Introduction
Noah Parker discovered trilobites while quarrying slate near Saint Albans in northwestern Vermont in 1855 (Marcou, Reference Marcou1888). These remarkable fossils, first reported by James Hall (Reference Hall1859), are now known as Mesonacis vermontanus, Olenellus thompsoni, and Bathynotus holopygus. The trilobites from the locality have continued to merit attention (Whittington, Reference Whittington1989; Lieberman, Reference Lieberman1999; Webster, Reference Webster2009; Webster and Landing, Reference Webster and Landing2016). Walcott's (Reference Walcott1884) description of the non-biomineralized bivalved arthropod Protocaris marshi established the Parker Quarry as the first locality to yield a Burgess Shale-type soft-bodied fossil (Landing, Reference Landing2007; Pari et al., Reference Pari, Briggs and Gaines2021). Shaw (Reference Shaw1954, p. 1041) reported that “the Noah Parker Quarry has been entirely quarried away,” giving rise to the assumption that there was no longer scope for collecting soft-bodied fossils there (Briggs, Reference Briggs1976; Rigby, Reference Rigby1987; Conway Morris, Reference Conway Morris1993; Landing, Reference Landing2007; Webster, Reference Webster2009; Webster and Landing, Reference Webster and Landing2016). The productive layers were rediscovered by GP in 2001 and have since yielded several new specimens of the trilobites and new soft-bodied fossils, including taxa previously unknown from the site (Pari et al., Reference Pari, Briggs and Gaines2021) (Figs. 1–10).

Figure 1. EDS elemental maps of ?Ottoia (YPM IP 239053) and Vermontcaris montcalmi n. gen. n. sp. (YPM IP 543321). (1) Photograph of YPM IP 239053 and YPM IP 543321, showing the areas mapped. (2, 3) Elemental maps showing the distribution of C, O, Al, Si, Fe, and S in areas (2) (YPM IP 239053) and (3) (YPM IP 543321).

Figure 2. Fuxianospira gyrata Chen and Zhou, Reference Chen and Zhou1997. (1) YPM IP 239095. (2) YPM IP 239097.

Figure 3. (1–7) Leptomitus zitteli Walcott, Reference Walcott1886. (1–3) USNM 15308, lectotype, entire specimen, plumose spicules evident at distal termination, long vertical and short horizontal oxeas. (4) YPM IP 543326, isolated bunch of oxeas. (5–7) YPM IP 239151, distal end showing long vertical oxeas, explanatory drawing of entire specimen (dw = detached wall), and entire specimen showing apparent branching. (8, 9) ?Protospongia hicksi Hinde, Reference Hinde1887, isolated cruciform spicules associated with USNM 15308, and associated with USNM 419731.

Figure 4. ?Ottoia Walcott, Reference Walcott1911 (Walcott, Reference Walcott1911b), (1, 2) YPM IP 239053, specimen and explanatory drawing. an = trunk annulations; g = gut trace; p = proboscis.
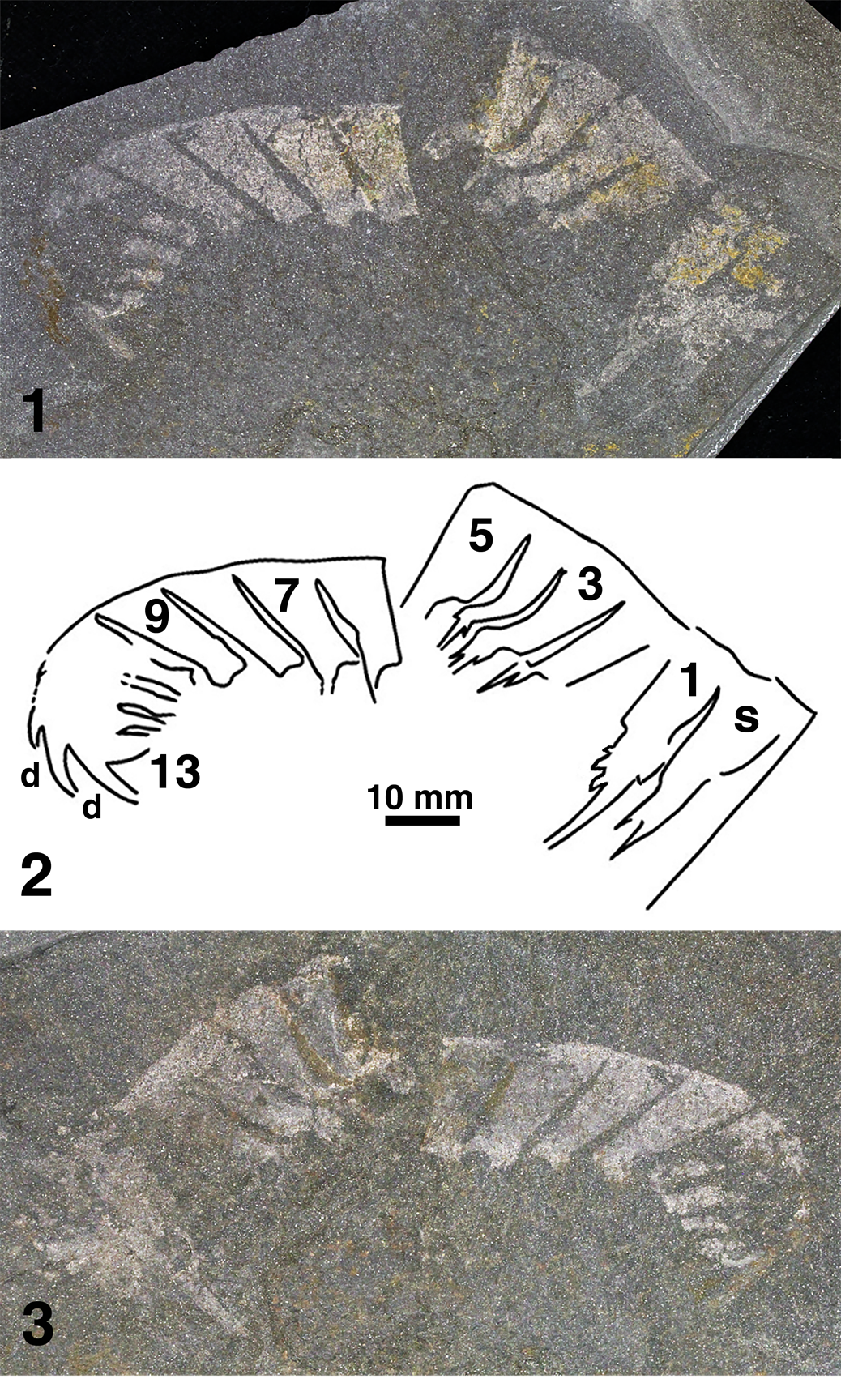
Figure 5. Radiodont appendage. (1–3) YPM IP 239052, part, explanatory drawing, and counterpart. d = dorsal spine; s = distalmost podomere of shaft; 1, 3, 5, etc. = podomeres of articulated region.

Figure 6. (1–10) Tuzoia polleni Resser, Reference Resser1929. (1, 2) USNM 26728, specimen and explanatory drawing; (3, 4) YPM IP 239051, explanatory drawing and specimen; (5, 6) YPM IP 239150, specimen and explanatory drawing; (7, 8) YPM IP 239149, specimen and explanatory drawing; (9, 10) YPM IP 543331, specimen and explanatory drawing. (11) Protocaris marshi Walcott, Reference Walcott1884, USNM 15400, holotype.

Figure 7. Vermontcaris montcalmi n. gen. n. sp. (1, 2) USNM 15314D, specimen and explanatory drawing. (3, 4) USNM 15314E, specimen and explanatory drawing. (5, 6) USNM 15314F, specimen and explanatory drawing. ap = thoracic appendages; e = eye; g = gut trace.

Figure 8. Vermontcaris montcalmi n. gen. n. sp. (1, 2) YPM IP 543320, holotype, specimen and explanatory drawing. (3, 4) YPM IP 543316, specimen and explanatory drawing. (5, 6) YPM IP 543333, specimen and explanatory drawing. (7) YPM IP 239153. (8–10) YPM IP 543334, specimen dry, explanatory drawing, and specimen wet. a = anus; ab = abdomen; ap = thoracic appendages; ba = serially repeated structures indicating limb bases; e = eye; g = gut trace; m = paired relief structures indicating mouth appendages; t = telson; ta = leaf like appendage associated with telson.

Figure 9. Vermontcaris montcalmi n. gen. n. sp. (1, 2) YPM IP 543335, specimen and explanatory drawing; (3, 4) YPM IP 543312, explanatory drawing and specimen; (5, 6) YPM IP 239148, specimen and explanatory drawing; (7, 8) YPM IP 543319, explanatory drawing and specimen; (9, 10) YPM IP 543321, specimen and explanatory drawing. a = anus; ab = abdomen; ap = thoracic appendages; ba = serially repeated structures indicating limb bases; e = eye; g = gut trace; m = paired relief structures indicating mouth appendages; t = telson; ta = leaf like appendage associated with telson; th = exposed thorax.

Figure 10. (1, 2) ?Herpetogaster Caron, Conway Morris, and Shu, Reference Caron, Conway Morris and Shu2010, YPM IP 239054, specimen and explanatory drawing. (3–5) Emmonsaspis cambrensis (Walcott, Reference Walcott1890). (3, 4) YPM IP 239155 and YPM IP 239156, two specimens in association with Vermontcaris montcalmi n. gen. n. sp. (YPM IP 543319), which is distinguished by the trunk and gut trace (see Fig. 9.8), specimen and explanatory drawing with lines indicating the attitude of the somites; (5) YPM IP 543315. e = eye; li = liver; s = stomach; tn = tentacles; V = trunk of Vermontcaris montcalmi n. gen. n. sp., YPM IP 543319 (see Fig. 9.8); w = compaction wrinkles.
Walcott's (Reference Walcott1884) description and illustration of Protocaris marshi was appended to a report treating Cambrian faunas of New Brunswick and Massachusetts. The description was reprinted in Walcott's (Reference Walcott1886) account of the Cambrian faunas of North America, with some additional remarks on a possible relationship between Protocaris and the notostracan branchiopod Triops (then known as Apus). Walcott (Reference Walcott1886) listed and described fossils from the Parker Quarry, including illustrations (pl. 11, figs. 4, 4a) of two fossils, which he referred to Diplograptus ? simplex. Resser and Howell (Reference Resser and Howell1938) argued that these specimens are likely to represent a chordate and one of them (Walcott, Reference Walcott1886, pl. 11, fig. 4a) was subsequently identified as such (Conway Morris and Caron, Reference Conway Morris and Caron2014). The other (Walcott, Reference Walcott1886, pl. 11, fig. 4) is an example of Vermontcaris montcalmi n. gen. n. sp., a new bivalved arthropod named and described here. Walcott identified a third ‘soft-bodied’ specimen (Reference Walcott1886, pl. 11, fig. 5) as Climacograptus ? emmonsi, which Resser and Howell (Reference Resser and Howell1938) regarded as arthropodan and probably Anomalocaris. Briggs (Reference Briggs1978b, Reference Briggs1979) rejected this interpretation, and the affinities of this specimen remain uncertain. Walcott (Reference Walcott1886, pl. 2, figs. 2, 2a) also described and illustrated the sponge Leptomitus zitteli, which preserves soft tissue as dark carbonaceous material associated with the spicules. Finally, in his synopsis of the fauna of the lower Cambrian or Olenellus Zone, Walcott (Reference Walcott1890) reproduced his figure of Protocaris marshi (pl. 81, fig. 6) and those of Leptomitus zitteli (pl. 49, figs. 1, 1a). He refigured the two specimens he previously identified as Diplograptus ? simplex, referring them to Phyllograptus ?? with a new species name cambrensis (pl. 59, figs. 3, 3a) and remarking on “the imperfection of the material for study.” Walcott also illustrated a specimen of Tuzoia (pl. 59, fig. 1) without description, the first record of this bivalved arthropod, noting it as “a problematical organic marking.” Thus, the importance of the Parker Formation as a source of non-biomineralized fossils dates from 1884, and by 1890, 20 years before the first report of fossils from the more famous Burgess Shale (Walcott, Reference Walcott1911a), five soft-bodied or lightly sclerotized genera representing three different phyla had been discovered, even though only Protocaris and Leptomitus had been named and correctly assigned to a group.
