Introduction
Clays or clayey rocks have numerous applications and are important in various industrial and environmental sectors (Murray, Reference Murray1999; Bergaya & Lagaly, Reference Bergaya and Lagaly2006). In the ceramic industry, they play a key role in the production of complex products, as well as in processes used in the production of electronic components in advanced ceramics and in the production of blocks and bricks obtained from structural ceramics (Smoot, Reference Smoot1961). Several authors have studied clay deposits as a source of raw material for the ceramic-tile industry (Beltrán et al., Reference Beltrán, Sánchez, García-Ten and Ginés1996; Boix et al., Reference Boix, Gargallo, Jordan, Segura and Sanfeliu1994; Dondi, Reference Dondi1999; Dondi et al., Reference Dondi, Raimondo and Zanelli2014; Ferrari & Gualtieri, Reference Ferrari and Gualtieri2006; Fiori, Reference Fiori1996; Galos, Reference Galos2011; Kadır et al., Reference Kadιr, Erman and Erkoyun2011; Külah et al., Reference Külah, Kadir, Gürel, Eren and Önalgil2014; Manju et al., Reference Manju, Nair and Lalithambika2001; Zalba, Reference Zalba1979; Zanelli et al., Reference Zanelli, Dondi, Guarini, Raimondo, Domínguez, Iglesias and Dondi2008, Reference Zanelli, Iglesias, Dominguez, Gardini, Raimondo, Guarini and Dondi2015).
The Corumbataí Formation, a geological unit of the Paraná Basin of Permian age, contains one of the largest deposits of raw materials for the ceramic industry in Brazil (Christofoletti et al., Reference Christofoletti, Moreno and Motta2009; Motta et al., Reference Motta, Christofoletti, Garcez, Florêncio, Boschi, Moreno and Zanardo2004, Reference Motta, Christofoletti, Garcez, Florêncio, Boschi, Moreno, Del Roveri and Zanardo2005). It occurs widely in the State of São Paulo, and is used with great success in the Ceramic Cluster of Santa Gertrudes in the production of tiles, currently representing the largest ceramic center in the Americas, with an annual installed production of 812.29 million square meters per year of ceramic tile, having produced, in 2020, 538.32 million square meters, 87% being processed by dry grinding and 13% by wet (Aspacer, 2021).
Two types of mass -preparation processes are used in the manufacture of ceramic tile in Brazil: the dry process, more common, in which Ceramic Cluster of Santa Gertrudes leads the world. It uses the clayey rocks of the Corumbataí Formation as a unique raw material, where the mass is ground, moistened, and pressed, generating semi-porous products as the main product; the other process is the wet process, where the mass is composed of a combination of raw materials, where these are fractionated and granulated through a ‘spray dryer’ (atomizer), generating stoneware and porcelain as the main products.
Because of its economic importance, several studies have been carried out in the deposits near Santa Gertrudes Ceramic Cluster in order to understand the geological, geochemical, and technological characteristics. The facies concept was applied by Christofoletti et al. (Reference Christofoletti, Moreno and Batezelli2006), following initial description by Miall (Reference Miall1994), to the description and mapping of ceramic lithofacies in addition to technological, mineralogical, and chemical identification of the properties. Other studies focused on other discoveries (Azzi et al., Reference Azzi, Marek Osacký, Uhlík, Mária Čaplovičová, Zanardo and Jana Madejová2016; Christofoletti et al., Reference Christofoletti, Moreno, Del Roveri and Zanardo2010, 2018; Del Roveri et al., Reference Del Roveri, Cunha, Zanardo, Godoy, Moreno, Rocha and Maestrelli2016; Montibeller et al., Reference Montibeller, Beltran, Zanardo, Ronh, Del Roveri, Rocha and Conceição2020; Motta et al., 2010; Zanardo et al., Reference Zanardo, Montibeller, Navarro, Moreno, Rocha, Del Roveri and Azzi2016.). Preliminary results from one of those studies were presented by Christofoletti et al. (Reference Christofoletti, Batezelli and Moreno2015). Studies of the economic use of these deposits in the area are scarce; only Souza Campos et al. (Reference Souza, Christofoletti, Moreno and Corrêa2010) and Meneghel (Reference Meneghel2021) have otherwise studied these clays, seeking improvement and diversification of products in the Santa Gertrudes Ceramic Cluster.
Although the deposits of these rocks are large in the State of São Paulo, they show significant variations in their facies throughout the occurrence (Sousa, Reference Sousa1985); these variations are important in determining whether or not the materials can be used as raw materials for ceramics. In the region of the Santa Gertrudes Ceramic Cluster, the deposits have become increasingly scarce due to inefficient mining, urban expansion, increased environmental restrictions, and competition from other forms of land use, especially the expansion and strengthening of agricultural activity in sugar cane crops (Christofoletti & Moreno, Reference Christofoletti and Moreno2011).
In order to guarantee the economic and environmental sustainability of the Santa Gertrudes Ceramic cluster, the present study sought to discover and evaluate new deposits in the Corumbataí Formation. The facies identification helped in the preliminary interpretation of the depositional and diagenetic environments of formation of those deposits.
Study Area and Geological Setting
The area that was studied is in the northeast of the state of São Paulo, around the cities of Porto Ferreira, Tambaú, and Santa Rosa de Viterbo (Fig. 1). Porto Ferreira is known as the capital of artistic ceramics. The raw materials for manufacturing those products come from other regions, however. Tambaú represents one of the state ceramic clusters that produce structural ceramics, where material from the upper layers of the Corumbataí Formation are used in the production of tiles and bricks, and in the composition of masse for various ceramic coating companies by the wet ceramic grinding process. Santa Rosa do Viterbo contains only one clay mine of the Corumbataí Formation, where the raw material is marketed to various ceramic industries to form a proportion of processed formulations via wet grinding.

