Introduction
Aflatoxins are highly toxic metabolites produced by fungi Aspergillus flavus and Aspergillus parasiticus. These fungi contaminate a variety of grains, food, and feedstuffs and, therefore, cause health concerns and economic losses. The US Food and Drug Administration has established the maximum permitted concentration of aflatoxins present in food as 20 ng g−1. Contaminated products that exceed this limit can be directed to animal feed applications with restrictions if the aflatoxin content is <300 ng g−1. Contaminated grain with 300–500 ng g-1 of aflatoxin is usually blended with grain containing low levels of aflatoxins. Grain containing >500 ng g−1 aflatoxins must be destroyed.
Many chemical, physical, and biological methods have been proposed to deactivate, degrade, or remove aflatoxins from contaminated feed (Phillips et al., Reference Phillips, Clement, Park and Eaton1994). Among these techniques, incorporating clay minerals into animal diets as adsorbents of aflatoxin appeared to be an economical and practical detoxification measure in diverse animal trials. Numerous in vivo animal trials have been conducted to evaluate the efficacy of bentonites in ameliorating the aflatoxin toxicity (Bailey et al., Reference Bailey, Latimer, Barr, Wigle, Haq, Balthrop and Kubena2006; Kubena et al., Reference Kubena, Harvey, Huff and Corrier1990; Lindemann et al., Reference Lindemann, Blodgett, Kornegay and Schurig1993; Phillips & Carpenter, Reference Phillips and Carpenter2008; Phillips et al., Reference Phillips, Kubena, Harvey, Taylor and Heidelbaugh1987; Pimpukdee et al., Reference Pimpukdee, Kubena, Bailey, Huebner, Afriyie-Gyawu and Phillips2004; Ramos & Hernandez, Reference Ramos and Hernandez1996, Rosa et al., Reference Rosa, Miazzo, Magnoli, Salvano, Chiacchiera, Ferrero and Dalcero2001; Thieu & Pettersson, Reference Thieu and Pettersson2008). Adding bentonite to aflatoxin-contaminated feed reduced the concentration of aflatoxin M1, an aflatoxin B1 metabolite, in milk from dairy cows (Maki et al., Reference Maki, Thomas, Elmore, Romoser, Harvey, Ramirez-Ramirez and Phillips2015) and from goats (Ramos & Hernandez, Reference Ramos and Hernandez1996). The effectiveness of clay minerals in protecting animals from aflatoxicosis, without any adverse effects from the clays, was the basis for recent clinical trials. When the adsorbent was offered as a supplement or combined with food, the biomarkers indicated that aflatoxin exposure was reduced (Phillips et al., Reference Phillips, Wang, Elmore, Hearon and Wang2019).
In addition to bentonites, natural zeolites have also shown aflatoxin adsorption in solution (Dakovic et al., Reference Dakovic, Tomasevic-Canovic, Dondur, Vujakovic and Radosevic2000; Tomasevic-Canovic et al., Reference Tomasevic-Canovic, Dakovic, Markovic and Stojsic2001) and protection against aflatoxin metabolization in vitro (Katsoulos et al., Reference Katsoulos, Karatzia, Boscos, Wolf and Karatzias2016; Oguz et al., Reference Oguz, Kececi, Birdane, Onder and Kurtoglu2000). In some animal experiments, clinoptilolite was added to the feed at concentrations >0.5% used commonly for bentonites (Oguz et al., Reference Oguz, Kececi, Birdane, Onder and Kurtoglu2000). Unlike smectites, zeolites cannot expand substantially upon hydration. The cages and tunnels in zeolites are generally believed to be too small for aflatoxin access and, therefore, the adsorption of aflatoxin in zeolite is limited to the external surfaces only.
The detoxification effect of the clay minerals was attributed to the presence of smectites in most of the commonly reported clays or binders. Smectites adsorb aflatoxin in the gastrointestinal tract before the toxin can be assimilated and metabolized by the animals (Phillips et al., Reference Phillips, Kubena, Harvey, Taylor and Heidelbaugh1987). Yet, in vitro studies have shown high variations of aflatoxin adsorption capacity among clay mineral samples. Kannewischer et al. (Reference Kannewischer, Arvide, White and Dixon2006) tested twenty bentonite samples and observed that the aflatoxin adsorption capacity varied 10-fold among the bentonite samples, which implied different aflatoxin adsorption effectiveness of the bentonites in inactivating aflatoxins in vivo.
The selectivity and adsorption capacity of clay minerals for aflatoxins appear to be determined by the interaction of the aflatoxin molecules with the surface of the smectites (Tenorio Arvide et al., Reference Tenorio Arvide, Mulder, Barrientos Velazquez and Dixon2008). The size and polarity matching between the aflatoxin molecules and the adsorbing sites in the interlayer of smectite was demonstrated to be the key factor (Deng et al., Reference Deng, Velázquez, Billes and Dixon2010, Reference Deng, Liu, Luisa Barrientos Velazquez and Dixon2012; Deng & Szczerba, Reference Deng and Szczerba2011). An adequate structural charge density and the type of exchange cations are important considerations for smectite-based binders used in animal feed.