New specimens collected from the Parker Quarry Lagerstätte (Pari et al., Reference Pari, Briggs and Gaines2021) include representatives of all these soft-bodied and lightly sclerotized taxa except Protocaris, as well as the alga Fuxianospora and single examples of a stem ecdysozoan similar to Ottoia, a radiodontan appendage, and a possible cambroernid comparable to Herpetogaster, taxa previously unknown from the site. These soft-bodied and lightly sclerotized taxa are the subject of this paper. Rare shelly taxa have also been found, including trilobites, an obolid brachiopod and a hyolith (Pari et al., Reference Pari, Briggs and Gaines2021). The Parker Formation biota is important among Burgess Shale-type faunas not only as the first reported, but also as one of the oldest (Dyeran Series 2, Stage 4) on the North American continent (Conway Morris, Reference Conway Morris1989; Hagadorn, Reference Hagadorn, Bottjer, Etter, Hagadorn and Tang2002; Pari et al., Reference Pari, Briggs and Gaines2021).
Locality and stratigraphic horizon
Fossils were collected from the Parker Quarry, Franklin County, Vermont, within the Parker Formation in the latter half of the 19th century (see Webster and Landing, Reference Webster and Landing2016). The most significant material was recovered by Walcott in 1883 and 1884 (Walcott, Reference Walcott1885; see Yochelson, Reference Yochelson1998). That original material was assigned to three US National Museum localities: 319g, 319m, and 25. Shaw (Reference Shaw1954, p. 1040) considered 319g and 319m, both described by Walcott (Reference Walcott1912b), to be “almost certainly the same,” a view endorsed by Webster and Landing (Reference Webster and Landing2016). Confusion about the location of USNM 25, and by inference the total thickness of the productive layers of the Parker Quarry (Shaw, Reference Shaw1954; Webster and Landing, Reference Webster and Landing2016), reflects an error in Walcott (Reference Walcott1912b) where USNM 25 is recorded in the ‘sandstone’ unit above the Parker Quarry rather than in ‘argillaceous shales’ (Walcott, Reference Walcott1910). USNM 25 is the type locality for Protocaris marshi Walcott, Reference Walcott1884, and Tuzoia polleni Resser, Reference Resser1929, both of which occur in poorly oxygenated mudstones characteristic of Burgess Shale-type preservation. Our own observations reject the possibility of soft-bodied fossil collections from the arenaceous dolomites that cap the productive, interbedded mudstone layers. Marcou's (Reference Marcou1880, p. 24) view that “near the top of the quarry, the shale became sandy and slightly calcareous … It was in this interval that most of the trilobite specimens were found” (translated from French) is misleading. USNM localities 25 and 319m should be equated (Resser and Howell, Reference Resser and Howell1938). Their faunas are virtually indistinguishable (Shaw, Reference Shaw1954) and similar to ours; they likely refer to a lost site (or nearby sites: Yochelson, Reference Yochelson1998) on the south-western flank of Parker's Cobble where we have carried out our own excavations (44°44′N, 73°09′W). Under these assumptions, the productive layers of the Parker Quarry could not have exceeded 8–10 m and not extended to 18.3 m or even 27.4 m (Webster and Landing, Reference Webster and Landing2016). The new fossils described here came from an interval of just 3 m within this larger thickness (Pari et al., Reference Pari, Briggs and Gaines2021).
Webster and Landing (Reference Webster and Landing2016) provided a detailed review of the geology and stratigraphic setting of the Parker Quarry Lagerstätte within the Parker Formation (see also Ratcliffe et al., Reference Ratcliffe, Stanley, Gale, Thompson and Walsh2011). Determination of the biostratigraphic position of the fauna is hampered by the lack of an up-to-date taxonomic treatment of most of the trilobites, many of which are known only from northwestern Vermont. Webster and Landing (Reference Webster and Landing2016) tentatively correlated the Parker Quarry Lagerstätte with the Bolbolenellus euryparia Zone or overlying Nephrolenellus multinodus Zone of the Laurentian Cordilleran margin within Dyeran Series 2, Stage 4. The exceptionally preserved fossils of the Kinzers Formation of Pennsylvania likewise have been assigned to Series 2, Stage 4 (Skinner, Reference Skinner2005; Webster and Landing, Reference Webster and Landing2016), older than the Burgess Shale, which falls in Miaolingian (formerly Series 3), Wuliuan.
Sedimentology
The mudstone-dominated strata of the Parker Formation at Parker's Cobble have undergone low-grade metamorphism resulting in a bedding-parallel cleavage, which facilitates splitting. Sedimentary features are largely obscured in the field by pyrite weathering, which stains surfaces with iron oxides, but they are clearly revealed in polished slabs (Pari et al., Reference Pari, Briggs and Gaines2021). The soft-bodied fossils occur in claystone event beds that, combined with evidence for graded bedding and a lack of wave influence, indicate deposition from mud-rich flows below storm wave base (Pari et al., Reference Pari, Briggs and Gaines2021). Trilobites occur in the same beds and in other mudstone lithologies, but they have not been observed in sandstone beds (Pari et al., Reference Pari, Briggs and Gaines2021). The setting, near the foot of a major break in slope (Landing, Reference Landing2007; Webster and Landing, Reference Webster and Landing2016), is similar to that of the Burgess Shale at the base of the Cathedral Escarpment (Conway Morris, Reference Conway Morris1986; Aitken, Reference Aitken1997) and, as in the Burgess Shale, slump folds and other evidence of synsedimentary deformation are abundant in the ~3 m interval excavated for this study (Pari et al., Reference Pari, Briggs and Gaines2021). The complete absence of evidence for bioturbation on bedding planes as well as polished cross-sections, and the enrichment in molybdenum in the fossil-bearing layers, suggest low oxygen levels (Pari et al., Reference Pari, Briggs and Gaines2021).
EDS mapping (Fig. 1) of two little-weathered specimens in close proximity (Figs. 4.1, 9.9; Pari et al., Reference Pari, Briggs and Gaines2021, fig. 2G) reveals areas preserved as a thin carbonaceous film, the primary mode of Burgess Shale-type preservation. The maps show that other elements are present on the surface of the fossils in proportions similar to the matrix. Most specimens of the new bivalved arthropod Vermontcaris montcalmi n. gen. n. sp. preserve traces of the gut, indicating that carcasses, and not molts, dominate. The preservation of soft and lightly sclerotized tissues indicates that soft-bodied fossils are rare due to unfavorable living conditions rather than loss through decay (Pari et al., Reference Pari, Briggs and Gaines2021).
The preservation of highly labile tissues, such as the myomeres and eyes of chordates, in a relatively low-diversity soft-bodied assemblage in which fossils are not abundant, is an aspect of the Parker Quarry Lagerstätte that appears to be unique among Burgess Shale-type deposits. Preservation of such tissues generally occurs in association with a high abundance of soft-bodied fossils and with the highest diversity assemblages (Tier 1 of Gaines, Reference Gaines2014), and has been interpreted to reflect settings where biostratinomic and diagenetic conditions for Burgess Shale-type preservation were optimized.
In many typical lower-diversity Burgess Shale-type assemblages, fossils were conserved under conditions that were persistently anoxic, yet in locations far enough from habitable benthic environments to render transportation to the preservation environment unlikely under low-energy depositional regimes dominated by claystone sediments (Gaines and Droser, Reference Gaines and Droser2010; Gaines, Reference Gaines2014). A notable aspect of the sedimentology of the Parker Quarry Lagerstätte, however, is the interbedding of sandstone and siltstone, and the presence of graded beds capped by massive claystones, indicating episodes of energetic deposition (Pari et al., Reference Pari, Briggs and Gaines2021), in contrast to many outer-shelf Burgess Shale-type deposits, where mm-laminated claystones dominate (Gaines, Reference Gaines2014). The fine-grained fossil-bearing claystones are interpreted as the distal expression of energetic flows, which had sufficient power to transport organisms across chemical gradients (Pari et al., Reference Pari, Briggs and Gaines2021); experimental studies have shown that delicate soft-bodied organisms can withstand significant transport (Allison, Reference Allison1986; Bath Enright et al., Reference Bath Enright, Minter, Sumner, Mángano and Buatois2021). The preservation of trilobite molt associations, however, suggests that trilobites intermittently colonized the seafloor locally (Whittington, Reference Whittington1988; Webster, Reference Webster2009), although the activity of infaunal organisms was consistently excluded. The setting of the Parker Quarry Lagerstätte near the toe of a substantial break in depositional slope (Landing, Reference Landing2007; Webster and Landing, Reference Webster and Landing2016), as in the case of the Burgess Shale, appears to have been the key in promoting energetic conditions that resulted in the transport and burial of soft-bodied fossils, and ultimately, the remarkable preservation of labile tissues within many specimens.
Taphonomy
The major challenge to understanding the mode of preservation of soft-bodied fossils in the Parker Quarry Lagerstätte is weathering of the surfaces along which soft-bodied fossils occur. At Parker's Cobble, the interbedded mudstones exhibit a strong slaty cleavage that is parallel to bedding and therefore favorable for fossil quarrying (Pari et al., Reference Pari, Briggs and Gaines2021), but also provides pathways for groundwater flow through the rock. A major consequence of this fluid flow is the oxidation of a portion of a substantial pyrite component of the slate, and Fe-oxide staining of surfaces and features of individual fossils (e.g., Figs. 2.2, 6, 10.5; Pari et al., Reference Pari, Briggs and Gaines2021). Optical microscopy and elemental mapping also indicate that native S is present as veneers on some surfaces. Although S may be present in excess of Fe, optical microscopy and bulk chemical analyses (Pari et al., Reference Pari, Briggs and Gaines2021) indicate that both are derived from oxidation of pyrite and redistribution across joints and fractures during recent oxidative weathering. Nevertheless, several fossils occur on relatively fresh, although not pristine, surfaces. These were selected for elemental mapping (see Material and methods).
