at 512-pixel resolution in tapping mode on a TT-AFM instrument (AFM Workshop, Signal Hill, California, USA). Representative images were examined using ACT-20 (AppNano, Mountain View, California, USA) silicon tip cantilevers with radius <10 nm with a resonance frequency of ~353 kHz. Three areas of each sample were examined. The images were analyzed using Gwyddion 2.4 software. All roughness results were expressed as mean ± SD. To compare the average roughness (Ra values) of surfaces in the LBL films, an analysis of variance (ANOVA) was performed. Statistical analyses were performed using GraphPad Prism® 6.0 software, and a p < 0.05 value was considered statistically significant.
Results and Discussion
XRD Analysis of Plg
The X-ray patterns of Plg in the non-magnetic <20 μm fraction and purified Plg were obtained after the chemical treatment (Fig. 1). The results indicated that the sample consisted mainly of palygorskite and, secondarily, kaolinite and quartz were identified with the help of crystallographic data sheets for both samples (JCPDF No. 31-0783, 1-830971, and 1-791910).
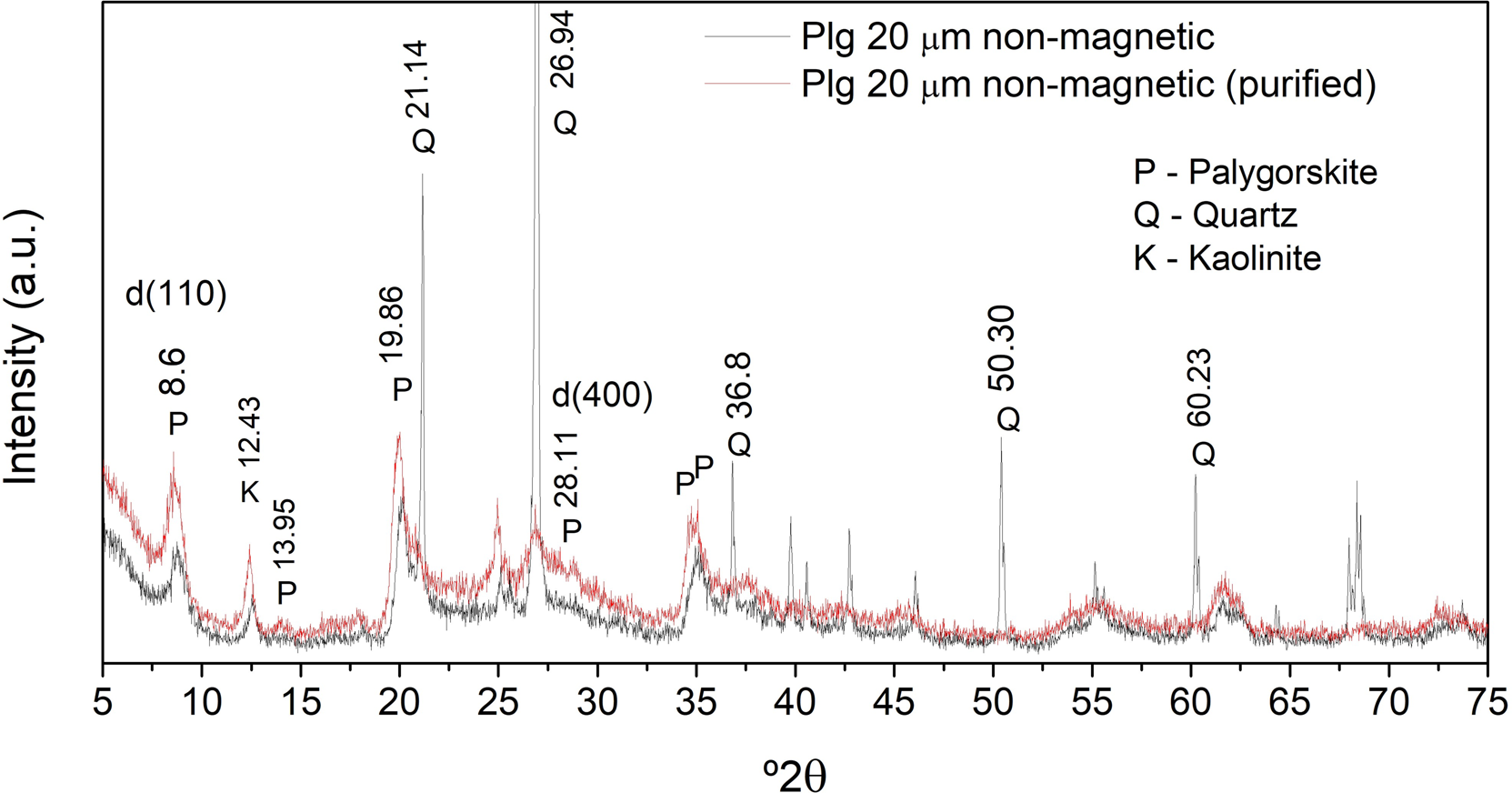
Fig. 1 XRD patterns of non-magnetic <20 μm and purified non-magnetic <20 μm clay samples. CuKα (40 kV/40 mA). The letters above each peak are assignments of the origin (see legend)
The XRD region of Plg with the highest reflection intensity was at ~8.6°2θ (Reference Sheng and WangSheng & Wang, 2005), which corresponded to d 110 = 1.05 nm attributed to the basal plane in the Plg structure (Reference Oliveira, Coelho, Gomes, Costa, Fonseca, Sousa, Espínola and FilhoBailey, 1980; Oliveira et al., 2013; Reference da Silva, Fortes, Tomé, da Silva Filho, Freitas, Soares-Sobrinho, Leite and Soaresda Silva et al., 2013).
Other reflections characteristic of this clay material were also identified at 13.95, 19.86, and 28.11°2θ for the (200), (040), and (400) reflections, respectively. The diffraction next to 27.00°2θ is associated with the presence of quartz.
X-ray diffraction of the purified sample revealed an increase in the characteristic intensity of Plg attributed to the (110) reflection, which is of greater intensity than the quartz reflection. This evidence suggests a high purity obtained by complementing the 635# mesh screening techniques with washing and centrifugation of the raw Plg.