Most of the animal trials have shown that clay minerals could reduce aflatoxicosis, but a 100% recovery was not achieved regardless of the level of aflatoxin contamination. These lower-than expected recovery efficacies might be caused by side effects of the clays or inadequate incorporation methods in the diet. The presence of heavy metals or other impurities in the clays could be concerns but it appeared that animal feed and human clinical trials have indicated that clays are generally safe. Other potential causes are inadequate clay incorporation methods that could not deal with the high heterogeneity of aflatoxins distribution in the contaminated feed, or the slow interaction kinetics of the clays with the aflatoxins in the gastric fluids. The first objective of the present study was to evaluate the mineral and chemical compositions of two selected bentonites to find potential minerals or elements that can represent a health-concern when the bentonites are incorporated in animal feed. The second objective was to characterize the adsorption of aflatoxin B1 (AfB1) by the bentonites such as adsorption selectivity, capacity, and reversibility, and interlayer accessibility. The last objective was to evaluate the safety and efficacy of selected clays as amendments of aflatoxin-contaminated feed for broiler chickens.
Materials and Methods
Based on preliminary characterization of bentonites from Gonzales, Fayette, and Kernes counties in Texas (Barrientos Velazquez, Reference Barrientos Velazquez2011), and quantification of their aflatoxin adsorption capacity, two bentonites with the highest AfB1 adsorption were selected for this study. The bentonite samples 1TX and 4TX (20 kg of each) were provided by BYK additives (Gonzales, TX) (formerly Southern Clay Products) from the production line of the mining company.
Mineralogical Characterization
Chemical composition
Total elemental compositions of the two bentonites were determined using X-ray fluorescence spectroscopy by Mineral Lab, Inc. (Golden, Colorado). The bulk samples were analyzed for cation exchange capacity (CEC), exchange cation composition, and organic-matter content following the procedures described in the Soil Survey Laboratory Method Manual (Soil Survey Staff, 2004). The pH values of the bulk samples were determined at a 5:1 DI water:sample ratio.
Size fractionation
The bentonite samples were size-fractionated for detailed mineral analysis following the procedures by Deng et al. (Reference Deng, White and Dixon2013). Ten grams of each bentonite was treated with 20 mL of pH 5 sodium acetate solution to ensure Na+ saturation for better dispersion. Sample 4TX was treated with 30% H2O2 (Reagent ACS) to remove organic matter by adding 10 mL of hydrogen peroxide into the suspension containing the sample plus sodium acetate. The H2O2 treatment was omitted in sample 1TX to avoid oxidation of pyrite. The clays were then dispersed in dilute, pH 10 sodium carbonate solution. The sand fraction was wet separated with a 53-μm sieve. The silt and clay fractions were separated by centrifugation (Kunze & Dixon, Reference Kunze, Dixon and Klute1986). The collected clay suspensions were flocculated by adding an excess of NaCl. Once the flocculated clay particles settled, the supernatant was removed and the flocculated clay particles were transferred to dialysis tubing (VWR) and placed in DI water to remove the excess salt (Deng et al., Reference Deng, White and Dixon2013).
Mineral identification
For X-ray diffraction (XRD) analysis, the sand and silt fractions were ground to pass through a 140-mesh sieve and front loaded into 25-mm diameter cavities of XRD sample discs. The clay suspensions containing 50 mg of the clay were saturated with Mg by mixing the clay suspension with 0.5 M MgCl2 followed by shaking and centrifugation. The saturation was done three times and the clay dispersions were washed twice with DI water. The Mg-clays were air-dried on glass discs to enhance the d 001 diffraction of phyllosilicate minerals. The Mg-saturated films were misted with a 20% glycerol solution using an air spray to obtain glycerol-solvated films. The XRD patterns were recorded on a D8 Bruker Advance diffractometer (Bruker AXS GmbH, Karlsruhe, Germany) with a 1-D position-sensitive LynxEye detector (Bruker AXS GmbH, Karlsruhe, Germany) and CuKα radiation operated at 35 kV and 45 mA.
Infrared spectra of the clay fractions were recorded in diffuse reflectance mode and were used to determine the cation composition in the octahedral sheets of the smectites. Powder samples were prepared by mixing 0.01 g of clay with 0.3 g of KBr for Fourier-transform infrared (FTIR) spectroscopy analysis. The FTIR spectra were recorded at a resolution of 4 cm−1 from 450 to 4000 cm−1 on a PerkinElmer Spectrum 100 infrared spectrometer (PerkinElmer, Waltham, Massachusetts, USA). Sixty-four scans were recorded and averaged for each specimen.