Soft-bodied fossils of Burgess Shale-type are preserved as carbonaceous compressions, with anatomical features of some taxa replicated in calcium phosphate or pyrite (Butterfield, Reference Butterfield2002; Gabbott et al., Reference Gabbott, Hou, Norry and Siveter2004; Gaines et al., Reference Gaines, Briggs and Zhao2008; Edgecombe et al., Reference Edgecombe, Ma and Strausfeld2015), although debate has persisted regarding the role of pyrite and aluminosilicate-like minerals as pervasive mineral phases that capture soft-bodied anatomy (Orr et al., Reference Orr, Briggs and Kearns1998; Butterfield et al., Reference Butterfield, Balthasar and Wilson2007; Gaines, Reference Gaines2014; Schiffbauer et al., Reference Schiffbauer, Xiao, Cai, Wallace, Hua, Hunter, Xu, Peng and Kaufman2014; Broce and Schiffbauer, Reference Broce and Schiffbauer2017; Anderson et al., Reference Anderson, Tosca, Saupe, Wade and Briggs2021). The two soft-bodied fossils that were mapped here (Fig. 1), a possible priapulid (?Ottoia) (Fig. 4.1) and the new bivalved arthropod Vermontcaris montcalmi n. gen. n. sp. (Fig. 9.9), which occur in close proximity on the same bedding plane (Fig. 1.1, Pari et al., Reference Pari, Briggs and Gaines2021, fig. 2G), provide evidence of the nature of soft-bodied preservation within the Parker Quarry Lagerstätte.
In the ?priapulid map (Fig. 1.1, area 2, 1.2), C picks out areas of the fossil that appear reflective to the naked eye. Carbon is most enriched in the structure interpreted as the gut, but also highlights cuticular annulations. The absence of Ca indicates that C within the fossil is organic in origin and not a constituent of a carbonate mineral. The distributions of Si, Al, and O, the core constituents of clay minerals, are essentially the inverse of C. Silicon is elevated on parts of the gut, but otherwise there is a consistently stronger signal of Si, O, and Al in the matrix than within the fossil. The distributions of K and Mg are similar to those of Si, O, and Al, but the signal of these two elements is significantly weaker.
Mapping of V. montcalmi n. gen. n. sp. (Fig. 1.1, area 3, 1.3) revealed elemental distributions similar to the ?priapulid. Areas of V. montcalmi n. gen. n. sp. that appear reflective to the naked eye are characterized by enrichment in C, and depleted in the matrix-forming elements Al, Si, and O. The inverse relationship of C versus Al, Si, and O in both fossils suggests that the range of elemental variation is largely controlled by variability in the thickness of the carbonaceous material that comprises them, which determines the degree to which the EDS signal of elements within the matrix is suppressed. Although C is particularly enriched in areas of both fossils, most regions of the fossils show no compositional difference to the matrix, a finding that has been interpreted for Burgess Shale-type fossils to represent the presence of vanishingly thin carbonaceous films with a C concentration below the detection limits of EDS (Gabbott et al., Reference Gabbott, Hou, Norry and Siveter2004; Gaines et al., Reference Gaines, Briggs and Zhao2008).
Sulfur picks out the entire cuticle of the ?priapulid more clearly than any other element. The distribution of Fe is similar, but its signal is much weaker and exhibits a clear inverse relationship with C. The distribution of S also corresponds well to the overall morphology of Vermontcaris n. gen., in contrast to the distribution of Fe in this specimen. Although correspondence of Fe and S can indicate the presence of pyrite, no crystals of pyrite are evident in either fossil under microscopy. EDS spectra of the ?priapulid suggest that S is >4 times as abundant as Fe, whereas the expected S to Fe ratio in pyrite is 2:1. Iron is less conspicuous in V. montcalmi n. gen. n. sp. and shows weaker correspondence with S. The correspondence of Fe and O suggests that Fe is present in both fossils as an oxide, whereas the distribution of S suggests that it is in elemental form. The reddish hue of Fe-rich areas also suggests that Fe is present as an oxide rather than a sulfide phase. The most parsimonious interpretation, in the absence of evidence for pyrite crystals, is that both Fe and S represent secondary veneers over the cuticle that were derived from the weathering of pyrite in the bulk rock. Neither Fe nor S are enriched in bulk rock relative to composite shale standards (Pari et al., Reference Pari, Briggs and Gaines2021).
Most specimens of V. montcalmi n. gen. n. sp. exhibit 3-dimensional preservation of the gut (Figs. 7–9). EDS spot analyses and elemental mapping confirm that the gut filling is comprised of matrix sediment, rather than an authigenic mineral such as calcium phosphate, which is a relatively common replacement in Burgess Shale-type (BST) fossils that captures anatomical information in arthropod guts and other soft-tissue features (Butterfield, Reference Butterfield2002; Gaines et al., Reference Gaines, Briggs and Zhao2008; Aria et al., Reference Aria, Caron and Gaines2015; Nanglu and Caron, Reference Nanglu and Caron2018). Three-dimensional preservation of the gut by sediment filling is conspicuously different from most BST fossils, but is prominent in some Chengjiang arthropods (Hou et al., Reference Hou, Siveter, Siveter, Aldridge, Cong, Gabbott, Ma, Purnell and Williams2017). It may reflect ingestion of sediment during turbid transport or deposit feeding.
In summary, data from elemental mapping support carbonaceous compressions as the primary mode of preservation of the soft-bodied fossils of the Parker Quarry Lagerstätte. Although pyrite is abundant within the sediments (Pari et al., Reference Pari, Briggs and Gaines2021), it was rarely found in association with soft-bodied fossils (the specimen of the sponge illustrated in Fig. 3.4 is an exception). Authigenic clays or aluminosilicate-like minerals are also absent from the soft-bodied fossils. The formation of clay minerals during BST fossilization has been interpreted as a product of early diagenesis (Anderson et al., Reference Anderson, Tosca, Saupe, Wade and Briggs2021) or metamorphism (Butterfield et al., Reference Butterfield, Balthasar and Wilson2007). In the latter case, authigenic clay minerals would be expected to have formed within the Parker Quarry Lagerstätte soft-bodied fossils as carbonaceous materials were volatilized during slate grade metamorphism. The unusual nature of the fossils from the Burgess Shale in British Columbia may reflect the alteration of most early diagenetic clay during the higher, greenschist grade of metamorphism experienced by that unit (Anderson et al., Reference Anderson, Tosca, Saupe, Wade and Briggs2021).
Material and methods
Two trenches exposing surface areas of ~50 m2 and 25 m2 were excavated to depths of up to 3 m using hand tools on the south-west flank of Parker's Cobble. Both trenches yielded soft-bodied fossils, at a combined rate of 0.7 specimens per m3 (exclusive of algae). The new specimens were collected by GP between 2016 and 2019, except for a very few collected under his direction, by splitting large slabs of slate (Pari et al., Reference Pari, Briggs and Gaines2021).
The elemental maps (Fig. 1) were generated with an EDS X-ray detector on an environmental scanning microscope (FEI Quanta 200 FEG) at the University of Windsor Great Lakes Institute for Environmental Research (Canada). The maps were run at 12 kV, spot size 5, map resolution 512 × 400 pixels, dwell time 200 μs; each field of view (1.93 mm wide) was scanned eight times, and the data collated. In the case of the possible priapulid (YPM IP 239053), there were 228 fields of view and the map ran for 25 hours, 7 minutes; in the case of Vermontcaris montcalmi n. gen. n. sp. (YPM IP 543321), there were 192 fields of view and the map ran for 21 hours, 10 minutes. In addition, we conducted elemental mapping and spot analyses of several other specimens described herein using a Hitachi SU7000 at Yale, operated at 15 kv in VP mode at 100 pascals.
Repositories and institutional abbreviations
Smithsonian Institution (USNM); Yale Peabody Museum of Natural History (YPM).
Systematic paleontology
?Division Chlorophyta
Genus Fuxianospira Chen and Zhou, Reference Chen and Zhou1997
Type species
Fuxianospira gyrata Chen and Zhou, Reference Chen and Zhou1997, from the Yuanshan Formation, Chengjiang, Yunnan, China, by monotypy.
Fuxianospira gyrata Chen and Zhou, Reference Chen and Zhou1997
Figure 2
- 2021
Fuxianospira gyrata Chen and Zhou, Reference Chen and Zhou1997; Wang et al., Reference Wang, LoDuca, Wu, Tang and Sun2021, p. 3, figs. 4–6 (includes a synonymy).
Holotype
Early Life Research Center, Chengjiang ELRC80001, Yuanshan Formation, Chengjiang, Yunnan, China (Chen and Zhou, Reference Chen and Zhou1997, p. 88, fig. 143).
Description
Masses of fragments of poorly preserved, carbonaceous strap-like features are preserved as reflective films (Fig. 2.1) or, in some cases, with a covering of iron oxide, presumably the result of weathering pyrite (Fig. 2.2). The straps are mainly straight, but many show curvature and folding, and they occasionally appear to branch. They are parallel sided, ~0.5–1.0 mm wide, with irregular undulating margins and convex terminations. They rarely exceed 2 cm in length. No structure is evident on the cuticle.
Material
YPM IP 239095, part and counterpart; YPM IP 239097.
Discussion
The specimens fall into Morphogroup 1 of LoDuca et al. (Reference LoDuca, Bykova, Wu, Xiao and Zhao2017) among early Paleozoic non-calcified macroalgae, and their morphology is consistent with that of Fuxianospira, which is common in the Chengjiang biota (LoDuca et al., Reference LoDuca, Wu, Zhao, Xiao, Schiffbauer, Caron and Babcock2015b, Reference LoDuca, Bykova, Wu, Xiao and Zhao2017; Wang et al., Reference Wang, LoDuca, Wu, Tang and Sun2021). The undulating margins reflect the coiled structure, although the preservation, like that of occurrences of Fuxianospora elsewhere (LoDuca et al., Reference LoDuca, Wu, Zhao, Xiao, Schiffbauer, Caron and Babcock2015b; Wang et al., Reference Wang, LoDuca, Wu, Tang and Sun2021), is inadequate to show the morphology in detail. The apparent branching is likely the result of overlap (see LoDuca et al., Reference LoDuca, Wu, Zhao, Xiao, Schiffbauer, Caron and Babcock2015b, fig. 1). The thalli of Sinocylindra, which is also from the Chengjiang biota, tend to be longer and thinner, with smoother margins (Wang et al., Reference Wang, LoDuca, Wu, Tang and Sun2021). We follow LoDuca et al. (Reference LoDuca, Wu, Zhao, Xiao, Schiffbauer, Caron and Babcock2015b) and Wang et al. (Reference Wang, LoDuca, Wu, Tang and Sun2021) in tentatively assigning Fuxianospira to Chlorophyta.
Remarks
Similar specimens from other Cambrian localities were assigned mainly to Yuknessia simplex Walcott, Reference Walcott1919, which was originally described from the Burgess Shale and interpreted by Walcott (Reference Walcott1919) as an alga. LoDuca et al. (Reference LoDuca, Caron, Schiffbauer, Xiao and Kramer2015a) showed that most of Walcott's Burgess Shale specimens preserve banding on the cuticle and identified them as a benthic colonial pterobranch hemichordate (see also Ramírez-Guerrero and Cameron, Reference Ramírez-Guerrero and Cameron2021); just a few of Walcott's specimens turned out to be algal. However, LoDuca et al. (Reference LoDuca, Wu, Zhao, Xiao, Schiffbauer, Caron and Babcock2015b) also investigated specimens attributed to Yuknessia from the Chengjiang biota in China and other Cambrian Lagerstätten in North America, and assigned them to the alga Fuxianospora Chen and Zhou, Reference Chen and Zhou1997.