FTIR Results of Plg
The bands in the FTIR spectra (Fig. 2) were divided into the stretching vibrations of structural O-H and adsorbed water and the O–H bending vibrations in the microchannels or “zeolitic” water (Reference MurrayMurray, 2007; Reference Suárez and García-RomeroSuárez & García-Romero, 2006a).
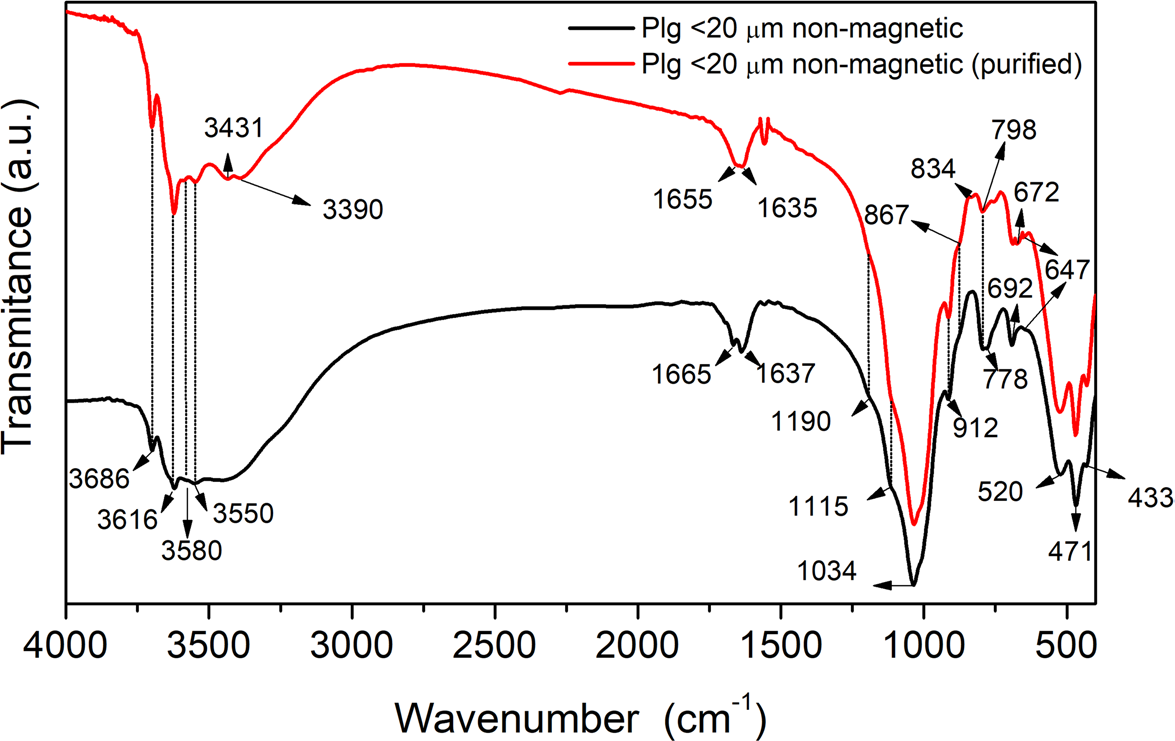
Fig. 2 FTIR spectra in the 4000-400 cm-1 region of non-magnetic <20 μm and purified non-magnetic samples
The band at 3686 cm-1 (Fig. 2) is attributed to the stretching vibrations of octahedral Mg3–OH groups (Reference Cai, Xue and PolyaCai et al., 2007; Reference Yan, Liu, Tan, Yuan and ChenYan et al., 2012). The 3616 cm–1 and 912 cm–1 bands are attributed to the octahedral Al2–OH stretching and deformation modes, respectively (Reference Suárez and García-RomeroSuárez & García-Romero, 2006a). Although the band positions in the two Plg samples were similar, the greater intensity of the band at 3616 cm–1 in the purified sample confirmed the XRD results.
A low-intensity band at 3580 cm–1 was attributed to Al–OH–Fe3+ stretching (Reference Suárez and García-RomeroSuárez & García-Romero, 2006a) and the band at 3550 cm–1 can be attributed to the octahedral OH–Fe+3 stretching (Reference Yan, Liu, Tan, Yuan and ChenYan et al., 2012). By the results of Reference Suárez and García-RomeroSuárez & García-Romero (2006b), the band at 3431 cm–1 appeared more clearly after the purification of the Plg, which can be attributed to the stretching of hydroxyls in the adsorbed water molecules. The band at 3390 cm–1 originates from intramolecular hydrogen bonding of OH with Al–Fe3+ or Al–Mg groups in the Plg structure (Reference Xavier, Santos, Osajima, Luz, Fonseca and Silva FilhoXavier et al., 2016; Reference Suárez and García-RomeroSuárez & García-Romero 2006a, Reference Suárez and García-Romero2006b). According to Madejová and Komadel (2001), the bands at 1006 and 1028 cm–1 can be attributed to the Si–O vibrational stretching in the plane and 1114 cm–1 in the Si–O stretching vibrations in the plane (longitudinal mode).
The band at 834 cm-1 appeared only in the purified sample. According to Reference Suárez and García-RomeroSuárez and García-Romero (2006a), it belongs to Al–Mg–OH bending, indicating that Mg2+, for this sample, may occupy the M2 sites in the octahedral sheet. However, as observed by Reference Chahi, Petit and DecarreauChahi et al. (2002), the low intensity of this band casts doubt on this suggestion.
The band at 647 cm-1, according to Reference Yan, Liu, Tan, Yuan and ChenYan et al. (2012), consists of a characteristic vibration of the Plg related to its channel structure. As stated by Reference Cai, Xue and PolyaCai et al. (2007), in samples poor in Mg2+, as is the case for the Plg sample in this study, this band is attributed to Fe3+–OH groups.
Impurities in the samples may account for the FTIR bands at 798 and 778 cm-1, typical of symmetrical quartz stretching vibrations (Si-O-Si), as reported by Reference Suárez and García-RomeroSuárez and García-Romero (2006a) and Reference Yan, Liu, Tan, Yuan and ChenYan et al. (2012). The first band showed a visible reduction in the purified sample, while the latter disappeared, corroborating the XRD results.