Fig. 1 Geological map of the study area. Below is the South American Continent, including Brazil and the Paraná Sedimentary Basins. A simplified stratigraphic column is given on the right (modified from Perinotto & Zaine, Reference Perinotto and Zaine2008)
Geologically, the area that has been studied is in the Paraná Basin, which is a geotectonic entity located in the southeast part of the South American plate, covering an area of 1,600,000 km2. Most of that area is in Brazilian territory (~1,000,000 km2), distributed in the states of Rio Grande do Sul, Santa Catarina, Paraná, São Paulo, Mato Grosso do Sul, Mato Grosso, Minas Gerais, and Goiás (Milani, 1997). In the studied region, the following lithostratigraphic units occur from the bottom to the top, Itararé Subgroup, Passa Dois Group (Irati and Corumbataí Formations), São Bento Group (Pirambóia and Botucatu Formations), basic intrusive rocks of Serra Geral Formation, and culminating in Quaternary sediments and recent Cenozoic coverings (Fig. 1).
According Mezzalira (Reference Mezzalira1964), the term Corumbataí was first described initially by the Geographical and Geological Survey of São Paulo in a 1916 report as “clay and bituminous schist with fossils.” The Corumbataí Formation was referred to (Landim, Reference Landim1970) as “the essentially clayey sedimentary rocks of reddish or purplish color with interleaves of fine sandstone lenses in the Corumbataí River Valley.” Several authors have described this unit from various perspectives, e.g. Sousa (Reference Sousa1985), who studied the Corumbataí Formation and Estrada Nova facies in the state of São Paulo. It is Upper Permian in age and, according to its features, was formed in a platform environment with tidal influence, with input from the shallower-facies (lower shoreface) and deeper-facies associations (upper shoreface).
Materials and Methods
The research began with the elaboration of base maps for fieldwork purposes; these focused on the recognition of the lithofacies of the Corumbataí Formation in the area studied. The lithofacies identification was based on the methodology developed by Miall (Reference Miall1994). Detailed geological descriptions were given for 16 vertical profiles by means of data collection from mines in the area. Samples were collected and photographed in the field and then were sent to the laboratory for analyses as described below.
Granulometric analysis of ground samples was done by laser diffraction (Malvern Instruments Ltd, Malvern, Worcestershire, England), in which a sample aliquot, with particle size of <0.42 mm, was placed in a water solution and three drops of sodium hexametaphosphate (10%) added, obtained from the American House for Laboratory Articles (São Paulo, Brazil). The main methods of granulometric analysis in the control of ceramic raw materials were described by Orts et al. (Reference Orts, Campos, Pico and Gozalbo1993). X-ray diffractometry was used to identify the mineral phases (Brindley & Brown, Reference Brindley, Brown, Brindley and Brown1980; Thiry, Reference Thiry1974). The clay fraction was arranged on glass sheets (using preferred orientation) and analyzed after undergoing natural drying, solvation with ethylene glycol for 48 h, and heating in a muffle furnace at 500°C for 2 h. Mineralogical analyses were performed using a Panalytical X-ray diffractometer (Almelo, Netherlands). Measurements were made using CuKα1 radiation (λ = 1.54056 Å) and a Ni filter. Interpretation of XRD traces and the semi-quantitative abundance of minerals were based on peak height using the Panalytical software X'Pert HighScore Plus.
For major elements s, a fraction of each of the samples was ground (to a diameter of ≤0.42 mm). The major elements were analyzed using inductively coupled plasma-atomic emission spectrometry (ICP-AES) by Labexchange (Baden-Württemberg, Germany). Loss on ignition (LOI) was measured by weight difference before and after combustion at 1000°C. The sample-preparation method used was fusion with lithium metaborate obtained from PGM Vectra (São Paulo, Brazil) (Penanes et al., Reference Penanes, Reguera-Galan, Huelga-Suarez, Rodríguez-Castrillón, Moldovan and Alonso2022).
Following mineralogical, chemical, and granulometric analyses, ceramic characterization of the samples by the dry process was carried out under laboratory conditions. Initially, complete samples were crushed, divided into four, ground in a hammermill, moistened, pressed, and heated in a lab oven at 1050°C. This temperature was chosen for laboratory testing because it is close to the temperatures used commercially. After those procedures, tests were carried out for water absorption, apparent porosity, apparent density, linear firing and drying shrinkage, flexural strength module, and loss on ignition. All measurements followed the ABNT NBR–13818/1997 standards, and the results were sorted according to the water absorption group (Table 1).
Table 1 Classification of ceramic tiles according to ABNT (1997) applied to pieces 2 cm × 7 cm in size

Results
Geology and Lithofacies
The Corumbataí Formation crops out broadly in the area that has been studied (Fig. 1). In Porto Ferreira, this unit occurs consistently throughout the city. The upper contact has mostly undifferentiated Cenozoic cover, and the bottom contact has tabular intrusions. In Tambaú, this unit presents the upper contact with the Botucatu Formation, and the lower contact with the Itararé Subgroup. This unit offers less exposure in Santa Rosa do Viterbo, and its upper contact has undifferentiated Cenozoic cover; its bottom contacts are tabular intrusions.
According to the facies survey, the lithofacies were grouped into lower shoreface and upper shoreface associations. The geological profiles studied, the lithofacies identified, and the sample locations are shown in Fig. 2.

Fig. 2 Studied profiles in the study area. Thickness (m), granulometry, facies (associated colors and sedimentary structures present) and samples collected
Lower shoreface association. This facies association contains massive lithofacies at the bottom and laminated siltstone lithofacies on the top. (Fig. 3).

Fig. 3 Details of Lower Shoreface Association lithofacies: a,b massive siltstone lithofacies; c laminated lithofacies; and d contact between massive and laminated lithofacies
Massive siltstone (Sm): showing massive structure and gray, red, and purple coloration, forming beds with thicknesses ranging from 70 cm to ~5 m. The boundary between them is given by thin layers (up to 5 cm) of very-fine sandstone composed of quartz grains or by Bone beds, consisting of fragments of up to 6 mm of teeth and scales of Palaeonisciformes and bivalve shells of subclasses Anomalodesmata, Pteriomorphia, Heterodonta, and Palaeoheterodonta (Salvador & Simone, Reference Salvador and Simone2010).
Laminated siltstone (Sl): showing gray, red, and purple coloration, represented by massive siltstone showing millimetric laminations of whitish, very fine sand. Iron and manganese nodules can also be found.
Upper shoreface association: consisting from bottom to top of sandy-silty lithofacies, including heterolithic sandstone, lenticular sandstone, intercalated silty, and sandy. This association is dominated by an increase in the sand toward the tops of sections.