Aflatoxin Adsorption and Stability in Bulk Bentonite and Clay Fractions
Aflatoxin adsorption was conducted on both bulk bentonites and on their clay fractions following the procedure described by Kannewischer et al. (Reference Kannewischer, Arvide, White and Dixon2006). Diluted bentonite (or the clay fraction) dispersions were prepared by dispersing 0.01 g of each bentonite in 5 mL of deionized water. The adsorption isotherms were prepared by transferring 50 μL of the suspension containing 0.1 mg of bentonite clay to a series of 15 mL polypropylene centrifuge tubes. Then, predetermined amounts of 8-ppm aflatoxin solutions and water were added to the 15-mL centrifuge tubes to achieve 5.0 mL of the following aflatoxin concentrations in duplicates: 0.0, 0.4, 1.6, 3.2, 4.8, 6.4, and 8.0 mg L–1. The 8 mg L–1 aflatoxin solution was prepared by diluting a 1000 mg L–1 AfB1/acetonitrile stock solution with deionized water. The stock solution was prepared by dissolving 50 mg of aflatoxin from Aspergillus flavus (Sigma-Aldrich Inc. St. Louis, Missouri, USA) in 50 mL of acetonitrile. After overnight shaking, the samples were centrifuged at 4500 rpm (5440×g based on formula (rpm)2r/g, where r is the radius) for 57 min. The aflatoxin concentration left in solution was analyzed using a Beckman Coulter DU 800 UV-spectrophotometer (Beckman Coulter Inc., Brea, California, USA) at 365 nm. The adsorption isotherms were fitted with the Langmuir equation to obtain the maximum adsorption capacity and the affinity constant Kd, with goodness-of-fit values,η2, calculated based on a normal non-linear least-squares regression method (Deng et al., Reference Deng, Liu, Luisa Barrientos Velazquez and Dixon2012; Schulthess & Dey, Reference Schulthess and Dey1996).
The clay dispersion of each bentonite sample was saturated with Na or Ca to investigate the effect of common exchange cations on aflatoxin adsorption. To obtain enough AfB1-Na-clay complexes for later XRD and FTIR analysis, the quantities of the Na-clays and the volumes of aflatoxin solutions were scaled up 10 times. Due to the requirement of large quantities of the aflatoxin solutions with this scale-up, aflatoxin adsorption on the Na-clays was not duplicated at each concentration. To investigate the resistance of adsorbed aflatoxin to washing, two aflatoxin-Na-clay complexes that had different aflatoxin loadings from the adsorption experiments were selected, transferred to 15 mL centrifuge tubes, and subjected to four successive washings with deionized water. The complexes were mixed with deionized water, shaken overnight, and centrifuged. The aflatoxin concentration in the supernatant after each centrifugation was quantified using the UV spectrophotometer to calculate aflatoxin desorption.
One of the water-washed aflatoxin-Na-clay complexes from each sample 1TX and 4TX was characterized with XRD and FTIR at nearly 0% and room humidity to verify intercalation of aflatoxin into the smectites. Each aflatoxin-clay complex dispersion was air dried on a zero-background quartz slide and the slide was mounted in an XRD 900 reactor chamber (Anton Paar Gmbh, Graz, Austria) for variable temperature XRD analysis.
One XRD pattern was recorded at room humidity (~40%) at 30°C, and a second pattern was recorded at nearly 0% humidity, which was achieved by alternative N2 flushing and evacuating the chamber for 20 min. During XRD recording at 0% humidity, the chamber was filled with dry N2.
Infrared spectra of the aflatoxin-clay complexes were recorded to confirm the stability of the adsorbed aflatoxin and to check bonding strength. After recording XRD patterns, the AfB1-clay complexes were transferred into centrifuge tubes, redispersed in water, and air dried on ZnS windows (ClearTran, International Crystal Labs, Garfield, New Jersey, USA). Infrared spectra were recorded with dry N2 purge in the transmission mode. Thirty-two scans of 1 cm−1 resolution were recorded for each complex and were averaged.
In vivo Poultry Experiment
To verify the detoxification efficacy in vivo of the clays, the bulk bentonites were introduced into chicken feed contaminated by Aspergillus parasiticus. Corn containing 803 ng g−1 aflatoxin was harvested from an experimental field at the Texas AgriLife Research Farm, College Station, Texas, USA. Another highly-contaminated corn containing 6250 ng g−1 aflatoxin was obtained by inoculating a corn with Aspergillus parasiticus cultures (supplied by Dr. Deepak Bhatnagar, ARS-USDA, New Orleans, Louisiana, USA) under high humidity and warm conditions. Both corn batches were ground and mixed into a chicken feed to achieve a final concentration of >1000 ng g−1 of aflatoxin in the feed. “Clean” corn was used as a control in the feed which had an aflatoxin concentration of <20 ng g−1. The amounts of soybean meal, vitamins, salts, and other ingredients in the feeds were the same as those reported by Bailey et al. (Reference Bailey, Latimer, Barr, Wigle, Haq, Balthrop and Kubena2006). Aflatoxin concentrations (B1+B2+G1+G2) in the final feeds were quantified by the Office of the Texas State Chemist using high-performance liquid chromatography (OTSC, 2011). The control feed (clean corn) had an aflatoxin concentration of <20 ng g−1 and the contaminated feed contained 1400 ng g−1of aflatoxin. The clean feed and the aflatoxin-contaminated feed were subject to three clay treatments: (1) no clay, (2) 0.5% (w/w) Bentonite 1TX, and (3) 0.5% (w/w) Bentonite 4TX. The bentonite samples were added as dry powders into the feed and homogenized using a horizontal rotary mixer.
The clean feed group or control group was used to identify any potential negative effects caused by the clays. The aflatoxin-contaminated group was used to evaluate the efficacy of selected clays to adsorb aflatoxin before it can be assimilated by the chickens. A total of 240 chickens were randomly assigned among the six treatments (2 aflatoxin levels×3 clay treatments).
For each dietary treatment, eight replications were employed with each replication composed of five chickens in one pen. The same housing and safety measures of Bailey et al. (Reference Bailey, Latimer, Barr, Wigle, Haq, Balthrop and Kubena2006) were followed in this study.