XRF Analysis of Plg
The chemical composition (wt.%) of the purified Plg was obtained by XRF analysis (Table 1), which identified the main oxides present in the sample. The results obtained by XRF indicated that SiO2 and Al2O3 are the main constituents of the sample and that the Al content is greater than Si. This result also indicated that the Plg is of type I, according to the classification proposed by Reference Suárez, García-Romero, Del Río, Martinetto and DooryhéeSuárez et al. (2007), giving it a dioctahedral character in which the octahedral sheet is occupied primarily by Al, with lesser Mg (20.4% Al2O3 and 3.9% MgO). These results agreed with those of Reference Belaroui, Youcef and BengueddachBelaroui et al. (2014).
Table 1 Chemical composition of purified Plg sample (wt.%)

* Loss on ignition
As Reference Suárez and García-RomeroSuárez and García-Romero (2006a) stated, the use of the chemical composition data obtained by XRF to determine the di- or trioctahedral character of the Plg can be checked by comparing with the FTIR spectra. The position and intensity of the band at 3616 cm-1 is more consistent with Al2-OH than with Mg3-OH and, thus, indicates that the Plg is primarily dioctahedral.
Because the sample was subjected to magnetic separation, the remaining amount of iron consisted mostly of structural octahedral Fe3+. Reference Stucki, Bergaya, Theng and LagalyStucki (2006) stated that removing separate iron oxide phases by chemical means without some alteration in the remaining phases is unlikely. An advantage of the magnetic separation technique is that it does not alter the constituents in the clay mineral structure, as occurs with chemical processes.
Morphology Analysis of Plg by SEM and TEM
The morphology and porosity of the purified Plg were evaluated by SEM (Fig. 3A,B) and TEM (Fig. 3C,D) measurements. SEM images displayed aggregates of rods, usually formed by lath units scarcely visible at a magnification of <50,000×. According to the SEM images, several aggregated rods can form bundles of fibers, which has also been observed by others (García-Romero et al., 2013).
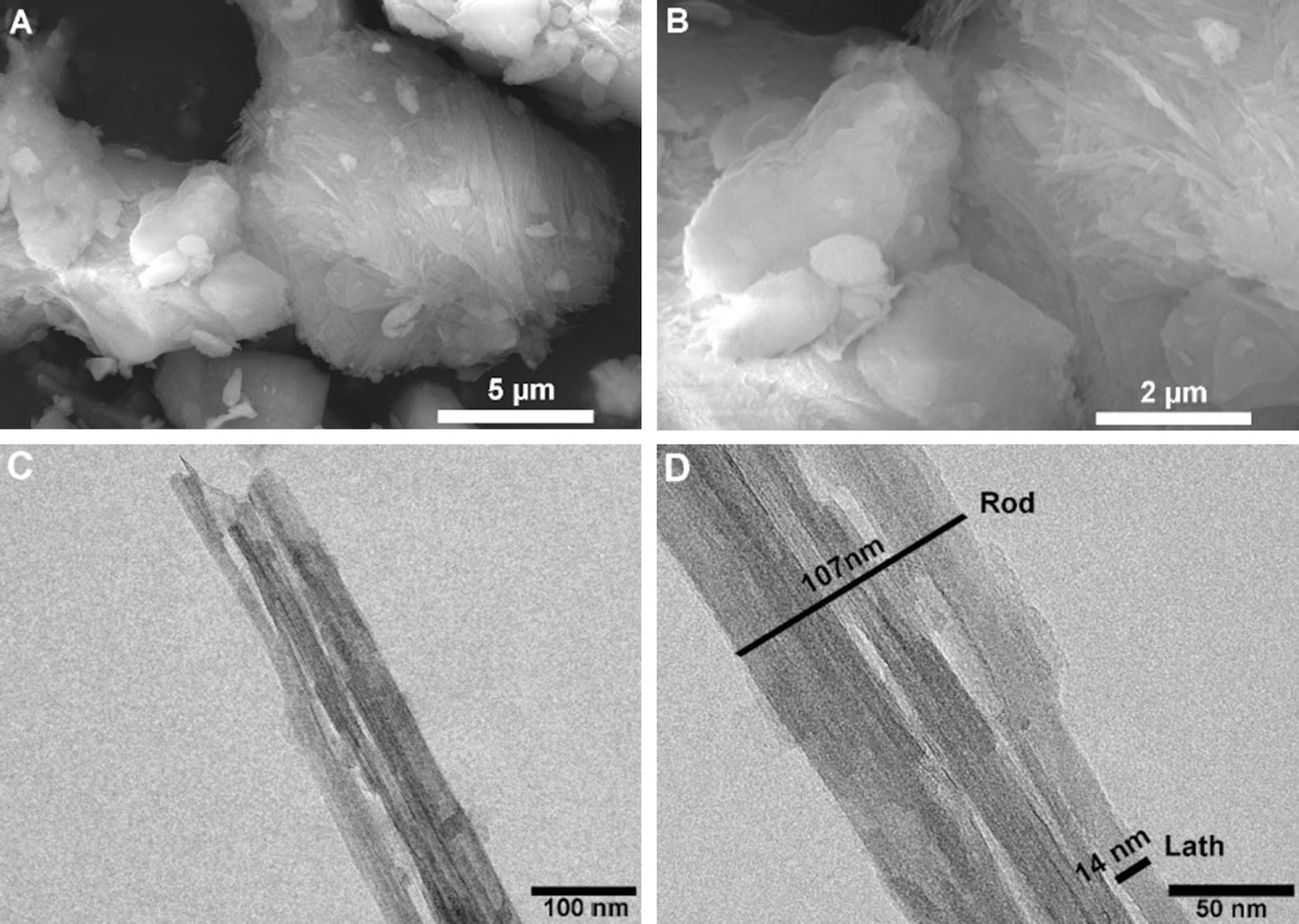
Fig. 3 SEM (A, B) and TEM (C, D) images of purified Plg
The TEM images (Fig. 3C,D) corroborated the SEM results, showing individual laths building up rods and, consequently, a group of rods. The laths in the rods showed a width <20 nm, which also agreed with previous works (Reference Gan, Zhou, Wang, Du and ChenGan et al., 2009; García-Romero et al., 2013; Reference Xavier, Santos, Osajima, Luz, Fonseca and Silva FilhoXavier et al., 2016). SEM and TEM analysis revealed lath lengths from 0.5 to 5 μm, confirming the formation of small- and medium-sized fibers (García-Romero et al., 2013). SEM and TEM images also displayed straight and rigid fibers without bending variation.