Heterolithic sandstone (Sh): is characterized by fine, silty sandstone intercalations, with green pelitic blades, forming mud couplets. The sandstone presents well-sorted grains; rounded with reddish beige coloring, and strata with thicknesses ranging from 1 to 15 cm. They present planar-parallel stratification and wavy marks with drapes of clay. Concentrations of manganese nodules up to 2 cm in diameter are common, as well as shafts of silicon carbonate composition discordant with bedding (Fig. 4).

Fig. 4 a Heterolithic sandstone lithofacies, outcrop exposing (at the bottom) reddish-brown pelite overlapped by thin sandstone intercalations, well-sorted grains with greenish gray claystones (cut and fill). At the top is fine sandstone with planar-parallel, wavy, and clay drapes. b Close-up of the conjugated pairs of clay (mud couplets). c Close-up of manganese dioxide concentration and d P11 profile
Lenticular sandstone (Sle): consists of reddish-brown pelitic deposits with lenses from very fine to fine sandstone, well-sorted grains, 1.5 m long, with a thickness of up to 15 cm, and lenticular cross-stratification. In addition, it has irregular horizontal tubes, 1 cm in diameter, filled with very fine sandstone, which can be found at some sites. Sandstones can often form layers tens of meters long and up to 30 cm thick. It also has sole marks on the bottom and steep top (Fig. 5). Commonly, these lithofacies are repeated above the sandy facies at the tops of the units.
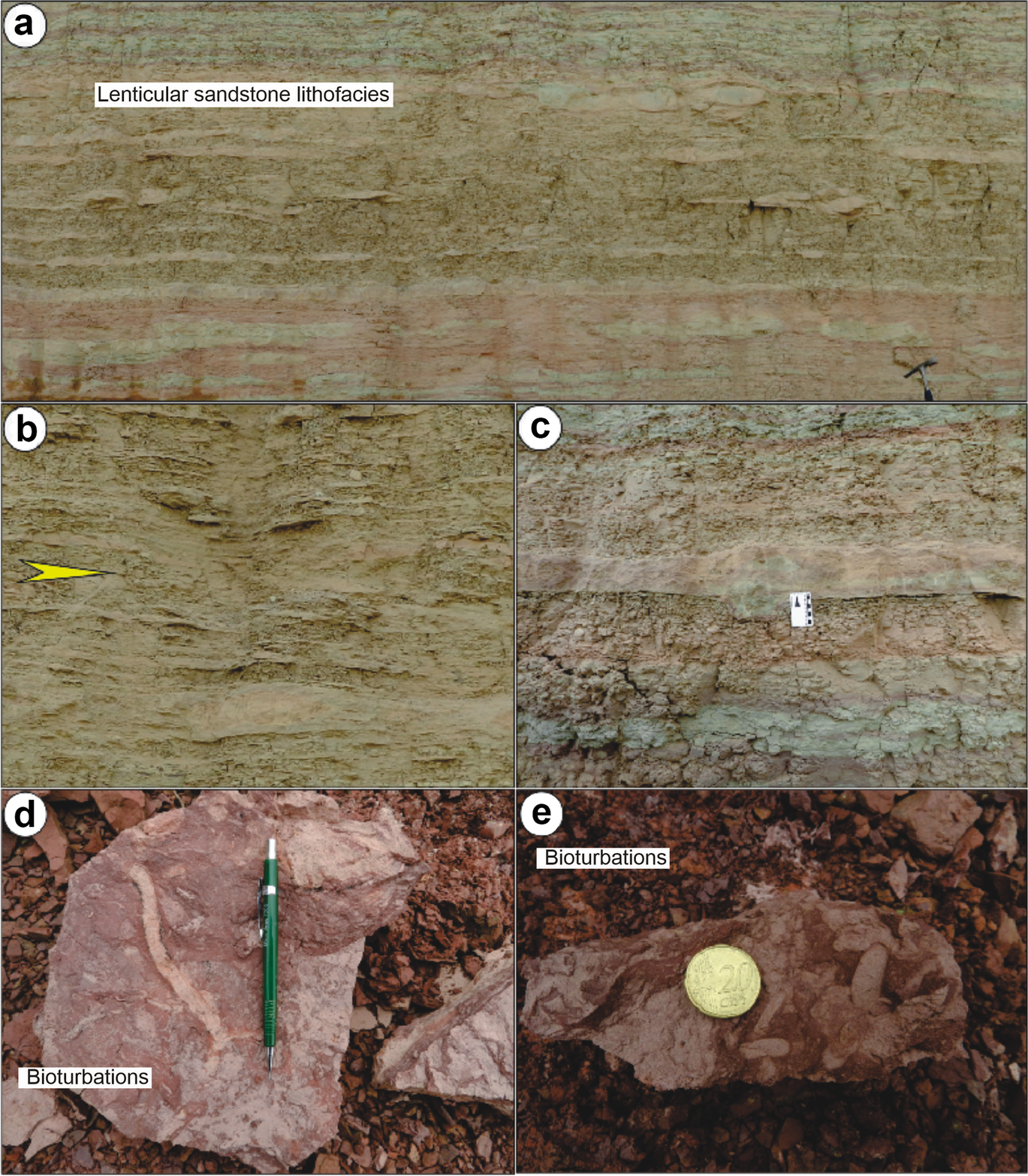
Fig. 5 Lenticular sandstone lithofacies. a Mudstones and sandstones with cross lenticular stratification; b Close-up of lenticular sandstone (yellow arrow indicates the direction of the paleochannel); c Sandstone layers with sole marks at the bottom and steep top. d,e Bioturbations filled with very fine sandstone
Intercalated sandstone/siltstone (Si): this lithofacies occurs either with sand prevailing over the clay (sandy intercalated lithofacies) or clay over the sand (silty intercalated lithofacies). Sandstone and siltstone layers feature varying centimeter thicknesses and can be referred to as ‘rhythmite’ (Fig. 6a,b).

Fig. 6 a,b Intercalated siltstone lithofacies; c intercalated sandstone lithofacies; and d altered siltstone lithofacies
Altered siltstone (Sa): altered without preserved sedimentary structures, it has white, light red, and yellowish colors, the superficial part of the profiles.