Chicken growth and mortality were monitored daily, and the weights of chickens were recorded weekly. After three weeks of feeding, all the chickens were sacrificed. The body weight and organ weights (liver, kidney, spleen, and heart) of each chicken were recorded. Five liver samples from each of the six treatments were collected. Aflatoxins AfB1, AfB2, AfG1, AfG2, and AfM1 in the livers were quantified by the Veterinary Diagnostic Laboratory at Iowa State University. An ANOVA F-test following a complete randomized design (CRD) was used to analyze the chicken-growth performance data.
Results
Characterization of Bentonites as Aflatoxin Adsorbents
Chemical and mineral compositions of bentonites 1TX and 4TX
Both bentonite samples (1TX and 4TX) contained significant proportions of clay-sized particles (>65.7%) and small amounts (<4%) of sand-sized particles (Table 1). The bentonite samples had small organic carbon contents which indicated that the calculated CEC represented only the mineral phase (Table 1). Bentonite 4TX had a greater CEC (81 cmol(+)/kg) than bentonite 1TX (74 cmol(+)/kg) due to higher clay content in the former sample. Calcium was the dominant exchangeable cation in both natural samples.
Table 1 Chemical and physical properties of bulk bentonites 1TX and 4TX

The total elemental composition analysis indicated that the concentrations of most heavy metals in the two bentonites were small (Table 2). Adding 0.5 wt.% of clay to the feed resulted in very small increments in the trace metals compared to the total tolerable levels of the heavy metals for poultry. Similar results were observed for most major elements except Si and Al (Table 3) as these two elements are the major structural components in smectites.
Table 2 Trace-element composition of bulk samples and maximum tolerable levels in a poultry diet
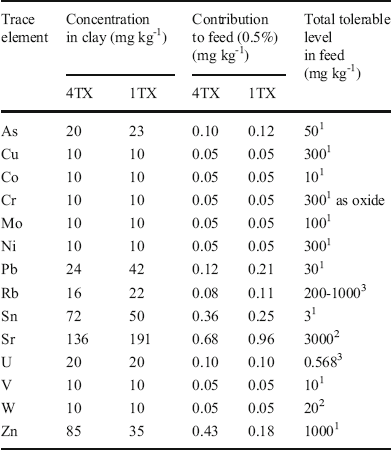
1 Ewing & Charlton (Reference Ewing and Charlton2005), 2 Pais & Jones (Reference Pais and Jones1997), 3National Research Council (2005)
Table 3 Major element composition of bulk samples and maximum tolerable levels in poultry diet

1 Ewing & Charlton (Reference Ewing and Charlton2005), 2 Pais & Jones (Reference Pais and Jones1997), 4 Georgievski (Reference Georgievski, Georgievski, Annenhov, Samokhin and Ifis1982).
Montmorillonite was the dominant clay mineral present in both bentonite samples (Figs 1 and 2). Montmorillonite was also identified in the sand and silt fractions as indicated by the broad d-value at ~12.0 Å, which suggested that some smectite clay remained as aggregates after the dispersion treatments. The ∼12.0 Å peak intensities suggested that bentonite 1TX contained more smectite aggregates than bentonite 4TX in the sand and silt fractions.

Fig. 1 XRD patterns of bulk bentonite, sand, and silt fractions of samples 1TX and 4TX

Fig. 2 XRD patterns of clay fractions after Mg saturation and glycerol treatment
Montmorillonite was identified based on the OH-stretching and bending vibration bands that revealed the cation compositions in the octahedral sheets of the smectites (Fig. 3, Left). The strong OH-stretching band at 3626 cm−1 indicated that Al3+ was the dominant cation in the octahedral sheets (Madejová, Reference Madejová2003). Both bentonite samples showed characteristic bands at 917–919 cm−1 for Al3+–OH, ∼885 cm–1 for Al3+Fe3+–OH, and ∼845 cm−1 for Al3+ Mg2+–OH (Gates, Reference Gates and Kloprogge2005). The relative intensities of these OH-bending bands suggested that Al was the major cation in the octahedral sheets of smectites in both bentonites and, therefore, montmorillonite was the major mineral (Madejová et al., Reference Madejová, Gates, Petit, Gates, Kloprogge, Madejová and Bergaya2017). The Al3+Fe3+–OH band at 885 cm−1 was better resolved in sample 4TX than in sample 1TX, which suggested more octahedral Fe cations in the montmorillonite of sample 4TX. The band at 794 cm−1 in bentonite 4TX was due to the structural iron (Gates, Reference Gates and Kloprogge2005). In bentonite 1TX, the 794 cm−1 band was attributed to the Si–O along with the 628 cm−1 band (Madejová & Komadel, Reference Madejová and Komadel2001), which supports the identification of opal-CT in the XRD pattern.

Fig. 3 Diffuse reflectance infrared spectra of the clay fractions of bentonite samples 1TX and 4TX recorded at room humidity (left) and the transmission FTIR spectra of the clay fractions after adsorbing AfB1 under N2 purge (∼0% humidity, right)
Both bentonites contained quartz, feldspars, and biotite (Fig. 1) mainly in the sand and silt fractions. Minor amounts of muscovite and clinoptilolite were present in sample 4TX and occurred mainly as silt-sized particles (Fig. 1). Quartz, feldspars, and micas are relatively inert minerals. Gypsum (CaSO4·2H2O) and pyrite were identified in bentonite 1TX (Fig. 1). Pyrite occurred mainly as silt-sized particles. Opal-CT was identified in the clay fraction of sample 1TX.