The texture composed of straight fibers oriented in all directions suggested high porosity and, generally, associated with an increase in surface area available for adsorbence modification and related applications (Suarez et al., 2013; García-Romero et al., 2013; Wang et al., 2016).
It is possible to verify that the bundle of fibers was formed by smaller fibers that can vary between 10 and 30 nm, corroborating with the studies by Reference García-Romero and SuárezGarcía-Romero and Suárez (2013). That corresponds to the crystal unit. When dispersed in solution and subjected to a sonication process, these fibers form a nanoscale network with advantageous properties such as large area and surface activity (Reference Wang and WangWang & Wang, 2016).
Electrochemical Studies of LbL Films Based on Plg and DRS 01
Cyclic voltammetry was used to verify the electrochemical behavior of the peptide associated with the clay mineral after deposition in the LbL film (Fig. 4). The CV was initially recorded for the unmodified ITO substrate. ITO was modified with a monolayer of DRS 01 or purified Plg conjugated to the antimicrobial peptide DRS 01 in bilayer film form (ITO/Plg/DRS 01). Aiming to verify the influence of the purification process on Plg, a film containing unpurified Plg (IN) (ITO/Plg-IN/DRS) was also obtained (Fig. 4). The unmodified ITO exhibited no redox reaction over the potential range from +0.5 to +1.0 V (vs SCE and v = 50 mV/s). However, the ITO modified with a DRS 01 monolayer film was oxidized at +0.77 V vs SCE, which is attributed to the electroactivity of the peptide due to the presence of tryptophan residues (Trp) (Plgeček, 2015).
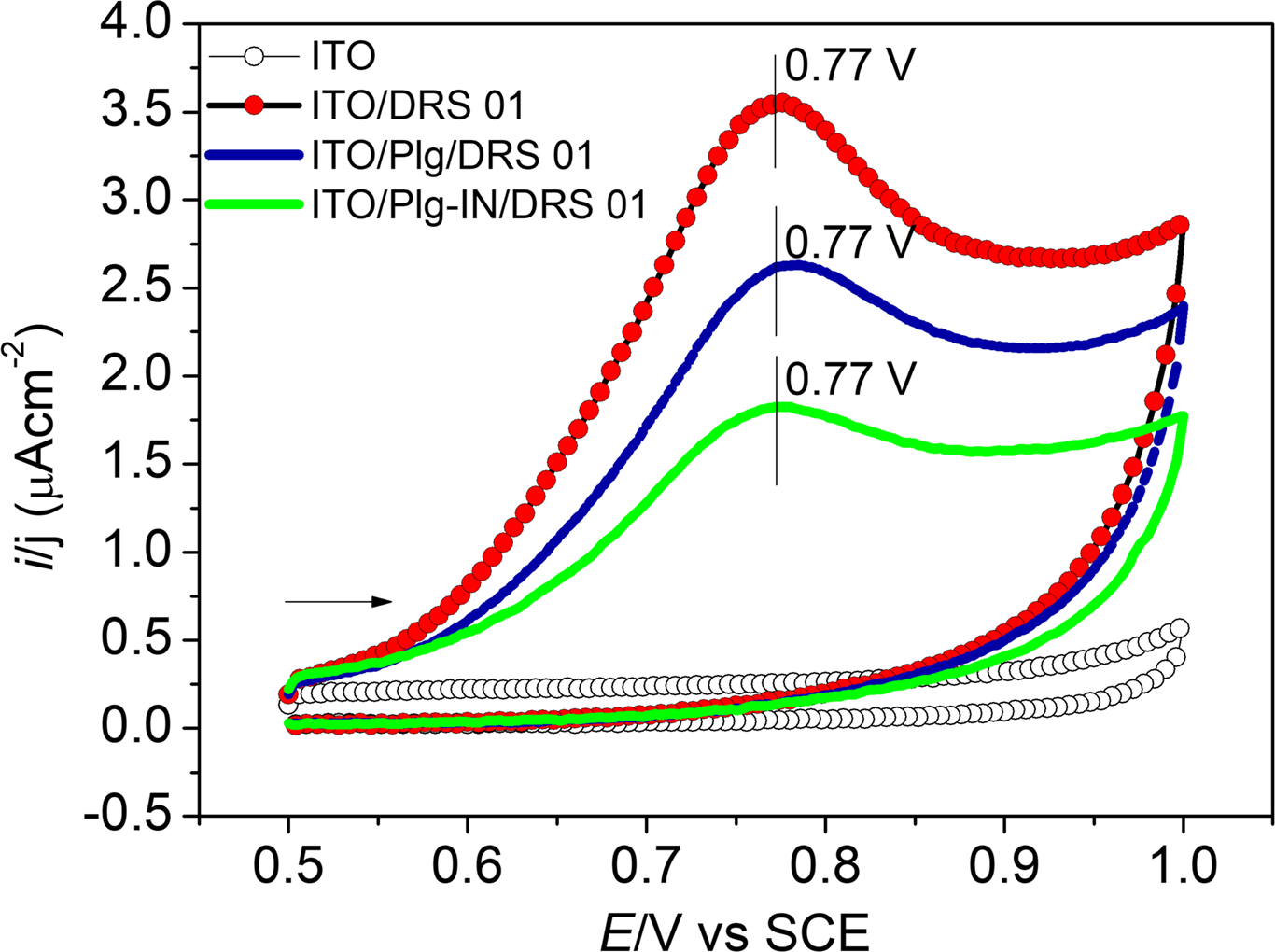
Fig. 4 Cyclic voltammograms of the unmodified ITO electrode (open circles), and ITO modified with DRS 01 monolayer film (ITO/DRS 01 - red), one bilayer of ITO/Plg/DRS 01 (blue), or ITO/Plg-IN/DRS 01 (green). All measurements were performed in 0.1 mol L–1 phosphate buffer, pH 7.5, at 50 mV s–1
The presence of the clay mineral in the ITO modified with bilayer films, i.e. ITO/Plg/DRS 01 or ITO/Plg-IN/DRS 01, notably neither blocked the electrode surface or interfered with the peptide response, which continued to be observed at the same potential (Fig. 4).