Granulometry
The granulometric distribution of dry-ground samples was analyzed by laser diffraction (Fig. 7). Multimodal behavior was observed in diagrams at various granulometric tracks. The largest concentrations of particles are in the intervals 1–10 µm and 10–100 µm, with mean values ranging from 48.23 to 73.03% and from 20.70 to 38.26%, respectively (Table 2, Fig. 7), classified, according to Wentworth (Reference Wentworth1922), as thick siltstone to very fine sandstone. Smaller percentages of grains are distributed in intervals which are 0.1–1 µm and 100–1000 µm in most of the studied sections; but when the distribution is less well sorted and there are grain concentrations in these intervals, there is an improvement in the ceramic properties, as can be seen in the samples of profiles P7 and P15. This happens because of the packing of particles during the pressing process which favors sintering and thus improves the ceramic properties.

Fig. 7 Laser granulometric distribution of the P3, P4, P7, P11, P15, and P16 profiles
Table 2 Granulometric distribution by laser diffraction (%)
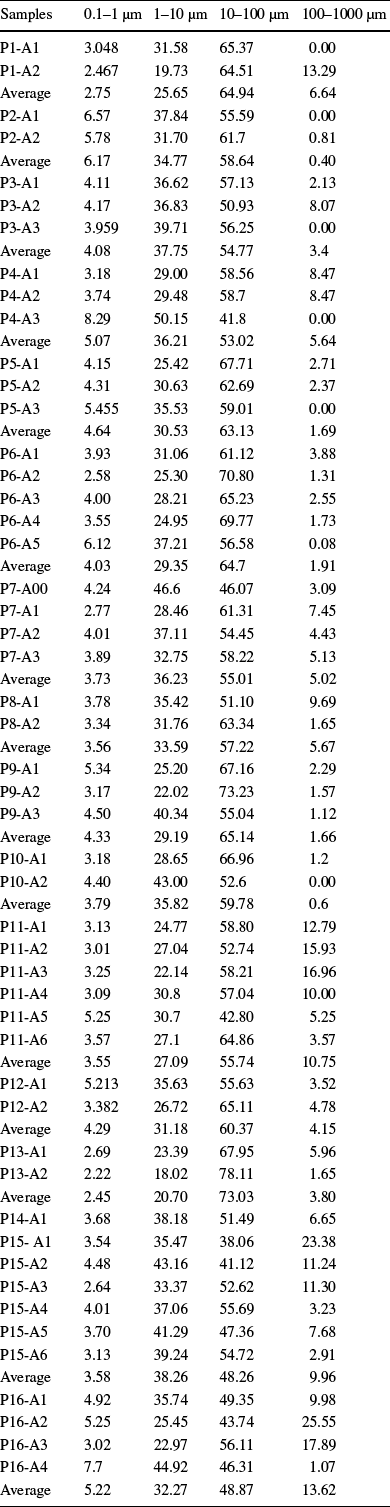
Mineralogy
The mineralogical composition is illustrated in Table 3 and Fig. 8. Among the minerals present were as follows. (1) A group of silicates; quartz occurred in all XRD traces of the whole rocks, with its principal peak at d 001 = 3.33 Å; two types of feldspar – microcline and albite – microcline was more abundant, with d 001 = 3.24 Å. (2) Non-silicate minerals; calcite and dolomite, were identified in the whole-rock sample; calcite was found in most of the profiles studied and showed a its main peak at d 001 = 3.00 Å. In the P5, P7, P11, and P16 profiles (Fig. 8) associated with calcite, the dolomite mineral occurs with greater intensity at d 001 = 2.88 Å. In the oxides – where hematite was prominent in samples of the P4 profile, with a notable peak at d 001 = 2.69 Å, magnetite also occurred, but less frequently, with the main peak at d 001 = 2.53 Å. (3) A group of clay minerals, where illite, kaolinite, and montmorillonite were prevalent, their structure (phyllosilicates) allowed preferred orientation in the fine fraction, revealing prominently the peaks for d 001, d 002, and d 003. The most abundant clay mineral in the Corumbataí Formation is illite, found in all studied profiles, with interlayer spacing (d 001) of 10 Å in natural conditions. This did not change when subjected to heating (500°C) and treatment with ethylene glycol, maintaining a peak of 10 Å. Peaks representing d 002 at 4.98 Å and d 003 at 3.32 Å were also noted.