Aflatoxin adsorption by the bentonites and their clay fractions
The bulk bentonite 4TX showed a greater adsorption capacity (0.58 mol/kg) when compared to bentonite 1TX (0.43 mol/kg) (Table 4 and Fig. 4). When the clay fractions of the two bentonites were saturated with Na or Ca, the type of exchange cation (Na vs. Ca) in smectite altered the shape of the adsorption isotherms. The bulk bentonites and the Ca-saturated clays had the typical L-shaped isotherms (Fig. 4), whereas the Na-saturated clay fractions demonstrated nearly linear isotherms (Fig. 4). The adsorption isotherm of Na-saturated clays had much smaller initial slopes, which suggested a lower affinity of aflatoxin for the Na-saturated clays. The affinity constant (Kd) significantly decreased for the Na-clays, suggesting difficulty of aflatoxin molecules to reach adsorption sites. η2 values for all isotherms were ~0.9.
Table 4 Aflatoxin adsorption isotherm fitting parameters for bulk samples and clay fraction
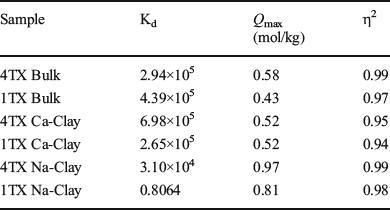

Fig. 4 Aflatoxin B1 adsorption on the original bulk samples, and on the <2 μm clay fractions saturated with Ca and Na of bentonites 1TX and 4TX
Stability of adsorbed aflatoxin to water washing
The aflatoxin molecules adsorbed on the clays were resistant to water washing, regardless of the initial aflatoxin loading on the clays (Fig. 5). When the aflatoxin-clay complexes from the adsorption isotherm experiment were washed with DI water, slightly more aflatoxin adsorption occurred in the first washing due to the presence of residual aflatoxin in the supernatant after the adsorption experiment. No distinct desorption was observed in the following three washings with DI water. The cumulative desorption after four washings accounted for <0.5% of the initially adsorbed aflatoxin B1 on the clays. This washing experiment indicated that aflatoxin adsorption on the clays were virtually irreversible.

Fig. 5 Aflatoxin B1 remaining on clays during four successive washings with DI water
Occurrence of interlayer aflatoxin adsorption
Adsorbed aflatoxin expanded the smectite basal spacing to ~14.0 Å, at room humidity (66%), and to ~13 Å at 0% humidity (Fig. 6) while the Na-saturated clays without aflatoxin showed basal spacings of ~12.0 Å at room humidity and a reduced ~10.0 Å at ~0% humidity (Fig. 6). The greater basal spacing in the aflatoxin-adsorbed clays confirmed that at least some of the adsorbed aflatoxin molecules were held in the interlayer space of these two clays. The FTIR spectra of the aflatoxin-clay complexes showed the presence of bands corresponding to the aflatoxin molecules (Fig. 3, right). The infrared bands of adsorbed aflatoxins were similar to those reported by Deng et al. (Reference Deng, Velázquez, Billes and Dixon2010) and, therefore, the same band assignments were followed for the adsorbed aflatoxin (Fig. 3).

Fig. 6 XRD patterns of Na-saturated clay fractions of bentonites 1TX and 4TX before and after adsorbing AfB1 at room humidity (∼40%) and under N2 purge (∼0% humidity)
In vivo Poultry Experiment
Growth Performance: Body weight, mortality, and feed conversion
Chickens in the control group (feed contained <20 ng g−1 aflatoxin, no clay) weighed 724 g, on average, at the end of the three-week feeding trial. Adding clays to the clean feed induced a slight decrease in average body weight of the birds: ~6.5% for 4TX and 6.8% for 1TX, respectively, but the differences were not significant statistically at the p < 0.05 level. No mortality was observed among the chickens fed with clean corn, with or without the clays in the feeds. The chickens that received the aflatoxin-contaminated feeds weighed only about half that of those which received the clean feeds (Table 5). Incorporating clay 4TX into the aflatoxin-contaminated feeds increased the body weight of the birds by 21% and incorporating clay 1TX increased it by 14%. The increases in body weight suggested that the clays provide protection from the aflatoxin toxicity. Chickens receiving the aflatoxin-contaminated feed had a mortality rate of 25%. Incorporating clays into the feed did not reduce the mortality of the chickens. A commonly used indicator of animal performance is the feed conversion ratio (FCR), which is the ratio of feed to the weight gained from consumption. A statistical difference was observed in the FCR between the group fed clean corn without clay and the group where sample 1TX was added. No statistical differences were found between the treatments in each group, possibly due to FCR not being a sensitive parameter for this study (Table 6).