According to Reference MurrayMurray (2007), Plg maintains its properties in the presence of electrolytes, without flocculation, because of the inhibition of sedimentation by the elongated crystals. In addition, when dispersed in water, fibrous clay minerals can interconnect their fibers, creating an anchorage network for biomolecules. Studies showed that raw clay minerals are suitable for incorporation or immobilization of biological molecules (Reference Huang, Liu and WangHuang et al., 2008, Reference Huang, Liu and Wang2009; Reference Zhao, Wang, Li, Chen and LiuZhao et al., 2011). The CV results suggest that DRS 01 and Plg maintained their integrity in the film system studied here.
Comparing the bilayer films (Fig 4.) revealed an increase in current density from 1.82 μA cm-2 for the film containing unpurified Plg (ITO/Plg-IN/DRS 01) to 2.63 μA m–2 in the film containing purified Plg (ITO/Plg/DRS 01). The results confirmed that removing impurities from Plg influences the electrochemical response of the bilayer film and, consequently, its application. In addition, these results corroborate those indicated by the XRD analysis. Thus, because of the improvement in the current signal, the film based on purified Plg was selected for subsequent studies.
As stated by Reference An, Zhou, Zhuang, Tong and YuAn et al. (2015), the large surface area of clay minerals exposes functional groups such as Si–O–Si, Si–OH, and Al(Mg)–OH, as well as hydrophobic groups in the tetrahedral sheet (hydroxyl groups) that can contribute to the interaction with organic molecules. For example, the hydrophilic portion of the octahedral surface interacts with the positively charged side chains of the proteins (Yu et al., 2013). Hydroxyl groups within the boundaries of tetrahedral and octahedral sheets are suitable for forming hydrogen bonds with enzymes, for example. In addition, van der Waal’s forces play an essential role in binding proteins onto clay minerals and afford a partial overlap of the electrostatic repulsion (Yu et al., 2013). Therefore, other non-covalent interactions can occur between the Plg and AMPs and may govern the formation of the LbL films.
Previous studies into the anchoring of biomolecules on Plg (Reference An, Zhou, Zhuang, Tong and YuAn et al., 2015; Reference Basiuk, Gromovoy and Khil'chevskayaBasiuk et al., 1995; Benteoli et al., 2007) suggested that the conjugation with DRS 01 mainly occurs through electrostatic interactions, including hydrogen bonds, between the negatively charged clay surface (Reference An, Zhou, Zhuang, Tong and YuAn et al., 2015) and the positively charged α-helical peptide structure (Reference Brand, Leite, de Sá Mandel, Mesquita, Silva, Prates, Barbosa, Vinecky, Martins, Galasso, Kuckelhaus, Sampaio, Furtado, Andrade and BlochBrand et al., 2006; Reference Castiglione-Morelli, Cristinziano, Pepe and TemussiCastiglione-Morelli et al., 2005). Furthermore, studies that investigated the adsorption of peptides and amino acids on the surfaces of silicates revealed a significant difference between the isoelectric point, e.g. for lysine (Lys) (pI = 9.74) and the point of zero charge for SiO2 (pzc = 2.8) (Reference ZaiaZaia, 2004; Reference Parbhakar, Cuadros, Sephton, Dubbin, Coles and WeissParbhakar et al., 2007). Further studies are necessary to determine the secondary structure of DRS 01 (pI =10.0) (Reference Leite, Brand, Silva, Kückelhaus, Bento, Araújo, Martins, Lazzari and BlochLeite et al., 2008) bound to Plg. Bioactivity is required to explore AMPs as detection agents, and the present study allowed a perspective of the application of these films in areas such as clinical medicine, food safety, infection prevention, and environmental protection.