Table 3 Relative quantification (by XRD) of minerals present. Obs. 15 Å (montmorillonite), 10 Å (illite), 7 Å (kaolinite), 4.26 Å (quartz), 3.24 Å (microcline), 3.19 Å (albite), 3.00 Å (calcite), 2.88 Å (dolomite), and 2.69 Å (hematite)

Frequency: * (very low), ** (low), *** (medium), **** (high)
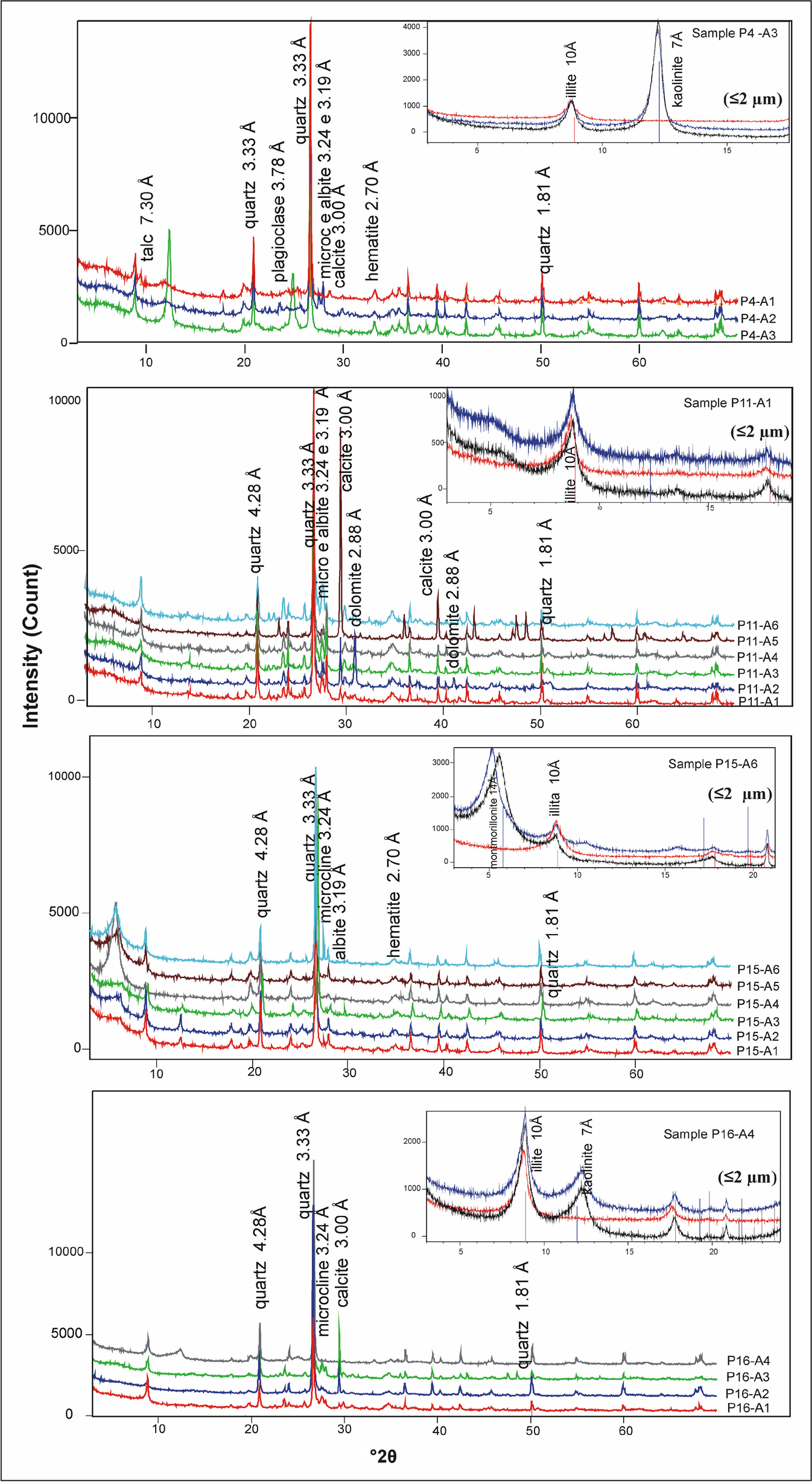
Fig. 8 XRD of whole-rock samples with clay fractions inset
Kaolinite and montmorillonite exchange places as the second most abundant mineral in the profiles studied. Kaolinite occurred as the most abundant clay mineral in samples P1-A2 and P4-A3, predominantly in the upper strata. It has a d 001 spacing of 7 Å in the natural condition, and when subjected to heat (500°C), the peak vanished. The d 002 is at 3.58 Å, and the d 003 at 4.36 Å. On the other hand, the clay mineral of the smectite group identified was montmorillonite, present with greater prominence in samples of the P3 and P15 profiles (Table 3, Fig. 8), showing d 001 = 14.8 Å, and when subjected to treatment with ethylene glycol, its value shifted to 17 Å.
Chemical
The major elements percentages and respective averages are listed in Table 4. SiO2 represents the most prevalent oxide in the studied sections, with average values of 65.01%, and MnO is the least abundant, with 0.05%. According to the oxides diagrams, shown in Fig. 9 (Rollinson, Reference Rollinson1995), we have observed that when with increasing SiO2, Al2O3 tends to decrease. It occurs due to the loss of SiO2 in the upper layers by the alteration process of the feldspar and the clay minerals illite and montmorillonite. On the other hand, Fe2O3, TiO2, and LOI increased when Al2O3 increased. Alkali oxides (K2O, Na2O) and alkaline earth metals (MgO and CaO) varied irregularly with increasing Al2O3. The average values of SiO2 were between 60 and 70% (Fig. 9, Table 4), and the greatest concentration was found in sample P16-A2, with 77.57%, and the lowest, 49.36%, found in sample P11-A5. The overall average amount of Al2O3 was 14.29%, with greater average values in the P4 profile of 18.60%; the lowest values of 10.63% were in the P11 profile (Table 4). Al2O3 and SiO2 are considered to be refractory elements.