Table 5 Effects of bentonites on feed conversion ratio and body weight

Data in a group with different letters (ab) are significantly different at p < 0.05
Table 6 Effects of bentonites on organ-body weight ratios

(ab) Data in columns with different superscripts are significantly different at p < 0.05
Relative organ weights and liver appearance
The relative spleen and kidney weights of the birds that received aflatoxin-contaminated feed were twice those that received clean feeds, and the relative liver and heart weights of the birds fed aflatoxin-contaminated feed were 40 to 50% greater than those that received clean feed. In the current experiment, adding clays into the feeds did not show significant changes at the p < 0.05 level in terms of the relative weights of liver, heart, kidney, or spleen within each of the two groups of treatments (clean corn vs. aflatoxin-contaminated corn). Livers from chickens subjected to the clean diet had a similar healthy dark red color and similar size (Fig. 7). Adding clays to the clean feed did not show any influence on the size, color, or other appearance of the livers. Livers from chickens fed with the high-aflatoxin diet were much smaller, were pale yellow in color, and were more rigid to the touch.

Fig. 7 Representative livers of 3-week old birds that received clean and aflatoxin-contaminated feeds
Aflatoxin content in livers
No aflatoxin was detected in livers from the birds that received the clean feeds with or without the clays. Aflatoxin residues and metabolites were detected in the livers of birds that received aflatoxin-contaminated feed (Table 7). Aflatoxins in the livers occurred mainly as AfB1 and AfG1, which are the major forms of aflatoxin present in feeds that can be metabolized to AfB2, AfG2, and AfM1. Adding clays into the aflatoxin-contaminated feed reduced aflatoxin contents in the livers but did not eliminate them completely. Among the five tested aflatoxins, only AfB1 and AfG1 showed significant differences (p < 0.05) between the no clay and clay treatments. The total aflatoxin content was significantly smaller in the aflatoxin-feed with clay treatment than in the aflatoxin feed with no clay (Table 7). Adding the two clays to the aflatoxin-contaminated feed reduced the total aflatoxins by 37%.
Table 7 Effects of bentonites on aflatoxin concentration in chicken livers*

*Aflatoxin concentrations in livers of chickens fed with clean corn, regardless of clay use, were below detection limits.
ab Data in columns with different superscripts are significantly different at p < 0.05
Discussion
Effect of Physical and Chemical Properties of Bentonites on Aflatoxin Adsorption
The slightly acidic pH in bentonite 1TX was due to the presence of pyrite that can produce sulfuric acid upon weathering, while the pH of sample 4TX was in the range previously observed for bentonites (Senkayi et al., Reference Senkayi, Dixon, Hossner, Abder-Ruhman and Fanning1984). The CEC values of both bentonites suggested a high clay content that was confirmed by the size fractionation. Bentonite 1TX could be expected to have less adsorption capacity than bentonite 4TX based on the large proportion of of sand-sized particles.
In addition to smectite, quartz, feldspars, and biotite were common minerals present in both bentonite samples, which are considered inert minerals. Clinoptilolite was identified in bentonite 4TX. This zeolite mineral is used commonly in animal feed as a feed additive and its occurrence in the bentonite is not likely to cause health concerns (Kraljević Pavelić et al., Reference Kraljević Pavelić, Simović Medica, Gumbarević, Filošević, Pržulj and Pavelić2018). On the contrary, the presence of clinoptilolite can potentially favor aflatoxin adsorption as some studies have demonstrated the capacity of this zeolite to adsorb aflatoxins (Dakovic et al., Reference Dakovic, Tomasevic-Canovic, Dondur, Vujakovic and Radosevic2000; Tomasevic-Canovic et al., Reference Tomasevic-Canovic, Dakovic, Markovic and Stojsic2001). Minerals present in bentonites, such as opal-CT in sample 4TX and gypsum and pyrite in sample 1TX, can potentially have some negative health effects. Heavy metals can be associated with minerals such as pyrite (Reyes et al., Reference Reyes, Gutiéttez and Joya2020). Opal-CT often occurs as nanometer-sized particles. Gypsum has a relative high solubility which may affect the electrolyte concentrations, once ingested by animals. The health effects of gypsum, pyrite, and opal-CT deserve more consideration when bentonite is used in animal feed.
As the silicate minerals such as montmorillonite, quartz, and feldspars are relatively stable in the digestive system, the large concentrations of Si and Al are probably not a concern. In the feed industry, up to 2 wt.% bentonite can be added to the feed to facilitate feed processing, and no adverse effects from the clays have been reported in animals (Phillips et al., Reference Phillips, Sarr and Grant1995; Quisenberry, Reference Quisenberry1968). The type of smectite can also affect the amount of aflatoxin adsorption. The evaluation of six smectite samples showed that montmorillonite and hectorite were more effective at adsorbing aflatoxin in solution than saponite, nontronite, and beidellite (Barrientos-Velazquez et al., Reference Barrientos-Velazquez, Marroquin Cardona, Liu, Phillips and Deng2016b).
The two bentonites and their clay fractions were capable of adsorbing aflatoxins. Their adsorption capacity and affinity for aflatoxin appeared to be determined by the smectite content and the type of exchange cation on the clays. The clay fraction showed a slightly greater aflatoxin adsorption than the bulk bentonite samples due to the removal of non-reactive minerals. The effects of Na- and Ca-clay saturation on aflatoxin adsorption were corroborated in a study by Deng et al. (Reference Deng, Liu, Luisa Barrientos Velazquez and Dixon2012): divalent cation saturation (Ca and Ba) resulted in greater affinity and greater adsorption capacity for aflatoxin due to a better charge density, size, and polarity matching between the adsorbing sites and aflatoxin molecules in the interlayer of smectite. Na-saturated smectites showed low aflatoxin adsorption, which was attributed to the large hydration ratio of Na+ interfering with the ion-dipole interaction of the exchange cation and the carbonyl oxygen on aflatoxin.