The next step of this study was to evaluate the influence of the number of deposited bilayers (n) on the electrochemical response of ITO/Plg/DRS 01 films (Fig. 5).
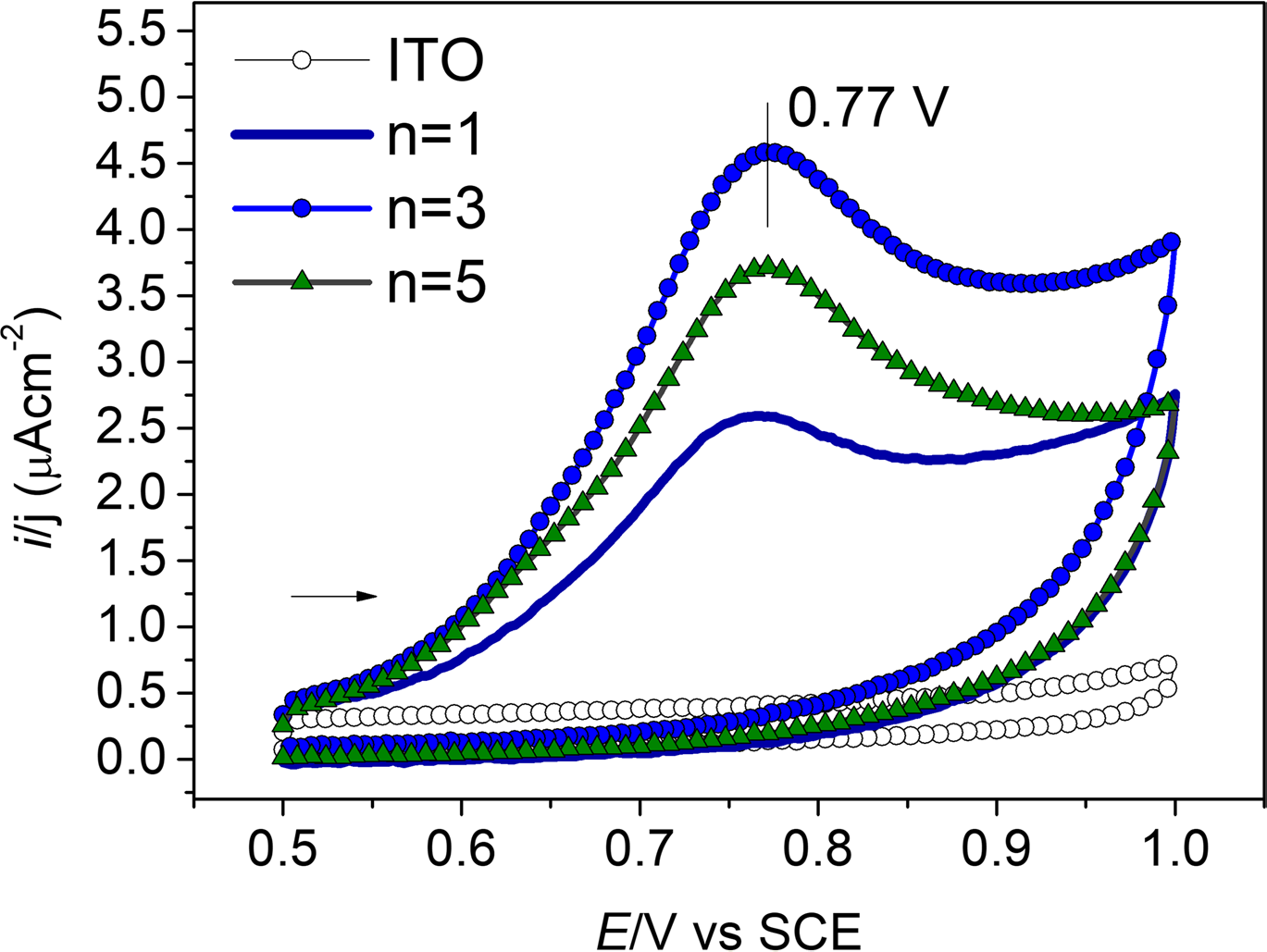
Fig. 5 Cyclic voltammograms for ITO/Plg/DRS 01 with different numbers (n) of bilayers adsorbed. All measurements were performed in phosphate buffer 0.1 mol L-1 phosphate buffer, pH 7.25, at 50 mV s–1
The three-bilayer film ITO/(Plg/DRS 01)3 showed an increase in current density to 4.60 μA cm–2 (Fig. 4) compared to 2.54 μA cm–2 in the film with a single bilayer (Fig 5). On the other hand, in the 5-bilayer film, the current density decreased to 3.71 μA cm–2.
Many adsorbed layers hinder the charge transfer to the electrode/electrolyte interface. Because of the results obtained, the ITO/(Plg/DRS 01)3 film appeared as a new material with potential applications in biotechnology. It was selected for infrared spectroscopy and atomic force microscopy characterization.
In other studies, DRS 01 peptide was immobilized onto cashew gum, a natural polymer (Reference Bittencourt, de Oliveira Farias, Bezerra, Véras, Silva, Costa, Bemquerer, Silva, de Almeida, Leite and EirasBittencourt et al., 2016), or on synthetic materials such as tetrasulfonated phthalocyanines (Reference Zampa, Araújo, Costa, Nery Costa, Santos, Zucolotto, Eiras and LeiteZampa et al., 2009). Both films exhibited satisfactory results in detecting Leishmania cells, also proving the leishmanicidal activity of these materials with different mechanisms observed.
In the present study, the immobilization of DRS 01 in conjunction with Plg allowed the addition of new chemical groups and properties to the film based on this biomolecule. This material may be able to detect other types of pathogens. Because of the antibacterial activity of DRS 01 (Reference Eaton, Bittencourt, Costa Silva, Véras, Costa, Feio and LeiteEaton et al., 2014), this perspective of application can be investigated by electrochemistry. Because ITO/Plg/DRS 01 film is a new material, the following steps of this study focused on characterizing it to direct future applications better.
Characterization of LbL Films by UV-Vis
Absorbance analysis in the UV-Vis region allowed us to monitor the growth of the film by measuring light absorption, which is directly related to film thickness. This analysis could indicate the continuity of the formation process of the LbL film after each stage of adsorption.
The growth dependence of ITO/Plg/DRS 01 films on peptide adsorption as a function of the number of bilayers is illustrated in Fig. 6. The insert represents the correlation between absorbance at 280 nm and the number of adsorbed bilayers. A linear increase in absorbance up to the fourth adsorbed bilayer was observed (Fig. 6). The DRS 01 adsorption is believed to occur on the surface and in the microchannels of the Plg fibrous clay. After five bilayers, the maximum incorporation of DRS 01 was reached, as shown by the absorbance saturation observed, and ordered layering begins to fall off. This result supports using the four-bilayer film because it is still in the region of ordered growth. In addition, many LbL films for electrochemical sensing applications in the literature point to four bilayers as the ideal number (Reference de Farias, Nogueira and S. S., de Oliveira Farias, A. M.Farias et al., 2017; Reference Plácido, de Oliveira Farias, Marani, Vasconcelos, Mafud, Mascarenhas, Eiras, Leite and Delerue-MatosPlácido et al. 2016).