Phylum Porifera Grant, Reference Grant and Todd1836
Class Ascospongiae Botting, Reference Botting2021
Family Leptomitidae de Laubenfels, Reference de Laubenfels and Moore1955
Genus Leptomitus Walcott, Reference Walcott1886
Type species
Leptomitus zitteli Walcott, Reference Walcott1886, Parker Formation, Parker Quarry, Franklin County, Vermont, USA.
Leptomitus zitteli Walcott, Reference Walcott1886
Figure 3.1–3.7
- Reference Rigby1987
Leptomitus zitteli Walcott, Reference Walcott1886; Rigby, p. 453, fig. 3.3–3.5 (includes a synonymy).
- Reference Pari, Briggs and Gaines2021
Leptomitus; Pari et al., p. 693, fig. 2I.
Lectotype
USNM 15308, Parker Formation, Parker Quarry, Franklin County, Vermont, USA. Cambrian Series 2, Stage 4 (Rigby, Reference Rigby1987, fig. 3.3–3.5).
Occurrence
Leptomitus zitteli is presently known only from the Parker Formation at Parker's Cobble (Rigby, Reference Rigby1987; García-Bellido et al., Reference García-Bellido, Gozalo, Chirivella Martorell and Liñán2007).
Description
Rigby (Reference Rigby1987) described the spicular structure of this elongate tubular sponge, which comprises an outer layer of parallel oxeas and an inner layer of tiny horizontal spicules (Fig. 3.1, 3.3). The lectotype (USNM 15308) reveals a plumose arrangement of spicules at the distal end (Fig. 3.2), which may represent their arrangement in the interior of the skeleton (Rigby, Reference Rigby1987). It is difficult to distinguish between coarse and fine long vertical oxeas and to determine their length. Dimensions of the sponge vary between specimens. YPM IP 543326 is a fragment ~70 mm long consisting of a bundle of oxeas, roughly aligned, with some diverging at either end (Fig. 3.4). The specimen is preserved in pyrite, which prevents individual spicules from being distinguished. USNM 15308, which is ~70 mm long, varies in width from 10–2 mm along its length. YPM IP 239151 (Fig. 3.5–3.7) is longer than other specimens from this locality at 12 cm, although incompletely exposed, but only 2–4 mm wide along its length. The distribution of the spicules indicates some decay and exfoliation along its length with small fragments displaced laterally and evidence that the outer layer has separated at one point about halfway along the preserved length, giving the appearance of branching. This is consistent with the occurrence of sponge fragments such as YPM IP 543326 (Fig. 3.4) and YPM IP 239096.
Material
USNM 15308, lectotype, and USNM 419730 and 419732 on the same block, part and counterpart. USNM 419731, part and counterpart, on a different block, YPM IP 239096, YPM IP 239151, YPM IP 239152, YPM IP 543326.
Remarks
Rigby (Reference Rigby1986) published a generalized reconstruction of Leptomitus based on his revision of L. lineatus (Walcott, Reference Walcott1920) from the Burgess Shale. The following year he provided a full description of Walcott's material of L. zitteli, and a synonymy (Rigby, Reference Rigby1987). He designated as lectotype the best-preserved specimen, USNM 15308, part and counterpart, which Walcott (Reference Walcott1886, legend to plate 2) referred to as the type specimen (the other two specimens on the same block now carry the numbers USNM 419730 and 419732). A specimen on a second block and two non-contiguous fragments of its counterpart were also originally included under USNM 15308, but that individual is now numbered USNM 419731.
Class Hexactinellida Schmidt, Reference Schmidt1870
Order Reticulosa Reid, Reference Reid1958
Superfamily Protospongioidea Finks, Reference Finks1960
Family Protospongiidae Hinde, Reference Hinde1887
Genus ?Protospongia Salter, Reference Salter1864
Type species
Protospongia fenestrata Salter, Reference Salter1864, Menevian Group, Porthyrhaw, St. Davids, Wales.
?Protospongia hicksi Hinde, Reference Hinde1887
Figure 3.8, 3.9
- Reference Rigby1987
?Protospongia hicksi Hinde, Reference Hinde1887; Rigby, p. 459, fig. 4.
Holotype
Sedgwick Museum, Cambridge SM A1035, Menevian Group, Porthyrhaw, St. Davids, Wales.
Occurrence
In addition to this probable occurrence in the Parker Formation at Parker's Cobble (Rigby, Reference Rigby1987), Protospongia hicksi is present in younger North American Cambrian Lagerstätten, including the Burgess Shale (Rigby, Reference Rigby1986) and the Trilobite Beds on Mount Stephen (Rigby and Collins, Reference Rigby and Collins2004). Spicules closely resembling those of Protospongia also have been reported from the upper part of the Kinzers Formation near Lancaster, Pennsylvania, which is similar in age to the Mount Stephen Trilobite Beds (Campbell, Reference Campbell1971).
Material
Fragments and isolated spicules associated with USNM 15308, USNM 419731, and USNM 419732.
Remarks
We follow Rigby (Reference Rigby1987) in tentatively assigning these spicules to ?Protospongia hicksi. In doing so, however, we acknowledge that their morphology is poorly constrained. Protospongia and similar taxa are in need of revision (Botting and Muir, Reference Botting and Muir2018) and may be more appropriately assigned to Ascospongiae than to Hexactinellida (Botting, Reference Botting2021). Rigby (Reference Rigby1987) recorded ?Protospongia hicksi in intimate association with the specimens of L. zitteli on the slab that includes the lectotype USNM 15308. Rigby (Reference Rigby1987, p. 459) noted that the lectotype, in its middle part, appears to have “buried a partially intact fragment of a protosponge” and (Reference Rigby1987, p. 460) “isolated Protospongia-like stauracts occur scattered throughout matrix between Leptomitus fragments.” He also observed first order quadrules associated with USNM 419732. These Protospongia spicules were not noted by Walcott (Reference Walcott1886). We illustrate examples of these cruciform spicules from Walcott's original specimens here (Fig. 3.8, 3.9). We did not find examples associated with the specimens of Leptomitus discovered during our excavations.
Superphylum Ecdysozoa Aguinaldo et al., Reference Aguinaldo, Turbeville, Linford, Rivera, Garey, Raff and Lake1997
Phylum ?Priapulida Delage and Hérouard, Reference Delage and Hérouard1897, stem group
Class uncertain
Order uncertain
Family ?Ottoiidae Walcott, Reference Walcott1911
Genus ?Ottoia Walcott, Reference Walcott1911
Type species
Ottoia prolifica Walcott, Reference Walcott1911 (Walcott, Reference Walcott1911b), Burgess Shale, British Columbia, Canada.
?Ottoia sp.
Figure 4
- Reference Pari, Briggs and Gaines2021
Priapulid reminiscent of Ottoia; Pari et al., p. 695, fig. 2G.
Description
The specimen, YPM IP 239053, which is ~9.5 cm long, with a maximum width of 8.5–9 mm, is tightly coiled (Fig. 4). It narrows gradually toward one end, curving sharply and expanding slightly at the termination, resulting in an outline reminiscent of a proboscis. The other end tapers more abruptly. Details are poorly preserved, but the body preserves evidence of transverse annulations and a wide reflective structure in the anterior portion that may represent the gut.
Material
YPM IP 239053.
Remarks
The annulations and presumed proboscis suggest a priapulid affinity, and the outline of the specimen is similar to Ottoia, among Cambrian genera. Smith et al. (Reference Smith, Harvey and Butterfield2015) demonstrated the value of sclerites in distinguishing species of ottoiids and they cautioned against identifying indeterminate priapulids that do not preserve such characters. On this basis, we assign our specimen to Ottoia with a query. Smith et al. (Reference Smith, Harvey and Butterfield2015, p. 706) noted that “there are no confirmed records of Ottoia macrofossils outside the Burgess Shale,” but Y. Yang et al. (Reference Yang, Zhao and Zhang2016) described a new species based on multiple body fossils from the Kaili Formation in South China, demonstrating the occurrence of the genus outside Laurentia. Resser and Howell (Reference Resser and Howell1938, p. 215) tentatively assigned a specimen from the Kinzers Formation to Ottoia, but Conway Morris (Reference Conway Morris1977, p. 85) identified it as a palaeoscolecid. If the Parker Formation specimen were Ottoia, it would be the oldest macrofossil representative of the genus discovered to date. Sansom (Reference Sansom2016) noted the decay-resistant nature of sclerites in living priapulids, and Howard et al. (Reference Howard, Edgecombe, Shi, Hou and Ma2020) indicated that their absence is a feature of stem ecdysozoans. YPM IP 239053 preserves cuticle annulations in places, but the distribution of carbon on the specimen and the associated Vermontcaris montcalmi n. gen. n. sp., is patchy (fig. 1), presumably as a result of degradation. Thus the apparent absence of scalids on the presumed proboscis may or may not be real (Howard et al., Reference Howard, Edgecombe, Shi, Hou and Ma2020). Thus the fossil may represent a stem ecdysozoan similar to Acosmia maotiania Chen and Zhou, Reference Chen and Zhou1997, rather than a stem priapulid.
The specimen shows some similarity to Atalotaenia adela García-Bellido and Conway Morris, Reference García-Bellido and Conway Morris1999, from the Kinzers Formation (García-Bellido and Conway Morris, Reference García-Bellido and Conway Morris1999). However, the single reported specimen of A. adela is incomplete, it preserves a ribbon-like structure for which there is no equivalent in YPM IP 239053, and the annulations are more widely separated. García-Bellido and Conway Morris (Reference García-Bellido and Conway Morris1999) assigned A. adela to Phylum, Class, Family uncertain.
Panarthropoda Nielsen, Reference Nielsen1995
Order Radiodonta Collins, Reference Collins1996
Family Amplectobeluidae Pates et al., Reference Pates, Daley, Edgecombe, Cong and Lieberman2021
Amplectobeluidae indet.
Figure 5
- Reference Pari, Briggs and Gaines2021
Radiodont; Pari et al., p. 695, fig. 2F.