Table 4 Chemical composition, obtained by ICP-MS, of the major elements (%)
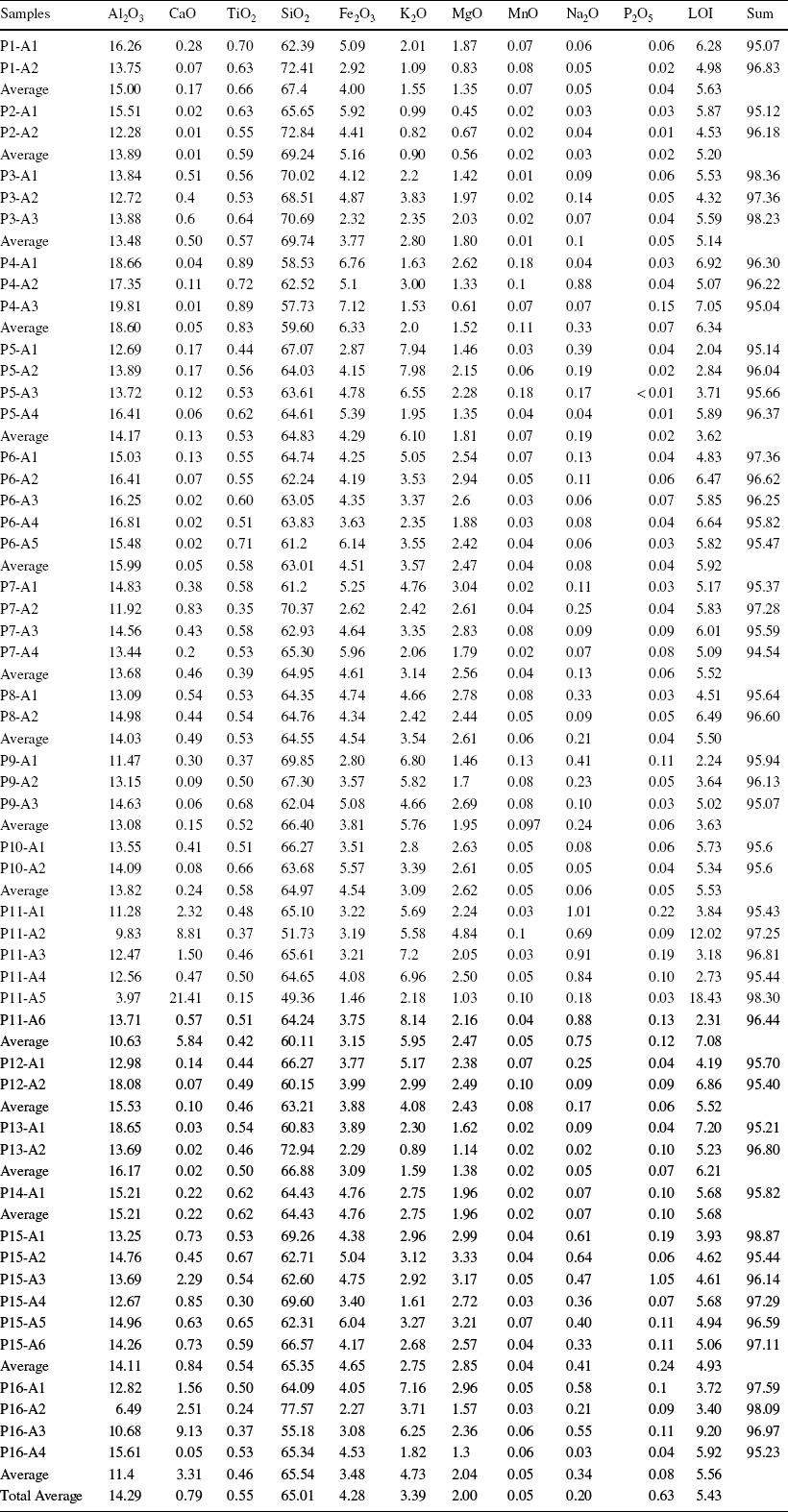

Fig. 9 Harker variation diagram, with major elements as a function of Al2O3
K (in illite) and Na (in albite) are responsible for sintering. Their average contents, expressed in oxide form, were 0.20% (Na2O) and 3.39% (K2O). These elements and MgO (2.00%) did not correlate well with increasing Al2O3, revealed by the scattered points (Fig. 9); this was probably the result of mixed sources (minerals) and distinct behavior; another major source of K2O is the mineral feldspar. Both feldspar and illite tend to evolve into kaolinite; on the other hand, Mg is found in minerals such as illite, chlorite, and carbonates, as the main element or substituted for other elements with similar ionic behaviors. For Na, most samples have small concentrations; it is found in albite and montmorillonite and as a substitute for other elements, such as mineral crystals of Ca and K (Rollinson, Reference Rollinson1995).
The largest relative amount of CaO was found in the P11 profile, with 5.84% on average, and the smallest in the P4 profile, with a value of 0.05%. This element stood out in samples P11-A2, P11-A5, and P16-A3, with 8.81, 21.41, and 9.13%, respectively. Greater amounts of MgO were found in samples of the P15 profile, with values of 2.85% and smaller values of 0.56% in the P2 profile. More Na2O (0.41%) was present in the P15 profile and less (0.05%) in the P13 profile; this is a very small amount compared to the levels found in the Corumbataí Formation in the Santa Gertrudes ceramic region. K2O made up 6.10% in the P15 profile and 0.90% in P10 profile. Profiles that showed greater percentages of MgO were P11 and P15, and the value in sample P11-A2 was 4.84%.