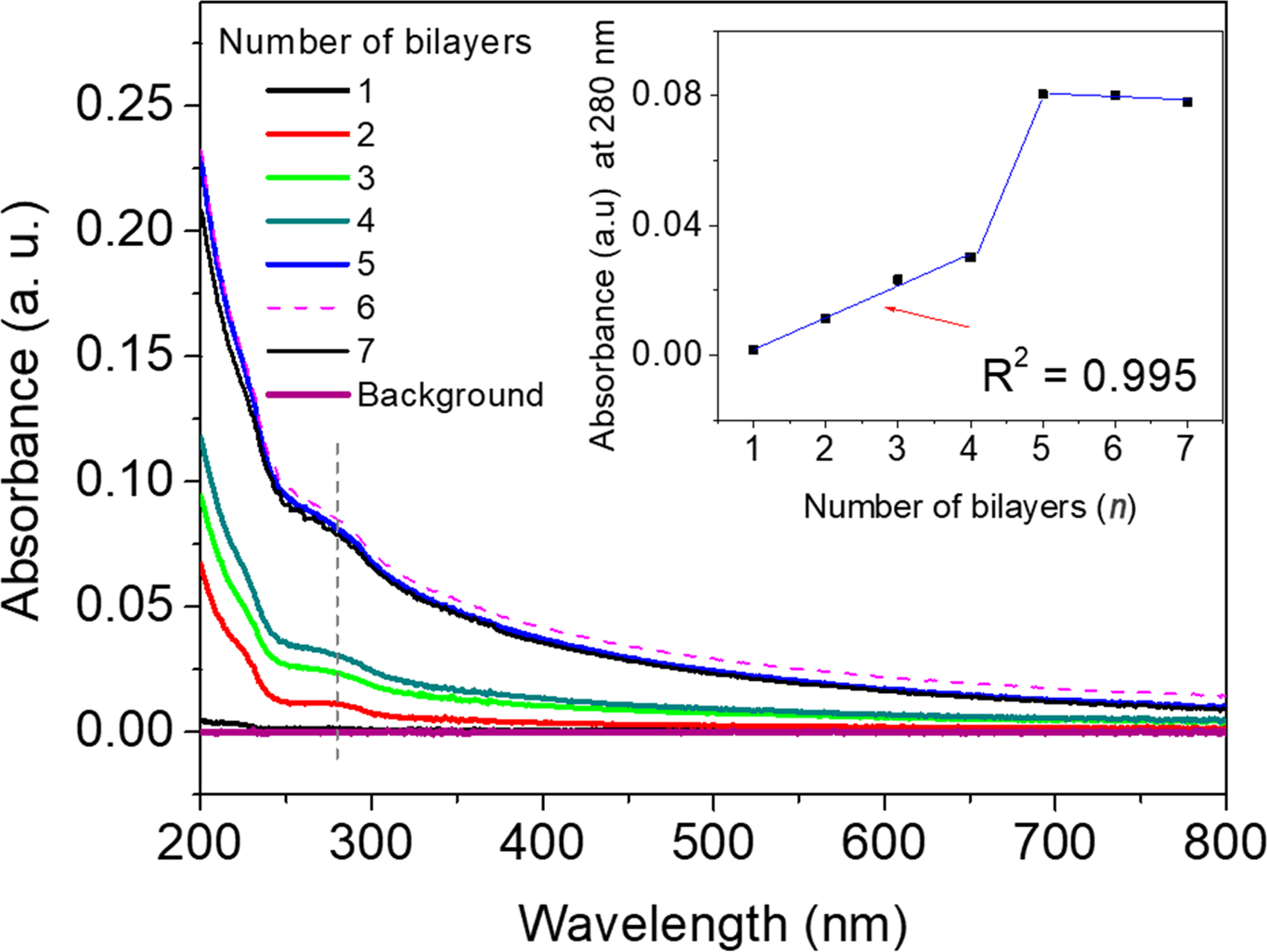
Fig. 6 UV-Vis spectra show the growth of ITO/Plg/DRS 01 with different numbers of bilayers. Insert: the relation between absorbance at 280 nm and the number of adsorbed bilayers
Characterization of LbL Films by Atomic Force Microscopy (AFM)
The deposition technique and materials that influence the morphology and roughness of the thin films were investigated by AFM analysis (Fig. 7).The characteristics observed for ITO used as the control (Fig. 7A) agreed with the AFM analyses reported by Reference Eaton and WestEaton and West (2010), which concluded that the surface consisted of small, globular granules. Concerning the height of the ITO substrate, the value found (51 nm) resembled that reported by Reference Bittencourt, de Oliveira Farias, Bezerra, Véras, Silva, Costa, Bemquerer, Silva, de Almeida, Leite and EirasBittencourt et al. (2016). The ITO surface covered with one layer of Plg (Fig 7B) had a more uniform appearance due to the coverage of the interstices and granules in a homogeneous way without producing a change in the height (51 nm).
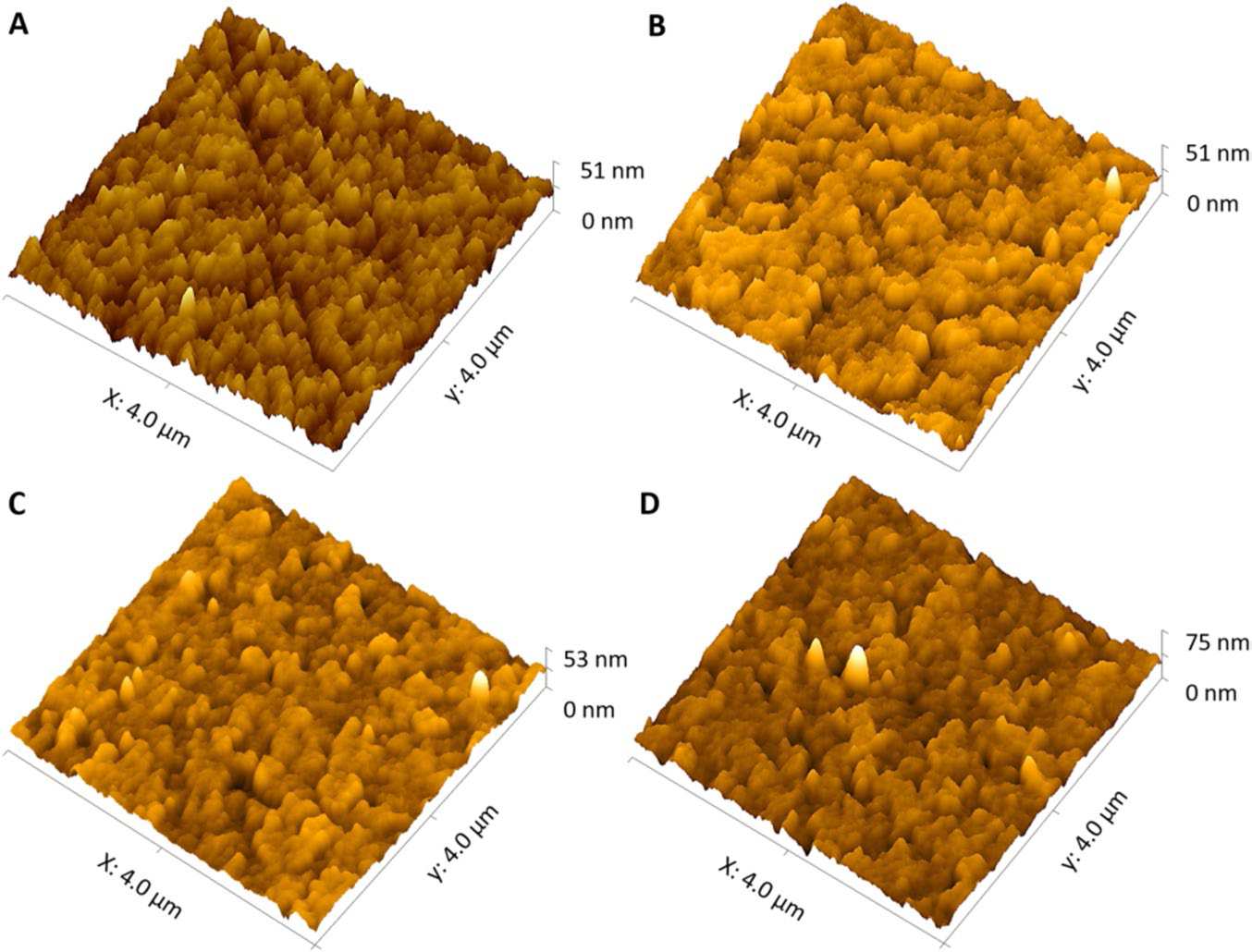
Fig. 7 AFM images of (A) ITO, and ITO coated with (B) ITO/Plg, (C) ITO/(Plg/DRS 01)1, and (D) ITO/(Plg/DRS 01)3 (Subscript number refers to the number of bilayers adsorbed on the substrate)
This result was due to the fine particle-size fraction of Plg obtained by purification, enrichment, and sonication. For the ITO/Plg/DRS01 film with one bilayer (Fig. 7C), its height increased, and visualization of the cracks decreased slightly. The surface of the ITO/Plg/DRS01 film with three bilayers (Fig. 7D) had a more regular coverage of the granules than the film with one bilayer, with some peaks at the top related to deposition.