Description
The specimen, which is preserved in lateral view, is comprised of the distalmost podomere of the shaft, which bears a large endite (= ventral spine: see Guo et al., Reference Guo, Pates, Cong, Daley, Edgecombe, Chen and Hou2019), and an articulated region of 13 podomeres, each bearing ventrally projecting endites with short lateral auxiliary spines (Fig. 5). The proximal part of the shaft and the endites, particularly in the more distal part of the appendage, are incomplete, which limits the detail available. Decay and disarticulation have resulted in separation of the appendage into two portions at the junction between podomeres 5 and 6 in the distal articulated region; the junction between podomeres 1 and 2 is also partially ruptured. Podomeres 6–9 of the articulated region are a little longer than those proximal to them, and the height (measured from the mid-point of the dorsal margin to apparent attachment of the endite) to length ratio of the podomeres averages ~2.0. It is not clear whether the endites are paired or not. The podomeres are separated by a gap, tapering dorsally, which represents the position of the articulating membrane. In spite of poor preservation it is clear that the endite on the distalmost podomere of the shaft and on the first podomere of the articulated region are enlarged (hypertrophied) compared to the rest (Fig. 5). The endite on podomere 1 of the articulated region is the largest, and three auxiliary spines radiate from its distal margin. The endite on podomere 2 is shorter than that on podomere 3. The endite on podomere 5 appears enlarged and was presumably longer than on podomere 3, based on the width at its base. Long robust dorsal spines are present on the distalmost podomeres, which curve strongly ventrally, and the thirteenth podomere bears two terminal spines. The specimen measures ~140 mm along its dorsal margin excluding the dorsal spines on the distal segments and the gap resulting from disarticulation.
Material
YPM IP 239052, part and counterpart.
Discussion
This specimen represents the first record of a radiodont from the Parker Formation and adds a new locality for this group on the Laurentian paleocontinent (Pates and Daley, Reference Pates and Daley2019).
Remarks
Although radiodont families are diagnosed on the basis of the complete morphology of the animal, and the mouth parts are particularly characteristic (Cong et al., Reference Cong, Edgecombe, Daley, Guo, Pates and Hou2018), specimens are often assigned, even to species, based on the frontal appendages (Pates et al., Reference Pates, Daley, Edgecombe, Cong and Lieberman2021). As far as can be determined, the morphology of the Parker Formation appendage does not correspond precisely to that of any previously described radiodont (see Cong et al., Reference Cong, Edgecombe, Daley, Guo, Pates and Hou2018; Guo et al., Reference Guo, Pates, Cong, Daley, Edgecombe, Chen and Hou2019; Pates and Daley, Reference Pates and Daley2019; Pates et al., Reference Pates, Daley, Edgecombe, Cong and Lieberman2021). It is incomplete proximally but likely comprises the distalmost podomere of the shaft, which bears a large hypertrophied endite, and an articulated region of 13 podomeres. It shows greatest similarity to Anomalocarididae and Amplectobeluidae, both of which include genera with paired endites. YPM IP 239052 differs from Anomalocarididae in the relative lengths of the endites and details of the auxiliary spines (Guo et al., Reference Guo, Pates, Cong, Daley, Edgecombe, Chen and Hou2019; Pates and Daley, Reference Pates and Daley2019; Pates et al., Reference Pates, Daley, Edgecombe, Cong and Lieberman2021). It shows greater similarity to the frontal appendage of Amplectobeluidae, which has a single hypertrophied proximal endite on the first podomere of the articulated portion, high/deep podomeres 12 or 13 in number, robust dorsal spines on the distalmost podomeres, and an endite on podomere 5 of the articulated region that is enlarged relative to that on podomere 3 (Cong et al., Reference Cong, Edgecombe, Daley, Guo, Pates and Hou2018; Guo et al., Reference Guo, Pates, Cong, Daley, Edgecombe, Chen and Hou2019; Pates et al., Reference Pates, Daley, Edgecombe, Cong and Lieberman2021). This last character is particularly evident in Ramskoeldia from the Chengjiang biota of China (Series 2, Stage 3), but that genus lacks a large endite on the shaft (Pates et al., Reference Pates, Daley, Edgecombe, Cong and Lieberman2021). The large height to length ratio of the podomeres, large first endite on the articulated region, and pronounced distal dorsal spines are shared with Amplectobelua (Daley and Budd, Reference Daley and Budd2010; Lerosey-Aubril et al., Reference Lerosey-Aubril, Kimmig, Pates, Skabelund, Weug and Ortega-Hernández2020), but the articulated region of the Parker Formation appendage includes 13 as opposed to 12 podomeres. The Parker Formation appendage shares more characters with Laminacaris chimera Guo et al., Reference Guo, Pates, Cong, Daley, Edgecombe, Chen and Hou2019, from the Chengjiang biota than with Amplectobelua, including a large endite on the shaft, 13 podomeres in the articulated region, and a larger endite on podomere 5 of that region than podomere 3 (Guo et al., Reference Guo, Pates, Cong, Daley, Edgecombe, Chen and Hou2019). Laminacaris has been recovered as an amplectobeluid in some phylogenetic analyses (Lerosey-Aubril and Pates, Reference Lerosey-Aubril and Pates2018; but see Moysiuk and Caron, Reference Moysiuk and Caron2021).
The phylogeny of radiodonts is not well resolved, but Anomalocarididae and Amplectobeluidae fall within the same clade (Cong et al., Reference Cong, Ma, Hou, Edgecombe and Strausfeld2014; Vinther et al., Reference Vinther, Stein, Longrich and Harper2014; Van Roy et al., Reference Van Roy, Daley and Briggs2015; Lerosey-Aubril and Pates, Reference Lerosey-Aubril and Pates2018; Moysiuk and Caron, Reference Moysiuk and Caron2021). The available evidence supports assignment of the Parker appendage to Amplectobeluidae. It shows similarities to a number of genera, but we do not assign it beyond family here in view of the incomplete preservation. The Parker Formation appendage may represent a new genus and species within this group.
Class uncertain
Order Tuzoida Simonetta and Delle Cave, Reference Simonetta and Delle Cave1975
Family Tuzoiidae Raymond, Reference Raymond1935
Genus Tuzoia Walcott, Reference Walcott1912
Type species
Tuzoia retifera Walcott, Reference Walcott1912 (Walcott, Reference Walcott1912a), Burgess Shale, British Columbia, Canada.
Tuzoia polleni Resser, Reference Resser1929
Figure 6.1–6.10
- Reference Walcott1890
Problematical organic marking; Walcott, pl. 59, fig. 1.
- Reference Resser and Howell1938
Tuzoia vermontensis Resser and Howell, p. 231, pl. 13, fig. 1.
- Reference Lieberman2003
Tuzoia polleni Resser, Reference Resser1929; Lieberman, p. 681.
- Reference Vannier, Caron, Yuan, Briggs, Collins, Zhao and Zhu2007
Tuzoia polleni Resser, Reference Resser1929; Vannier et al., p. 460, fig. 17.2, p. 462 (includes a synonymy).
- Reference Pari, Briggs and Gaines2021
Tuzoia polleni Resser, Reference Resser1929; Pari et al., p. 694, fig. 2B, C.
Holotype
USNM 80485, Eager Formation, Cranbrook, British Columbia, Canada (Resser, Reference Resser1929, pl. 5, fig. 1).
Description
USNM 26728 (Fig. 6.1, 6.2) represents a single right valve with an unusual subcircular outline. It preserves four spines on the posterior margin and perhaps five along the hinge line (only the posteriormost of these five is clearly preserved, the others are somewhat equivocal and have been outlined in pencil on the specimen). YPM IP 239051 (Fig. 6.3, 6.4) and YPM IP 239150 (Fig. 6.5, 6.6) also reveal the complete valve outline. The ventral margin of most of the specimens is followed by a well-delineated wide margin (Fig. 6.3–6.8), which may be modified by compression. YPM IP 239051 (Fig. 6.3, 6.4) preserves at least four spines around the posterior margin. There is also at least one spine on the anterior margin and there may be several on the hinge line, but these cannot be discerned with confidence due to the nature of the preservation. YPM IP 239150 (Fig. 6.5, 6.6), which preserves both valves slightly offset, preserves two slender posterior spines on each valve. YPM IP 239149 (Fig. 6.7, 6.8) and YPM IP 543331 (Fig. 6.9, 6.10) are incompletely exposed. They preserve four and seven spines, respectively, around the posterior margin; in neither case are spines evident on the hinge. Most of the specimens preserve a trace of the lateral ridge (Fig. 6.1–6.4, 6.7, 6.8) roughly aligned with the mid-posterior spine, its position and shape varying with the orientation and flattening of the carapace. Spines are not evident along the ridge, but these are difficult to discern when flattened onto the valve.
USNM 26728 (Fig. 6.1, 6.2) is 8 cm long and 7.6 cm high excluding spines (height/length, H/L 0.95). YPM IP 239051 (Fig. 6.3, 6.4) is 7.4 cm long and 4.7 cm high (H/L 0.64). YPM IP 239149 (Fig. 6.7, 6.8) and YPM IP 543331 (Fig. 6.9, 6.10) are ~3.9 cm high and ~6.2 cm high, respectively, based on extrapolations from the exposed parts of the valves. YPM IP 239150 (Fig. 6.5, 6.6) preserves both valves offset; the completely exposed (?left) valve is ~4.8 cm long and 3.2 cm high (H/L 0.67).
Material
USNM 26728, the only previously known specimen of Tuzoia from this locality, and four new specimens with part and counterpart: YPM IP 543331, YPM IP 239051, YPM IP 239149, YPM IP 239150.
Discussion
The wide ventral margin in the new specimens from the Parker Quarry Lagerstätte is similar to that in Tuzoia sp. from the Emu Bay Shale of Australia, which was interpreted as a doublure (García-Bellido et al., Reference García-Bellido, Paterson, Edgecombe, Jago, Gehling and Lee2009). Variability in the preservation of the carapace outline and spines makes the identification of species of Tuzoia difficult (Vannier et al., Reference Vannier, Caron, Yuan, Briggs, Collins, Zhao and Zhu2007). The shape and number of spines may change during ontogeny (Wen et al., Reference Wen, Zhao and Peng2015). The number of spines evident around the margin of the valves also shows intraspecific variability, which may reflect morphologic differences and/or taphonomic processes (Liebermann, Reference Lieberman2003; Vannier et al., Reference Vannier, Caron, Yuan, Briggs, Collins, Zhao and Zhu2007). USNM 26728 (Fig. 6.1, 6.2) shows a much greater height to length ratio than YPM IP 239051 (Fig. 6.3, 6.4) and YPM IP 239150 (Fig. 6.5, 6.6). This might be a result of burial of USNM 26728 with the valve tilted at an angle to bedding and consequent reduction of the length, but, apart from a hint of concentric lines close to the anterior margin of the valve, there is no independent evidence that this is the case. Specimens of the new arthropod Vermontcaris montcalmi n. gen. n. sp., however, clearly vary in outline as a consequence of flattening in different attitudes to bedding following transport and burial (Figs. 7–9). Such variation in orientation is analogous with that in the Burgess Shale and is consistent with evidence that the Parker Quarry Lagerstätte sediments were deposited from dense mud-rich flows (Pari et al., Reference Pari, Briggs and Gaines2021). Carapaces of Tuzoia from other localities are also characterized by variation in outline (Vannier et al., Reference Vannier, Caron, Yuan, Briggs, Collins, Zhao and Zhu2007).
If the length of USNM 26728 (Fig. 6.1, 6.2) does reflect compaction at an angle to bedding, its original length can be estimated based on the height to length ratio of YPM IP 239051 (Fig. 6.3, 6.4), which would extend it from 8 cm to 11.9 cm. This exceeds the maximum length of ~10 cm reported for T. polleni by Vannier et al. (Reference Vannier, Caron, Yuan, Briggs, Collins, Zhao and Zhu2007, p. 462). Valves of Tuzoia up to ~18 cm long have been reported from the Cambrian Miaolingian (Drumian) of Bohemia (Chlupác and Kordule, Reference Chlupác and Kordule2002, fig. 3), but the largest North American examples previously noted, specimens of T. retifera from the Burgess Shale, reached maximum lengths of ~12 cm (Vannier et al., Reference Vannier, Caron, Yuan, Briggs, Collins, Zhao and Zhu2007).