The sum of CaO + Na2O (Fig. 10c) was 0–3% in all samples except P11-A5, which had a value of 21.59%. A reverse trend was observed between SiO2 concentration and the sum of Al2O3 + TiO2 + Fe2O3 (Fig. 10a). The largest differences occurred in samples P11, P11-A2, and P11-A5 due to the small amount of SiO2 and the high percentages of CaO confirmed in the XRD patterns (Fig. 8). In the P16 profile, sample P16-A2 contained the most SiO2 (77.57%), also confirmed by the highest quartz peak in XRD (Fig. 8). The other elements, P2O5, MnO, and TiO2, were present in smaller amounts.
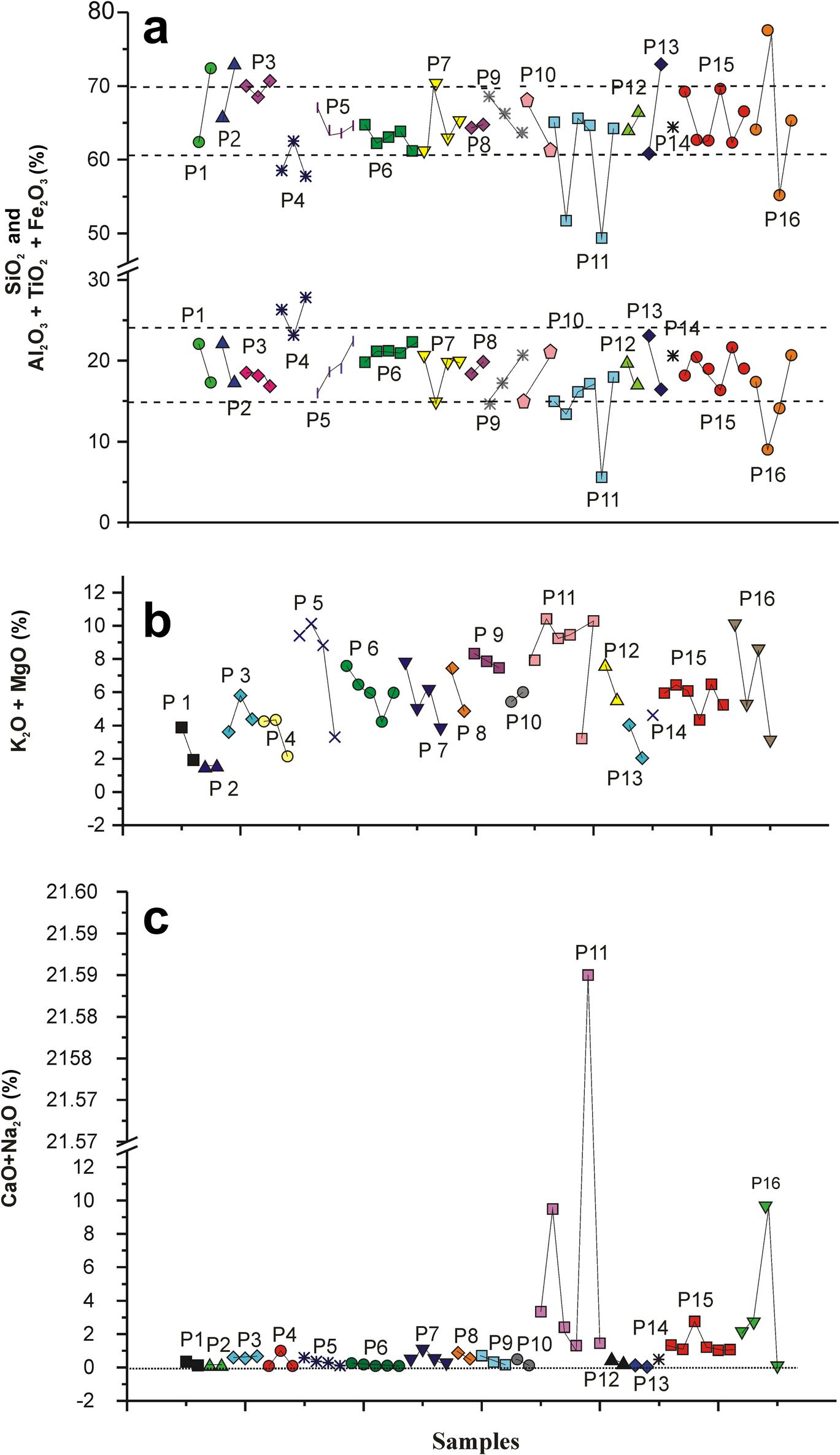
Fig. 10 Variation in concentrations of oxides in the samples of each sampled columnar profile
Ceramic Properties
To identify the ceramic properties, tests were performed on samples of the studied sections (Table 5). The use of clayey raw materials in tile manufacturing depends on the technological and appearance requirements of the ceramic body, such as color after firing and behavior of the masse during the tile-making process; both properties are related intimately to mineral composition, chemical composition, and particle-size distribution (Dondi, Reference Dondi1999).
Table 5 Ceramic characterization. MRF = modulus of rupture flexion after firing (kgf/cm2). WA = water absorption (%), AP = apparent porosity (%), LFS = linear firing shrinkage, Ddry = apparent drying density (g/cm3), and GWAs = groups of water absorption
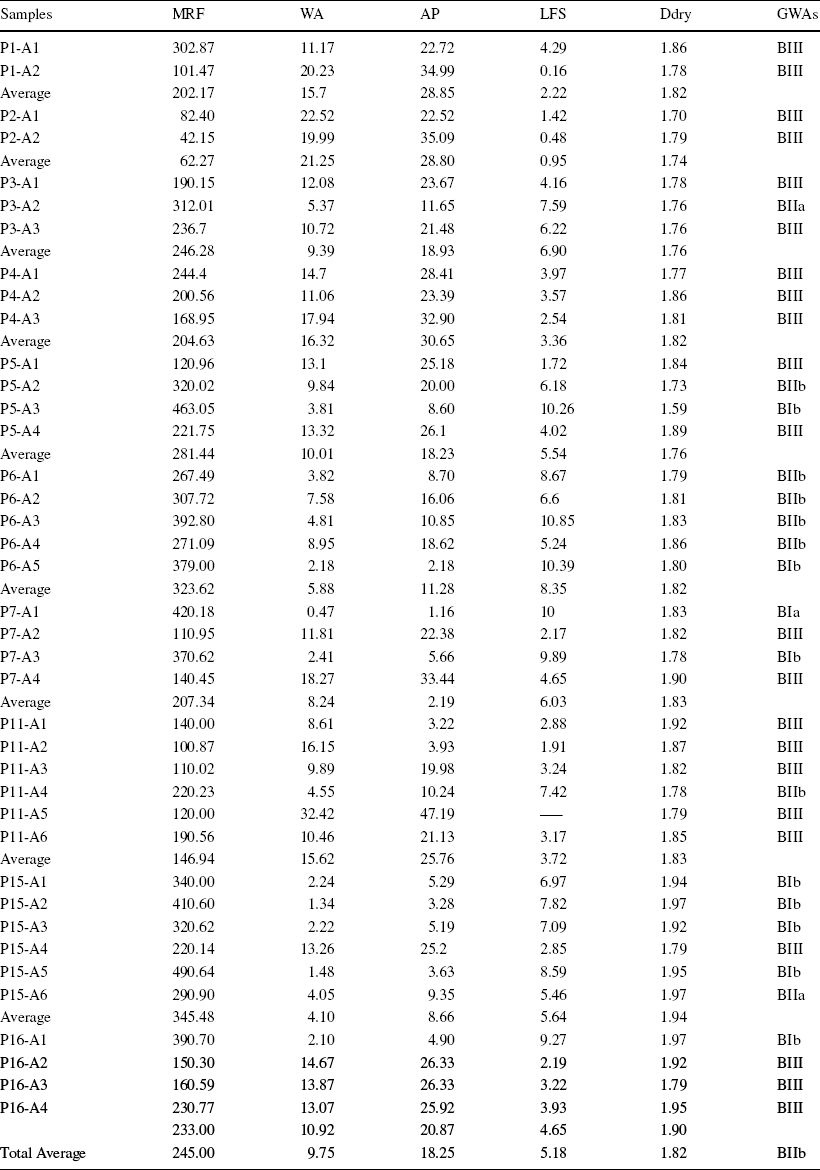
Of sixteen profiles, ten representative profiles were selected for ceramic testing, having been evaluated for the following features: flexural strength, water absorption, apparent porosity, apparent dry density, shrinkage upon linear firing, and granulometric distribution by laser diffraction. Thirty-nine samples were analyzed and classified according to their water absorption group (Table 5): 22 (56.4%) in Group BIII (porous); six (15.4%) within Group BIIb (semi-porous); two (5.12%) in BIIa (semi-stoneware); eight (20.5%) in Group BIb (stoneware) and one (2.6%) in Group BIa (porcelain). The overall average water-absorption percentage for all samples was 9.75%, which falls within the Group BIIb classification, and the average flexural strength was 245 kgf/cm2. Samples from the P1, P2, P3, P4, P11, and P16 profiles are classified in Group BIII and the P6 profile in Group BIIb. The P6 and P15 profiles gave the best ceramic results, with average flexural rupture modulus (MRF) values of 323.52 kgf/cm2 and 345.48 kgf/cm2 and water absorption (WA) of 5.88% and 4.10%.