Reference Decher and SchlenoffDecher and Schlenoff (2012) established that film roughness and uniformity influence directly the formation of the subsequent layer, as well as properties such as thickness, so the surface roughness of the two films was analyzed here (Fig. 8). The average roughness (Ra) value determined by AFM at 4 μm × 4 μm for the ITO was 3.75 ± 0.20 nm. Adding one layer of Plg to the film resulting in virtually no change in roughness (3.62 ± 0.28 nm, with no significant difference at p <0.05) is attributed to the small size of the particles. For the ITO-Plg/DRS 01 film with one bilayer, the change in Ra (3.02 ± 0.48 nm) was signficant (p <0.05) when compared to the value found for the ITO. The difference was even greater for the film with three bilayers (Ra = 4.37 ± 0.77 nm) in relation to ITO and other films and, thus, it could be chosen for biotechnological applications.
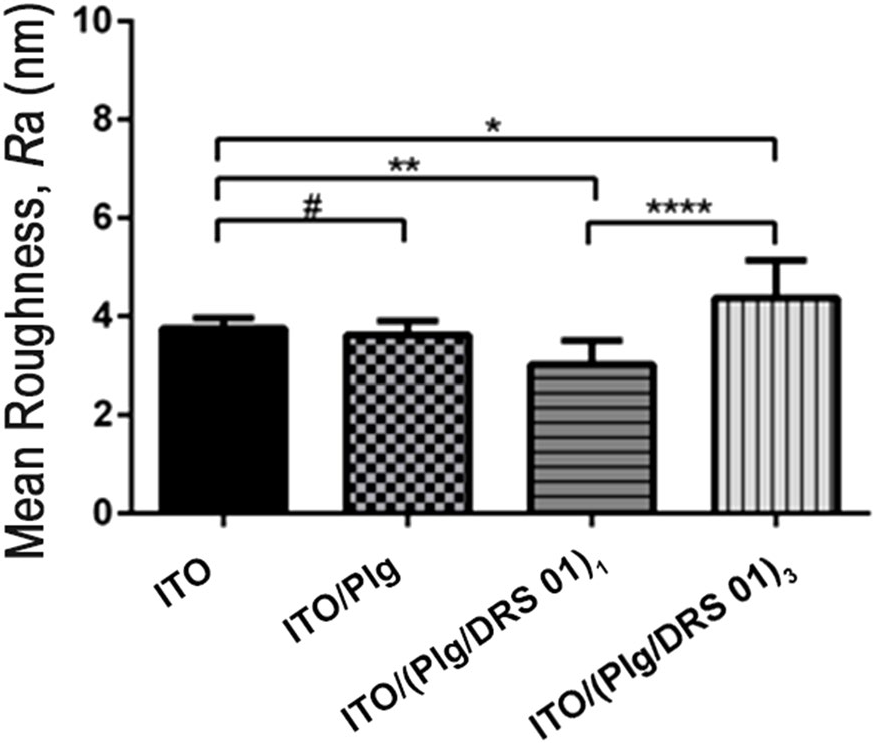
Fig. 8 Comparison of the roughness values (Ra) of the glass surfaces covered with ITO, ITO/Plg, ITO/(Plg/DRS 01)1, and ITO/(Plg/DRS 01)3. */**/****: statistically significant differences, #: no statistically significant difference (n = 10)
Conclusions
The results indicate that the Plg from Guadalupe in the State of Piauí, Brazil, was purified as confirmed by XRD, FTIR, and CV techniques. This study allowed a new use of raw Plg by improving its characteristics using simple and efficient techniques and a Layer-by-Layer self-assembly process with a biomedically relevant peptide. The LbL films thus obtained can be reproduced even on other substrates due to the good non-covalent interaction between the materials and to the reproducibility of the LbL technique. The purification of clay minerals plays an essential role in the electrode response during cyclic voltammetry. It provides new scientific results, such as its influence on the electrochemical characteristics of the few-layered assemblies. The binding of the DRS 01 peptide to Plg presents opportunities for developing functionalized sensors for rapidly detecting pathogens or treating substrates against biofilm formation with applications in the pharmaceutical, biomedical, and environmental areas. Besides, antimicrobial properties, among other biotechnological applications, can also be explored.
Acknowledgments
The authors are grateful to the ‘Coordination of Improvement of Higher-Level Personnel’ (CAPES) for the logistical and financial support and to Katiane C. M. Xavier (CETEM-PI, UESPI) for FTIR measurements of Plg.
Declarations
Conflict of Interest
The authors declare no conflict of interest.