Remarks
Walcott (Reference Walcott1890) figured USNM 26728 (Fig. 6.1, 6.2), without description, noting it as “a problematical organic marking,” and the specimen was subsequently labeled as Medusa? sp. (Resser and Howell, Reference Resser and Howell1938, p. 231). Resser and Howell (Reference Resser and Howell1938) described it as Tuzoia vermontensis, a new species of the genus that Walcott (Reference Walcott1912a) had erected for Burgess Shale material. Lieberman (Reference Lieberman2003) synonymized all the species of Tuzoia (T. polleni, T. nodosa Resser, Reference Resser1929, and T. spinosa Resser, Reference Resser1929) that Resser (Reference Resser1929) originally described from the Eager Formation, assigning them to T. polleni. Lieberman (Reference Lieberman2003) did not examine the original specimen of T. vermontensis, USNM 26728, but interpreted it as a single valve and synonymized it with T. polleni because of the presence of spines on the entire margin. Vannier et al. (Reference Vannier, Caron, Yuan, Briggs, Collins, Zhao and Zhu2007) went further and synonymized T. nitida Resser and Howell, Reference Resser and Howell1938, from the Kinzers Formation with T. polleni. They tentatively retained T. vermontensis as a synonym of T. polleni (Vannier et al., Reference Vannier, Caron, Yuan, Briggs, Collins, Zhao and Zhu2007, p. 463), but they interpreted the spines along the dorsal margin (hinge line) as ridge spines on the right valve (Vannier et al., Reference Vannier, Caron, Yuan, Briggs, Collins, Zhao and Zhu2007, fig. 17.2) based on their erroneous interpretation of USNM 26728 as a dorsoventrally compacted (‘butterflied’) specimen showing both valves.
The Parker Quarry Lagerstätte specimens vary in outline and the distribution of spines (Fig. 6). Identification is compromised by the lack of a dorsoventrally flattened specimen, which might show spines on the lateral ridge; clear evidence of reticulation is also absent. The morphological features of the Parker Quarry Lagerstätte specimens show a general similarity to T. canadensis Resser, Reference Resser1929, as well as to T. polleni (Pari et al., Reference Pari, Briggs and Gaines2021; see Vannier et al., Reference Vannier, Caron, Yuan, Briggs, Collins, Zhao and Zhu2007, fig. 25.3, 25.5). We follow Lieberman (Reference Lieberman2003) and Vannier et al. (Reference Vannier, Caron, Yuan, Briggs, Collins, Zhao and Zhu2007) in synonymizing the Parker Formation T. vermontensis with T. polleni. We note, however, that future discoveries and analysis may show otherwise, or even that the new specimens may represent a different species to Walcott's original, in which case more than one species is present in the Parker Formation, as in the Burgess Shale (Vannier et al., Reference Vannier, Caron, Yuan, Briggs, Collins, Zhao and Zhu2007) and the Balang Formation of Guizhou, China (Wen et al., Reference Wen, Babcock, Peng, Liu and Liang2019).
Class uncertain
Family Protocarididae Miller, Reference Miller1889
Genus Protocaris Walcott, Reference Walcott1884
Type species
Protocaris marshi Walcott, Reference Walcott1884, Parker Formation, Parker Quarry, Franklin County, Vermont, USA.
Protocaris marshi Walcott, Reference Walcott1884
Figure 6.11
- Reference Briggs1976
Protocaris marshi Walcott, Reference Walcott1884; Briggs, p. 2, text figs. 1, 3, pl. 1, figs. 1–3 (includes a synonymy).
- Reference Budd2008
Protocaris marshi Walcott, Reference Walcott1884; Budd, p. 567.
- Reference Pari, Briggs and Gaines2021
Protocaris marshi Walcott, Reference Walcott1884; Pari et al., p. 693, fig. 2A.
Holotype and only known specimen
USNM 15400, Parker Formation, Parker Quarry, Franklin County, Vermont, USA.
Discussion
In the absence of additional specimens we cannot add to the description by Briggs (Reference Briggs1976). Protocaris marshi is arguably the first soft-bodied Cambrian arthropod described in the literature (Walcott, Reference Walcott1884; see Pari et al., Reference Pari, Briggs and Gaines2021). Simonetta and Delle Cave (Reference Simonetta and Delle Cave1975) assigned P. marshi to a new Order Protocaridida, without a diagnosis. Budd (Reference Budd2008) noted the likely presence in P. marshi of an ‘anterior sclerite’ based on the illustrations in Briggs (Reference Briggs1976), a feature that Budd identified in a diversity of early arthropods, but otherwise P. marshi has received relatively little attention. Legg et al. (Reference Legg, Sutton and Edgecombe2013, fig. 4b) placed it on the stem leading to Euarthropoda. Aria and Caron (Reference Aria and Caron2017) amended Protocarididae to include Branchiocaris, Tokummia, and Loricicaris, noting that the assignment of Protocaris to their newly diagnosed Protocarididae is provisional. Aria and Caron's (Reference Aria and Caron2017) new diagnosis of Protocarididae includes multiple appendage characters, which are not preserved in Protocaris. They assigned Protocarididae to an emended Order Hymenocarina and recovered them as stem mandibulates rather than on the stem of euarthropods.
Remarks
USNM 15400 (Fig. 6.11; see Briggs, Reference Briggs1976, text fig. 3, pl. 1, figs. 1–3), the holotype and only known specimen, was redescribed by Briggs (Reference Briggs1976) who provided a full synonymy. A specimen from the Kinzers Formation of Pennsylvania (USNM 90826, counterpart in the North Museum, Franklin and Marshall College, P-A-392) referred to this taxon by Resser and Howell (Reference Resser and Howell1938), was rejected as unidentifiable (Briggs, Reference Briggs1976).
Class uncertain
Order uncertain
Family uncertain
Genus Vermontcaris new genus
Type species
Vermontcaris montcalmi n. gen. n. sp.
Diagnosis
As for species.
Etymology
After the State of Vermont in which the locality occurs, plus Latin caris for crab.
Remarks
Walcott (Reference Walcott1886) compared the specimens of this taxon available to him to graptolites, referring them to Diplograptus? simplex (see Conway Morris, Reference Conway Morris1993, for a history of early research). Resser and Howell (Reference Resser and Howell1938) assigned the material to a new genus, Emmonsaspis, noting (p. 233) that “the central rod, the ribbing, and the general shape of this animal argue strongly for its reference to the chordates.” Shaw (Reference Shaw1955), in contrast, regarded the affinities of the animal as unknown. Conway Morris (Reference Conway Morris1989, Reference Conway Morris1993) noted that most of the specimens are similar to the Burgess Shale bivalved arthropod Perspicaris Briggs, Reference Briggs1977. He realized that the central rod, presumably interpreted by Resser and Howell (Reference Resser and Howell1938) as a notochord, is the gut and he also observed evidence of a carapace and trunk, and occasional traces of appendages (Conway Morris, Reference Conway Morris1993). All but one of the specimens figured by Resser and Howell (Reference Resser and Howell1938, pl. 9, figs. 2–4) are examples of this arthropod (Fig. 7.1–7.6), which we assign, together with new material (Figs. 9, 10), to Vermontcaris n. gen. because they differ from previously described taxa. The other figured specimen (USNM 15314A: Resser and Howell, Reference Resser and Howell1938, pl. 9, fig. 1) is the lectotype of the chordate Emmonsaspis cambrensis (Walcott, Reference Walcott1890) (Conway Morris, Reference Conway Morris1993; Conway Morris and Caron, Reference Conway Morris and Caron2014) (see Remarks on Emmonsaspis cambrensis).
Vermontcaris montcalmi new species
Figures 7–9
- Reference Walcott1886
Diplograptus? simplex Walcott, part, p. 92, pl. 11, fig. 4 (non fig. 4a).
- Reference Walcott1889
Phyllograptus? simplex Walcott, part, p. 388.
- Reference Walcott1890
Phyllograptus? cambrensis (Walcott), part, p. 604, pl. 59, fig. 3a (non fig. 3).
- Reference Resser and Howell1938
Emmonsaspis cambriensis (Walcott); Resser and Howell, part, p. 233, pl. 9, figs. 2–4 (non fig. 1).
- Reference Conway Morris1989
Arthropod, ?comparable to Perspicaris; Conway Morris, p. 278.
- Reference Conway Morris1993
Arthropod, ?comparable to Perspicaris; Conway Morris, p. 600.
- Reference Pari, Briggs and Gaines2021
New bivalved arthropod; Pari et al., p. 693, fig. 2D, G.
Holotype
YPM IP 543320.
Diagnosis
Carapace with hinge line, valves suboval, eyes pedunculate, borne on a projection of the cephalon, trunk divided into a thorax of up to 40 short segments, similar in length, bearing elongate biramous appendages, and an apodous abdomen of 4 or 5 longer segments increasing in length posteriorly. Short telson tapering to a point, flanked by leaf-like appendages borne by the posteriormost abdominal somite.
Description
The head region is concealed by the carapace. Only a projection bearing the eyes extends beyond the valves. The eyes are borne on stalks attached a short distance from the end of this projection (Figs. 8.3, 8.4, 9.7, 9.8). They are suboval in outline, whether flattened parallel to bedding (Fig. 8.3, 8.4, where they are rotated relative to the carapace) or in lateral aspect (Figs. 8.5, 8.6, 8.8–8.10, 9.9, 9.10), indicating that they were ovoid in shape. None of the specimens preserves evidence of antenniform limbs. Small, poorly defined paired structures, one positive, the other negative in relief, are evident in the anterior part of the carapace of some specimens (Figs. 8.8–8.10, 9.1, 9.2); they may represent the impression of a more heavily cuticularized appendage, perhaps equipped for feeding. Specimens in which the carapace is flipped forward (Figs. 8.5, 8.6, 8.8–8.10, 9.5–9.10) indicate that it was only attached in the head region; no evidence of adductor muscle scars is preserved. The valves are elongate oval, but the preserved outline varies with attitude to bedding (compare Fig. 9.1, 9.2 with 9.3, 9.4).
The bivalved carapace covers most of the trunk, which is divided into two tagmata referred to here as thorax and abdomen. Only the posteriormost segments of the thorax and the abdomen extend beyond the valves (Fig. 8.3, 8.4), except where the carapace is flipped forward. Where the anteriormost part of the thorax is exposed (Figs. 8.8–8.10, 9.7, 9.8) it appears poorly preserved, suggesting that the cuticle normally protected by the carapace was lightly sclerotized and more prone to decay. The thoracic segments bear appendages, whereas the abdomen is apodous. The nature of the preservation does not reveal details of the thoracic appendages, but there is evidence of an elongate branch that tapers distally, terminating in a point. Podomere boundaries are not preserved. This branch, presumably the endopod, extends beyond the continuous outline of the appendages (Figs. 8.5, 8.6, 8.8–8.10, 9.5, 9.6), which probably indicates the extent of overlapping flap-like exopods. Thus, although the detailed nature of the appendages is unknown, they appear to be biramous.