Discussion of Results
Based on the results obtained, the Corumbataí Formation, other than samples P6, P7 and P15, shows similar geological, mineralogical, chemical, and ceramic characteristics to the profiles studied. The facies study allowed the lithofacies identified to be grouped into two facies associations: Lower Shoreface Association and Upper Shoreface Association. In the Lower Shoreface Association, the lithofacies found were massive siltstone (Sm) and laminated siltstone (Sl). According to Sousa (Reference Sousa1985), the characteristics indicated sedimentation by settling in a marine-platform environment, below the level of waves and low-energy waves. In the Upper Shoreface Association, the lithology consisted, from bottom to top, of heterolithic sandstone (Sh), lenticular sandstone (Sle), and intercalated siltstone/sandstone (Si) lithofacies. In this association, an increase in sand and energy prevailed towards the top of the profiles, reflecting directly the mineral and chemical composition and the ceramic properties found.
According to mineralogical identification by XRD, the main minerals present belong to the following mineral groups: tectosilicates, carbonates, oxides, and phyllosilicates. The tectosilicate is the most abundant mineral group in rocks of the Corumbataí Formation and is represented mainly by quartz and feldspars (microcline and albite). The dominant feldspar type in the studied samples differed from that found in the Santa Gertrudes Ceramic cluster region, however. In the studied area, microcline is predominant, while in the Ceramic cluster region, albite is more frequent (Christofoletti et al., Reference Christofoletti, Moreno and Batezelli2006).
Thus, potassium is the element that could contribute most to the formation of a liquid phase during firing as it is found in illite which releases this element at low temperatures; potassium feldspar remains in the firing processes virtually unchanged. The carbonates (calcite and dolomite) and oxides (represented by hematite) are lessfrequent. The calcite and dolomite increase the porosity and decrease the mechanical resistance, as can be observed in samples P11-A2, P11-A5, and P16-A3.
Regarding phyllosilicates, illite is predominant, followed by kaolinite and montmorillonite. Illite occurs in the basal and intermediate portions with kaolinite and smectite in the upper portions, sometimes in the form of alterites. The geochemical results obtained by quantifying the major elements confirm the mineralogy found. SiO2 is the most prevalent element and is found in the form of quartz, feldspar, and phyllosilicates. K2O is in the composition of the microcline feldspar and the composition of illite.
According to the ceramic results, almost all studied samples were classified within the BIII group of water absorption as ‘porous,’ with water absorption values of between 10 and 20% and modulus of rupture at between 150 and 200 kgf/cm2. This is due to low concentrations of Na2O, the significant amount of microcline, and coarser granulometry identified in fieldwork and confirmed by laser diffraction granulometry. These allied factors resulted in a greater porosity of the fired pieces, resulting in poor packaging during pressing, limiting the reactivity between the grains.
In profiles P6, P7, and P15, the ceramic results differ from the others that have been studied, with most samples classified within the BIIb and BIb Groups (semi-porous and stoneware). The ceramic properties of the P15 profile were similar to those obtained from the raw materials found in the Santa Gertrudes Ceramic Cluster, such as the red color, finer granulometry, and a better distribution of grains, favored by the greater concentration of clay minerals. This may be related to the proximity of a heat source – the basic intrusions resulting from the action of Serra Geral basaltic magmatism of Cretaceous age (Costa, Reference Costa2006).
Conclusions
The major outcomes of this study can be summarized as follows:
(1) the results obtained here expand the scientific knowledge of the Corumbataí Formation from the geological, mineralogical, chemical, and ceramic properties points of view. This information is critical to a better understanding of the same rocks in other locations as source materials, and is fundamental to the sustainability of the ceramic-coating industry in the State of São Paulo.
(2) The ceramic behavioral trends of the six facies identified and studied are similar to those in the Corumbataí Formation in the Santa Gertrudes Ceramic Cluster where the vertical succession or stacking comprises an ascending grain growth, with a predominance of finer/clayey pelitic facies at the base (massive and laminated), evolving to predominantly coarser/sandier lithofacies interspersed with pelitic facies on top (heterolithic, lenticular, and intercalated). These facies indicate sedimentary processes typical of a shelf marine environment, below the average level of normal waves and dominated by tidal flows. The increase in the sand content of the facies toward the top indicates the transition from a deep-shelf marine environment, dominated by decantation and traction processes associated with low-energy tides, going toward shallower domains, with combined flows.
(3) A lateral regional variation of these facies exists throughout their exposure in the State of São Paulo, resulting from the genetic formation of the deposits and the location of their source area. This is reflected, in particular, in the facies description, as well as in terms of the mineralogy and chemical composition, where the presence of a specific mineral or element is reflected in the ceramic properties and the type of product manufactured, as well as in the economic use as a ceramic raw material.
(4) The deposits studied differed from those found in the Santa Gertrudes Ceramic Cluster, where the granulometric, chemical, and mineralogical characteristics indicate a more refractory and less flux ceramic behavior.
(5) The water absorption results obtained under laboratory conditions revealed that most of the samples fall into the BIII Group, referred to as ‘porous’ according to ABNT; their application will focus mainly on the production of tiles for internal walls, used especially in bathrooms. Exceptions were found in samples of facies of profiles P6, P7, and P15 which have a more uniform granulometric distribution, resulting in better sintering than the clays found in the region of the Santa Gertrudes Ceramic cluster. This classifies these samples into Groups BIIb and BIb, named according to their commercial nomenclature as semi-porous and stoneware; their application is mainly in the production of tiles for floors or in the composition of ceramic masses for the production of porcelain by wet grinding. Note that ceramic tests were carried out in laboratory conditions and that industrial-scale tests are needed to verify the results.
Acknowledgements
The authors gratefully acknowledge the financial support of FAPESP (São Paulo Research Foundation) Process 2012/24219-9. The second author thanks CNPq for the productivity support (Process 310734/2020-7).
Declarations
Conflict of Interest
The authors declare that they have no conflict of interest