The in vitro aflatoxin adsorption experiment suggested that aflatoxin could be strongly adsorbed by smectites present in natural bentonites. As Ca-saturation induced much greater adsorption affinity, and the exchange cations of the natural bentonites were dominated by Ca, the bentonites should be effective at deactivating aflatoxin toxicity when properly used in animal feed.
Efficacy of Bentonites to Adsorb Aflatoxin in vivo
The three-week poultry experiment showed that both bentonites were effective at deactivating aflatoxin to some extent but a full recovery from the aflatoxin toxicity was not achieved. In the feed treatments with clean corn, adding clays into the feeds led to slightly reduced body weight and nearly the same relative organ weights and organ appearance. A similar small weight reduction after adding clays into feeds was observed in other animal experiments (Bailey et al., Reference Bailey, Latimer, Barr, Wigle, Haq, Balthrop and Kubena2006; Kubena et al., Reference Kubena, Harvey, Huff, Elissalde, Yersin, Phillips and Rottinghaus1993) but the explanation for this reduction was not addressed. The addition of 0.5% clay to the diet may have caused a slight dilution of the nutrient content. The clays may also adsorb some essential nutrients such as vitamins (Barrientos-Velazquez et al., Reference Barrientos-Velazquez, Arteaga, Dixon and Deng2016a) and micronutrients (Elliott et al., Reference Elliott, Connolly and Kolawole2020). Overall, the low mortality and body weight results in the clean corn diet suggested that the clays were largely safe to use, despite the presence of pyrite and opal-CT in bentonite 1TX.
The presence of aflatoxin in the feed severely inhibited the feed intake and growth of chickens. Adding clays to the aflatoxin-contaminated feed led to increased body weights, reduced liver aflatoxin content, and enhanced liver appearance. One of the most characteristic signs of aflatoxicosis is the deterioration of liver as illustrated by the enlargement of liver size relative to body size due to fat accumulation (Aletor et al., Reference Aletor, Kasali and Fetuga1981; Leeson et al., Reference Leeson, Diaz and Summers1995; Miazzo et al., Reference Miazzo, Peralta, Magnoli, Salvano, Ferrero, Chiacchiera and Dalcero2005; Phillips et al., Reference Phillips, Kubena, Harvey, Taylor and Heidelbaugh1988). Aflatoxicosis can also increase the relative size of heart, kidney, and spleen, resulting in the increased relative organ weights to body weight (Bailey et al., Reference Bailey, Kubena, Harvey, Buckley and Rottinghaus1998; Huff et al., Reference Huff, Kubena, Harvey and Phillips1992; Quezada et al., Reference Quezada, Cuéllar, Jaramillo-Juárez, Valdivia and Reyes2000). The increased relative organ weight (spleen, kidney, liver, and heart) in birds fed an aflatoxin-contaminated diet compared to those in a low-aflatoxin diet were similar to early observations on broiler chickens (Aletor et al., Reference Aletor, Kasali and Fetuga1981; Bailey et al., Reference Bailey, Latimer, Barr, Wigle, Haq, Balthrop and Kubena2006; Huff et al., Reference Huff, Kubena, Harvey and Phillips1992; Tessari et al., Reference Tessari, Oliveira, Cardoso, Ledoux and Rottinghaus2006).
Adding clays in the aflatoxin-contaminated feed improved the size of the liver, enhanced the color to a more reddish healthy appearance, and made the livers less rigid. A full recovery, however, was not achieved. This observation was in agreement with experiments reported by others (Aletor et al., Reference Aletor, Kasali and Fetuga1981; Leeson et al., Reference Leeson, Diaz and Summers1995; Miazzo et al., Reference Miazzo, Peralta, Magnoli, Salvano, Ferrero, Chiacchiera and Dalcero2005; Phillips et al., Reference Phillips, Kubena, Harvey, Taylor and Heidelbaugh1988). The present study showed results similar to those obtained by Bailey et al. (Reference Bailey, Latimer, Barr, Wigle, Haq, Balthrop and Kubena2006), where the addition of 0.5% of HSCA clay to a feed containing ∼3600 ng g−1 of aflatoxin showed no significant differences in relative liver weight. Fowler et al. (Reference Fowler, Hashim, Barrientos-Velazquez, Deng and Bailey2014) also observed that adding 0.2 wt.% bentonite effectively reduced aflatoxins in the liver. The bentonite samples provided some protection from aflatoxin toxicity but the differences between chickens with the clean feed and aflatoxin-contaminated feed, however, suggested that adding clays to the feed as dry powder did not result in a 100% toxicity recovery in the current experiment. This observation is consistent with most animal experiments reported in the literature. The lower than expected toxicity recovery from aflatoxicosis indicate that several factors may have hindered the aflatoxin adsorption capacity of the clays in the gastric fluid. These interfering factors should be investigated further and minimized in practice. At least the effect of the following three factors should be addressed: (1) The dispersion status of the clays and solubility of aflatoxins in the gastrointestinal fluid could determine its efficiency. Poor dispersion of the clays and the low solubility (20–30 mg/L) of aflatoxins in aqueous solution could potentially restrict adsorption sites for aflatoxin. (2) Localization of aflatoxin contamination in the feed could diminish the performance of the clays. Aflatoxin contamination concentrated in localized spots where the fungus grows. The contaminated spots may have extremely high concentration (hundreds of ppm instead of ppb level) whereas the adjacent grain might be free of aflatoxin. If the clay particles were not located near the high-aflatoxin spots during the digestion, the toxins would have been absorbed by the birds before they had the chance to interact with the clay. Yet, a similar animal trial where bentonite suspensions were prepared and spray-applied to the aflatoxin-contaminated feed showed no significant improvement in the biological markers tested due to the low aflatoxin concentrations tested (<1000 ppb). (3) Competition for other biological compounds for adsorption sites on the clays.