The trunk segment boundaries are fringed by spines, which are occasionally preserved in outline at the trunk margin (Figs. 8.1–8.4, 9.3, 9.4). The boundaries between thoracic segments are usually poorly preserved, particularly at the anterior of the trunk. Where they are evident (Figs. 8.1, 8.2, 9.3–9.8), the posterior thoracic segments appear to be roughly equal in length. The number of segments can be estimated by assuming that there was one pair of appendages per segment, although there may have been more, as in Branchiocaris pretiosa (Resser, Reference Resser1929) (Briggs, Reference Briggs1976). Small relief structures corresponding to thoracic segments (Figs. 8.1, 8.2, 9.5, 9.6) may represent the attachment sites of appendages. The outline of the appendages is never clear, but extrapolation based on their apparent outline in YPM IP 543316 (Fig. 8.3, 8.4) indicates >30, and based on trunk segment divisions, limbs, and attachment sites in YPM IP 239148 (Fig. 9.5, 9.6), >37.
The segments of the abdomen differ from those of the thorax in their greater length and lack of limbs. Identifying the boundary between thorax and abdomen is difficult because there is no abrupt change in the length of segments and the limbs often project posteriorly, overlapping the abdomen. There are at least four, probably five, abdominal segments (Figs. 8.1–8.4, 9.5, 9.6).
The telson is poorly preserved and details are often obscured by superimposition of associated appendages as a result of flattening of the specimens in lateral aspect. A triangular dorsal projection (Fig. 8.3, 8.4), which represents the telson, appears to bear some spines ventrally (Fig. 8.1, 8.2). The anus lies ventral of this projection in the more proximal part of the telson (Fig. 9.7, 9.8). A pair of leaf-like appendages, fringed with fine spines (Fig. 8.1–8.4), appears to articulate with the ventral portion of the pre-telson segment (Fig. 8.1–8.4).
Etymology
In honor of the Montcalm family on whose property the quarry lies.
Material
YPM IP 543320 holotype, USNM 15314D–F, USNM 15314 G (three poorly preserved specimens), YPM IP 239148, YPM IP 543312, YPM IP 543313, YPM IP 543316, YPM IP 543318–543322, YPM IP 543324, YPM IP 543325, YPM IP 543333–543335, YPM IP 239153.
Dimensions
Total length (from the anterior of the carapace to the posterior margin of the telson) is difficult to measure accurately due to the curvature of many specimens. It ranges from ~26 mm (Figs. 8.7–8.9, 9.9, 9.10) to ~40 mm (Figs. 7.5, 7.6, 8.5, 8.6). Different specimens, however, preserve the shortest and longest valve lengths, which range from ~20 mm (Fig. 9.1–9.4) to 28 mm (Fig. 8.1, 8.2). The ratio of carapace length to total length (from anterior of carapace to posterior margin of telson head) ranges from 0.60 (Fig. 8.5, 8.6) to 1.05 (Fig. 9.9, 9.10), emphasizing the effect of flattening. YPM IP 543312 (Fig. 9.3, 9.4) falls within this range at 0.64, but the outline of the valve is anomalous. The height of the valve is 16 mm, substantially greater than in any other specimen, and the height to length ratio is 0.89, much greater than the mean of ~0.5. It is likely that this reflects orientation during flattening. The preserved ratio of valve height to length varies from 0.38 (USNM 15314F and YPM IP 543321) (Figs. 7.5, 7.6, 9.9, 9.10) to 0.89 (YPM IP 543312) (Fig. 9.3, 9.4). Values of H/L: USNM 15314F = 0.38; YPM IP 543312 = 0.89; YPM IP 543316 = 0.47; YPM IP 543319 = 0.48; YPM IP 543320 = 0.41; YPM IP 543321 = 0.38; YPM IP 543333 = 0.43; YPM IP 543334 = 0.43; YPM IP 543335 = 0.53; YPM IP 239148 = 0.48 (Mean 0.49, s 0.15, N = 10; omitting the outlier, YPM IP 543312, mean 0.44, s 0.05, N = 9).
Preservation
We use the terminology introduced by Whittington (Reference Whittington1971) for Burgess Shale specimens to describe variation in the orientation of specimens of Vermontcaris montcalmi n. gen. n. sp. to bedding. Whittington (Reference Whittington1971) distinguished lateral, parallel (dorso-ventrally flattened), and oblique (intermediate) specimens. Vermontcaris montcalmi n. gen. n. sp. is flattened laterally or slightly obliquely (Figs. 7, 8.1–8.6, 8.8–8.10, 9), indicating greater hydrodynamic stability of carcasses in this attitude, presumably because the body was relatively narrow and the valves were not highly convex. YPM IP 239153 (Fig. 8.7), which appears to be flattened dorso-ventrally, as indicated by the narrow carapace and lack of evidence of appendages projecting laterally beyond the trunk, is an exception (a poorly preserved specimen on USNM 15314 G is similar). Specimens show little direct evidence of decay and disarticulation. The carapace is flipped forward in some examples (Figs. 8.5, 8.6, 8.8–8.10, 9.5–9.10) in an attitude that may correspond to the onset of molting (specimens of Canadaspis perfecta [Walcott, Reference Walcott1912] [Walcott, Reference Walcott1912a] from the Burgess Shale show a similar configuration; Briggs, Reference Briggs1978a, p. 475). All the specimens in question preserve traces of the gut along the length of the trunk, however, indicating that they represent carcasses; there is no evidence that they are preserved in the act of molting (cf., García-Bellido and Collins, Reference García-Bellido and Collins2004; Yang et al., Reference Yang, Ortega-Hernández, Drage, Du and Zhang2019). The gut in YPM IP 543334 is preserved in relief in three sections (Fig. 8.8–8.10); the middle of these has rotated, suggesting that the content was authigenically cemented within the carcass. Other specimens preserve the gut in relief (Figs. 7, 8, 9.3–9.10), including examples where it is evident even where it is overlain by the carapace (Figs. 8.1–8.4, 9.3, 9.4), indicating early cementation.
Discussion
The streamlined carapace, flap-like exopods extending beyond the ventral margin of the carapace, and leaf-like appendages associated with the telson suggest that Vermontcaris montcalmi n. gen. n. sp. was a swimmer, as were some other Cambrian bivalved arthropods (Fu and Zhang, Reference Fu and Zhang2011; Legg and Caron, Reference Legg and Caron2014). The flexible trunk (e.g., Fig. 9.5, 9.6) may have aided in locomotion. The multiple segments and resulting large number of exopods may have provided enhanced respiratory capability in response to low-oxygen conditions, such as those in the Franklin Basin (Pari et al., Reference Pari, Briggs and Gaines2021).
Remarks
The morphology of Vermontcaris montcalmi n. gen. n. sp., which is characterized by an elongate body, bivalved carapace, and multiple short trunk segments, aligns with that of Cambrian stem-group arthropods such as Jugatacaris (Fu and Zhang, Reference Fu and Zhang2011) and Pectocaris (Hou, Reference Hou1999; Hou et al., Reference Hou, Bergström and Xu2004; Jin et al., Reference Jin, Mai, Chen, Liu, Hou, Wen and Zhai2021). The number of thoracic segments in V. montcalmi n. gen. n. sp. is difficult to determine, but there may be as many as 40. There is a short abdomen of 4 or 5 segments without appendages. The carapace of Jugatacaris agilis from the Chengjiang biota has a dorsal keel or blade-like structure, in contrast to that of V. montcalmi n. gen. n. sp. The trunk comprises 55–65 segments, including 3–5 apodous segments at the posterior end (Fu and Zhang, Reference Fu and Zhang2011). Fu and Zhang (Reference Fu and Zhang2011, p. 574) did not designate these posteriormost segments as a separate abdomen, acknowledging the difficulty of drawing a distinction between it and the rest of the trunk. The trunks of Pectocaris spatiosa, P. eurypetala (Hou and Sun, Reference Hou and Sun1988), and P. inopinata Jin et al., Reference Jin, Mai, Chen, Liu, Hou, Wen and Zhai2021, have ~50 segments, but vary in the number that lack appendages: the first two have ~4, whereas the last, in contrast to V. montcalmi n. gen. n. sp., has 11 or 12 making up a distinct abdomen. The telson of P. spatiosa Hou, Reference Hou1999, is unknown (Hou, Reference Hou1999). The telson of P. inopinata bears a pair of lateral appendages distally, whereas P. eurypetala has a median as well as lateral flukes (Hou et al., Reference Hou, Bergström and Xu2004), similar to the arrangement in Odaraia alata Walcott, Reference Walcott1912 (Walcott, Reference Walcott1912a). The lateral appendages in V. montcalmi n. gen. n. sp., in contrast, appear to articulate with the pretelson segment. Phylogenetic analyses (Legg et al., Reference Legg, Sutton, Edgecombe and Caron2012; Legg and Caron, Reference Legg and Caron2014) resolved Pectocaris and Jugatacaris within a paraphyletic grade of bivalved forms, which are part of ‘Upper’ stem-Euarthropoda at the base of the arthropod stem (Ortega-Hernández, Reference Ortega-Hernández2016). Bivalved forms with multisegmented elongate bodies likely represent a symplesiomorphic morphology that consistently falls within this clade (J. Yang et al., Reference Yang, Ortega-Hernández, Lan, Hou and Zhang2016). Aria and Caron (Reference Aria and Caron2017) omitted Jugatacaris from their analysis but regarded it as a putative member of the hymenocarines, which resolved as stem mandibulates (Vannier et al., Reference Vannier, Aria, Taylor and Caron2018, obtained hymenocarines as crown mandibulates). These several alternative placements might apply equally to V. montcalmi n. gen. n. sp., but the lack of preserved details of the appendages prevent its position from being resolved further.
?Phylum Echinodermata/Phylum Hemichordata stem group
?Unranked Clade Cambroernida Caron, Conway Morris, and Shu, Reference Caron, Conway Morris and Shu2010
?Herpetogaster Caron, Conway Morris, and Shu, Reference Caron, Conway Morris and Shu2010
Type species
Herpetogaster collinsi Caron, Conway Morris, and Shu, Reference Caron, Conway Morris and Shu2010, Burgess Shale and Stephen Shale formations, Yoho and Kootenay National Parks, British Columbia, Canada.
?Herpetogaster sp.
Figure 10.1, 10.2
- Reference Pari, Briggs and Gaines2021
Herpetogaster?; Pari et al., p. 693, fig. 2H.
Description
YPM IP 239054 (Fig. 10.1, 10.2) is curved sharply through an angle of ~30°. The shorter, wider portion terminates in an array of tentacle-like structures, which are too poorly preserved to reveal details, and presumably represents the head. The bend coincides with a wide area of gut fill that extends to the outer convex margin and may represent the stomach. There is no unequivocal trace of the gut along the length of the trunk in either direction beyond this fill, and therefore no evidence of the position of the mouth or anus. The longer portion of the trunk ends in a blunt round, presumably posterior, termination. Wrinkles parallel to the margin near this posterior end indicate compaction of relief. The margins of the trunk are not clearly defined everywhere, and there is no evidence of segmentation. The specimen is ~80 mm long.
Material
YPM IP 239054.
Discussion
Although poorly preserved, the Parker Formation specimen preserves characters that align with Herpetogaster, including the worm-like body, prominent gut trace (possibly the stomach), and array of possible tentacles (Pari et al., Reference Pari, Briggs and Gaines2021). The compaction wrinkles are consistent with a tough, flexible integument (Caron et al., Reference Caron, Conway Morris and Shu2010, p. 4). The Parker Formation specimen differs from the lower Cambrian genus Phlogites from the Chengjiang Lagerstätte of China, to which Herpetogaster has been compared (Caron et al., Reference Caron, Conway Morris and Shu2010), in lacking a long stolon continuous with the trunk, although such a structure might be concealed by matrix. It is somewhat larger than previously reported specimens of Herpetogaster from other localities.
Caron et al. (Reference Caron, Conway Morris and Shu2010) and Kimmig et al. (Reference Kimmig, Meyer and Lieberman2019) provided comprehensive discussions of the possible deuterostome affinity of Herpetogaster. Caron et al. (Reference Caron, Conway Morris and Shu2010) assigned it to an unranked stem group, which they named cambroernids (without a formal cladistic analysis) lying somewhere on the branch leading to Echinodermata and/or Hemichordata; Kimmig et al. (Reference Kimmig, Meyer and Lieberman2019) followed their lead. The Parker Formation specimen potentially extends the geographic range of the genus to the opposite margin of the paleocontinent Laurentia.
Remarks
We tentatively assign the single specimen, YPM IP 239054, to Herpetogaster, a genus that was first described by Caron et al. (Reference Caron, Conway Morris and Shu2010) on the basis of remarkably preserved material from the Burgess Shale and Stephen Shale formations (Cambrian Miaolingian, Wuliuan). The type species, H. collinsi Caron, Conway Morris, and Shu, Reference Caron, Conway Morris and Shu2010, is a soft-bodied animal with a tentaculate feeding apparatus and worm-like body, which was attached to the substrate by a stalk or stolon. Herpetogaster collinsi also has been reported from the Ruin Wash Lagerstätte (Cambrian Series 2, Stage 4) in the Pioche Formation of Nevada (Kimmig et al., Reference Kimmig, Meyer and Lieberman2019), which is similar in age to the Parker Formation. The apparent arrangement of tentacles in the Parker Formation specimen is very different from that in H. collinsi, but more similar to that in a second species, H. haiyanensis Yang et al., Reference Yang, Kimmig, Lieberman and Peng2020, from the Chengjiang biota (Yang et al., Reference Yang, Kimmig, Lieberman and Peng2020). Confident assignment of the Parker Formation taxon will require discovery of additional specimens.
Phylum Chordata
Subphylum Vertebrata, stem group
Genus Emmonsaspis Walcott, Reference Walcott1890
Type species
Emmonsaspis cambrensis (Walcott, Reference Walcott1890), Parker Formation, Parker Quarry, Franklin County, Vermont, USA.
Emmonsaspis cambrensis (Walcott, Reference Walcott1890)
Figure 10.3–10.5
- Reference Walcott1886
Diplograptus ? simplex Walcott, part, p. 92, pl. 11, fig. 4a (non fig. 4).
- Reference Walcott1889
Phyllograptus? simplex Walcott, part, p. 388.
- Reference Walcott1890
Phyllograptus? cambrensis (Walcott), part, p. 604, pl. 59, fig. 3 (non fig. 3a).
- Reference Resser and Howell1938
Emmonsaspis cambriensis (Walcott); Resser and Howell, part, p. 233, pl. 9, fig. 1 (non figs. 2–4).
- Reference Conway Morris1993
Emmonsaspis cambrensis (Walcott, Reference Walcott1890); Conway Morris, p. 599, pl. 1, figs. 1, 2.
- Reference Conway Morris and Caron2014
Metaspriggina sp.; Conway Morris and Caron, Extended data fig. 6a–d.
- Reference Pari, Briggs and Gaines2021
Metaspriggina; Pari et al., Reference Pari, Briggs and Gaines2021, p. 693, fig. 2E.
Holotype
USNM 15314A, Parker Formation, Parker Quarry, Franklin County, Vermont, USA. Cambrian Series 2, Stage 4 (Resser and Howell, Reference Resser and Howell1938, pl. 9, fig,1).
Description
The original specimens are incomplete, but USNM 15314A, which is the largest, preserves ~50 myomeres (Conway Morris and Caron, Reference Conway Morris and Caron2014, Extended data fig. 6b). The eye is evident in USNM 15314C. USNM 15314A preserves dark ventral features (interpreted by Conway Morris and Caron, Reference Conway Morris and Caron2014, Extended data fig. 6b, as the heart and liver). YPM IP 239155 preserves evidence of the eyes (Fig. 10.3, 10.4) and even a distinct central area (interpreted as the eye lens by Conway Morris and Caron, Reference Conway Morris and Caron2014, fig. 1). YPM IP 239155 preserves evidence of ~50 myomeres (Fig. 10.3), but the distal extremity is not evident. A dark area (Fig. 10.3) is preserved in a similar position to that interpreted as the liver by Conway Morris and Caron (Reference Conway Morris and Caron2014). YPM IP 543315 (Fig. 10.5) is unusual in being preserved as a rust-colored coating, iron oxide presumably after pyrite, that obscures finer details. USNM 15314A was at least 40 mm long and 10 mm high. YPM IP 543315 (Fig. 10.5) was at least 45 mm long. YPM IP 239156 and YPM IP 239155 (Fig. 10.3, 10.4) were at least 35 and 40 mm long, respectively.
Material
USNM 15314A, holotype, USNM 15314B, C, YPM IP 239154-239156, YPM IP 543315, YPM IP 543323.
Discussion
Conway Morris and Caron (Reference Conway Morris and Caron2014) referred the original Parker Formation specimens (well illustrated in their Extended data fig. 6) to Metaspriggina spp. without comment. Metaspriggina was not named until 1993, when Simonetta and Insom erected a new genus and species from the Burgess Shale, M. walcotti Simonetta and Insom, Reference Simonetta and Insom1993, which was subsequently shown to be a chordate (Conway Morris, Reference Conway Morris2008). We retain Emmonsaspis as the generic name for the Parker Formation taxon, noting that it has priority. Conway Morris and Caron (Reference Conway Morris and Caron2014, p. 419) observed that the Parker Formation specimens differ in being less slender and having myomeres with a more angular closure; they also lack evidence of gill bars and zig-zag shaped myomeres. These differences, unless taphonomic, might justify assignment to a separate genus. Otherwise Metaspriggina is a junior synonym of Emmonsaspis.
Remarks
Resser and Howell (Reference Resser and Howell1938) erected Emmonsaspis to accommodate three specimens of this taxon and six specimens that we assign herein to the new taxon, Vermontcaris montcalmi n. gen. n. sp., all from the Parker Quarry Lagerstätte, noting (Reference Resser and Howell1938, p. 233) that the morphology “of this animal argue[s] strongly for its reference to the chordates.” They designated all specimens under USNM 15314 as cotypes. Conway Morris (Reference Conway Morris1993) initially considered Emmonsaspis cambrensis to be a frond-like form similar to some Ediacaran animals. Conway Morris (Reference Conway Morris1993, p. 599) provided a full synonymy of the taxon observing that only the first specimen figured by Resser and Howell (Reference Resser and Howell1938, pl. 9, fig. 1), USNM 15314A, is attributable to E. cambrensis (Walcott, Reference Walcott1886, pl. 11, fig. 4a; Reference Walcott1890, pl. 59. fig. 3; Conway Morris, Reference Conway Morris1993, pl. 1, fig. 1). He also noted that the other specimens represent a bivalved arthropod (here described as V. montcalmi n. gen. n. sp.). He therefore designated USNM 15314A as holotype (Conway Morris Reference Conway Morris1993, p. 600) (i.e., lectotype; ICZN Article 74.1), noting its association with two other less well-preserved specimens (USNM 15314B, C). Conway Morris and Caron (Reference Conway Morris and Caron2014, Extended Data fig. 6) reinterpreted E. cambrensis from the Parker Formation as a chordate as part of their redescription of the chordate Metaspriggina walcotti Simonetta and Insom, Reference Simonetta and Insom1993, from the Burgess Shale (Conway Morris, Reference Conway Morris2008), based on newly discovered material from the Walcott Quarry and Marble Canyon, assigning the Parker Formation chordate specimens to Metaspriggina sp.
Discussion and conclusions
Our excavations at Parker's Cobble have yielded a number of new soft-bodied taxa from the locality (Pari et al., Reference Pari, Briggs and Gaines2021), including Fuxianospora, a ?priapulid, a radiodont, and a possible Herpetogaster, as well as multiple specimens of known taxa, particularly the new bivalved arthropod described here as Vermontcaris montcalmi n. gen. n. sp. The only other significant Lagerstätten of Cambrian Series 2, Stage 4 age on the southern margin of Laurentia occur in the more widely known Kinzers Formation of Pennsylvania (Muscente et al., Reference Muscente, Schiffbauer, Broce, Laflamme and O'Donnell2017; Pari et al., Reference Pari, Briggs and Gaines2021). The Kinzers Formation is also dominated by trilobites, but Olenellus crassimarginatus Walcott, Reference Walcott1910, is one of very few that are common to both it and the Parker Formation (Webster and Landing, Reference Webster and Landing2016). Non-biomineralized taxa that occur in both the Kinzers and Parker formations include algae (Skinner, Reference Skinner2005), sponges (Rigby, Reference Rigby1987), possible priapulids (Conway Morris, Reference Conway Morris1977), radiodonts (Pates and Daley, Reference Pates and Daley2019), Tuzoia and other bivalved arthropods (Skinner, Reference Skinner2005), and Emmonsaspis (Conway Morris and Caron, Reference Conway Morris and Caron2014). The Kinzers fauna is much more diverse, however, reflecting a greater variety of sedimentary facies, a much greater abundance of fossils, both soft-bodied and shelly (e.g., echinoderms), and extensive collecting over many years (Thomas, Reference Thomas2021). The Parker Formation also shares taxa with the similarly aged Comet Shale Member of the Pioche Formation of Nevada (Lieberman, Reference Lieberman2003), which is on the northern margin of Laurentia, also a muddy bottom setting. The rarity of soft-bodied fossils from the Parker Formation limits meaningful comparisons, an issue that may be resolved by more collecting at the site.
Acknowledgments
We are grateful to G. Montcalm and family for permission to excavate on their land. J. Skabelund participated in the first of recent digs. S. Butts and J. Utrup (Yale Peabody Museum) provided collections support and they, and L.A. Parry, assisted with photography. M. Florence and D.H. Erwin facilitated the loan of material from the U.S. National Museum, Smithsonian Institution. Z. Jiang, Department of Earth and Planetary Sciences, Yale University, assisted with SEM and EDS analyses, and S. Lackie, Great Lakes Institute for Environmental Research, University of Windsor, Ontario, Canada, provided the EDS maps. J.P. Botting, S. Pates and R.D.K. Thomas provided detailed comments on the submitted paper which significantly improved the final version.