The high adsorption values for the bentonites were obtained from batch adsorption experiments performed in ideal solutions (water) with no other organic compounds present in solution that can compete with aflatoxin for adsorption sites on the surface of the smectite. Previous in vitro experiments have shown competition of organic compounds, such as vitamins and proteins, with aflatoxin molecules resulting in a decreased adsorption capacity from the clay minerals (Barrientos-Velazquez et al., Reference Barrientos-Velazquez, Arteaga, Dixon and Deng2016a). When the aflatoxin adsorption capacity of the clay fraction of bentonite 4TX was evaluated in simulated gastric fluid, the adsorption capacity was reduced from 0.5 mol/kg in water to 0.2 mol/kg in simulated gastric fluid (Barrientos-Velazquez et al. Reference Barrientos-Velazquez, Arteaga, Dixon and Deng2016a). The decrease was attributed to the adsorption of pepsin, a protein, in the interlayer of the smectite which blocked the aflatoxin accessibility.
The results from the current poultry trial and those reported in the literature suggested that clay could reduce the toxicity of aflatoxins, but several practical challenges need to be addressed in future studies: (1) the opportunities for the clays to interact with aflatoxins must be enhanced. As the dry powder form of the clays in the feed and low solubility of aflatoxins do not offer much opportunity for them to interact with each other, better ways of dispersing the clays in the feed and more efficient methods to increase the solubility of aflatoxins in gastric fluid need to be explored. (2) Develop strategies to deal with the extreme heterogeneity of the contamination. As fungi contamination is often localized and the contaminated individual grains could contain aflatoxin that are thousands or millions time more concentrated than the average value, these highly contaminated grains can cause mortality when ingested. (3) Develop strategies to enhance the selectivity and efficiency of the clays for aflatoxin but limit the uptake of nutrients by the clays in gastric fluid.
Barrientos-Velazquez and Deng (Reference Barrientos-Velazquez and Deng2020) proposed the modification of smectites with organic nutrients in the interlayer to prevent the adsorption of large molecules such as proteins. The intercalation of vitamin B1 significantly increased the aflatoxin adsorption of montmorillonite in simulated gastric fluid.
Conclusions
The mineralogical and chemical characterizations indicated that both bentonite samples, 1TX and 4TX, were dominated by smectite with Ca as the dominant exchange cation and would be effective in binding aflatoxins and reducing aflatoxicosis. Elemental composition did not indicate special health concerns for the uses of the bentonites in animal feed. Hight aflatoxin capacities were observed on both bentonites and Ca-saturation performed better than Na-saturation. The in vivo poultry experiment showed that both clays were effective in reducing aflatoxicosis in broiler chickens by enhancing body weight and liver appearance, and by reducing aflatoxin contents in livers, but a 100% recovery from aflatoxicosis was not achieved. The large amount and heterogeneity of aflatoxin in the feed, the poor dispersion of clays in gastric fluid, and the possible interaction of the clay minerals with nutrients and proteins present in the gastrointestinal tract could be significant factors in reducing aflatoxin adsorption.
Acknowledgments
Research funding was provided by the National Corn Growers Association. The bentonite samples were provided by Southern Clay Products; in particular Geologist Charlie Smith facilitated sample collection and provided additional information on the mine sites and samples. To the Veterinary Diagnostic Laboratory at Iowa State University, the authors express appreciation for conducting the analysis on the liver samples.
Author Contribution
Ana Luisa Barrientos Velazquez, Youjun Deng: Manuscript writing, experimental design, data analysis and data collection.
Christopher A. Bailey: Manuscript review, animal trial experimental design, data analysis and data collection for the animal trial.
Akramul Haq: Animal trial experimental design, data analysis and data collection for the animal trial.
Justin Fowler, Radhika Kakani: Data collection for the animal trial.
Funding
National Corn Growers Association. Grant Number: 406398-1226; 406573-9266; 04022013.
Data availability
The datasets generated during the current study are available from the corresponding author on reasonable request.
Declarations
Ethics approval
Ethical approval: All procedures performed in studies involving animals were in accordance with the ethical standards of the institution at which the studies were conducted and ethical approval was obtained from Dr. John N. Stallone, Institutional Animal Care and Use Committee, Permit Number: IACUC 2014-0030.
Consent for publication
The authors agree to publication in Clays and Clay Minerals
Conflict of interest
The authors declare that they have no conflict of interest.