Introduction
Terrestrial Antarctica contains many of the most pristine environments on Earth. It is governed internationally by the Antarctic Treaty System and, through the Protocol on Environmental Protection to the Antarctic Treaty (hereafter the Protocol) (ATCM, 1991), aims to provide for the comprehensive protection of the Antarctic environment. However, human presence continues to increase on the continent and impact the environment, with effects ranging from trampling of soils (Tejedo et al., Reference Tejedo, Benayas, Cajiao, Albertos, Lara, Pertierra and Reck2016) to contamination by fuels and trace metals (Bargagli, Reference Bargagli2008; Guerra, Neto, Prianti, Pereira-Filho, & Schaefer, Reference Guerra, Neto, Prianti, Pereira-Filho and Schaefer2013) and introduction of non-native taxa (Chown et al., Reference Chown, Huiskes, Gremmen, Lee, Terauds, Crosbie and Bergstrom2012; Hughes, Pertierra, Molina-Montenegro, & Convey, Reference Hughes, Pertierra, Molina-Montenegro and Convey2015). Human activity is also disproportionately concentrated in the relatively few ice-free areas of the continent, which are shared with the bulk of terrestrial biota (Brooks, Jabour, van den Hoff & Bergstrom, Reference Brooks, Jabour, van den Hoff and Bergstrom2019). Indeed, these authors found that 76% of all man-made structures are found in ice-free areas within 5 km of the coast, a zone that occupies <0.1% of the continent. Despite the intentions of the Protocol, which entered into force in 1998, human presence has left its mark (Brooks et al., Reference Brooks, Jabour, van den Hoff and Bergstrom2019; Deprez, Arens & Locher, Reference Deprez, Arens and Locher1999; Hodgson-Johnson, Jackson, Jabour & Press, Reference Hodgson-Johnston, Jackson, Jabour and Press2016, Klein et al., Reference Klein, Snape, Schaefer, Aislabie, Delille and De Azevedo Jurelevicius2018; Stark et al., Reference Stark, Johnstone, King, Raymond, Rutter, Stark and Townsend2023).
While the Protocol now seeks to provide comprehensive protection, prior to its adoption, a 1978 Annex to the Treaty, the “Code of Conduct for Antarctic Expeditions and Station Activities” (ATCM, 1978) required less stringent procedures for managing waste disposal, non-native taxa, interactions with breeding birds and mammals and general operational activities. The 1978 Annex allowed disposal of materials through burning and dumping at sea at coastal stations, while inland sites were advised to “concentrate all waste in deep pits.” Inevitably, legacy contamination of sites occupied prior to the Protocol is widespread (e.g. McIvor, Reference McIvor2014; Waterhouse, Reference Waterhouse, Lyons, Howard-Williams and Hawes1997). Recognising the extent of contaminated sites, the subsequent Article 1.5 of Annex III to the Protocol (Waste Disposal and Waste Management) specifically directs that, “Past and present waste disposal sites on land and abandoned work sites of Antarctic activities shall be cleaned up by the generator of such wastes and the user of such sites.” However, Article 1.5 further points to the instance “where the removal by any practical option would result in greater adverse environmental impact than leaving the structure of waste material in its existing location,” then a clean-up may not be required.
The nature of contaminants associated with Antarctic facilities was outlined in a review by Tin et al. (Reference Tin, Fleming, Hughes, Ainley, Convey, Moreno and Snape2009) and included chemicals such as hydrocarbons from fuel spills, trace metals/metalloids, polychlorinated biphenyls (PCBs), polybrominated diphenyl ether flame retardants and persistent contaminants, such as polycyclic aromatic hydrocarbons (PAH) and polychlorinated dibenzodioxins. The Committee for Environmental Protection (CEP), established under the Protocol to provide advice and recommendations to Antarctic Treaty Parties on the implementation of the Protocol, developed a manual to guide the clean-up of contaminated locations (ATCM, 2019) (CEP). Active clean-up is particularly important in Antarctica, as in the arid, cold conditions that prevail over much of the continent, natural remediation and flushing are at best slow. For example, while native hydrocarbon-degrading bacteria occur in Antarctic soils and respond to spills through increased population size of suitable bacteria (Gran-Scheuch, Ramos-Zuñiga, Fuentes, Bravo, & Pérez-Donoso, Reference Gran-Scheuch, Ramos-Zuñiga, Fuentes, Bravo and Pérez-Donoso2020), the short, cold summer season limits in situ bioremediation potential (Aislabie, Balks, Foght, & Waterhouse, Reference Aislabie, Balks, Foght and Waterhouse2004). Although de Jesus, Peixoto and Rosado (Reference de Jesus, Piexoto and Rosado2015) and Woodberry, Stevens, Snape and Stark (Reference Woodberry, Stevens, Snape and Stark2005) suggest strategies by which bioremediation may be improved in Antarctic soils, Annex II to the Protocol itself limits the unrestrained use of chemically or microbially enhanced remediation techniques that would see new contaminants or exotic micro-organisms introduced.
New Zealand is an original signatory to the Antarctic Treaty and has assumed responsibility for the rehabilitation of several legacy contaminated sites. Substantial remediation efforts have been undertaken at a severely contaminated abandoned coastal site shared with the United States Antarctic Programme at Cape Hallet in Northern Victoria Land (Carson, Reference Carson2008; NSF, 1993; USAP, 1994). This station was built within a penguin colony and displaced birds from historic breeding areas. Its remediation primarily focussed on creating conditions for the re-establishment of penguin breeding. Recovery of the Cape Hallet colony was recently re-evaluated (Kim, Kim, Younggeun, Kim, & Kim, Reference Kim, Kim, Younggeun, Kim and Kim2023), and the goal of penguin population recovery was found to be incomplete but still ongoing. The critical need to evaluate the success of site rehabilitation by post-action monitoring of ecological functions emerged from the Cape Hallett example as a crucial evaluation element that was not implemented by the rehabilitation team.
A review of past remediation, particularly in light of recent developments in remediation science and planning, and potential impacts of climate change provides insights into how best to define future efforts. This contribution focusses on the rehabilitation of another New Zealand outpost, Vanda Station on the shores of endorheic (closed basin) Lake Vanda. This facility was built in the late 1960s and operated for over two decades under guidelines pertaining prior to the Protocol taking effect. It was removed when rising lake levels threatened to flood the station site (Waterhouse, Reference Waterhouse, Lyons, Howard-Williams and Hawes1997). While this threat, resulting from persistent high flows of meltwater into the lake, precipitated the action, it has been suggested (Howkins, Chignell, & Fountain, Reference Howkins, Chignell and Fountain2021) that the closure coincided with a number of interconnected social, political, scientific and environmental factors of the time and that “the rising lake levels provided a convenient justification for the closure of Vanda Station, as the political status quo sought to adapt to the implications of the changing environment.” Following the removal of the buildings, vigorous attempts were made to rehabilitate the site to minimise the impact on the unique lake, as the level was expected to continue to rise and inundate the station site with obvious risks of contamination. In this case, monitoring continued for some time after decommissioning to allow the effectiveness of the remediation to be assessed.
Here we outline the decommissioning process at Vanda Station and review the efforts made to rehabilitate the site, from the assessment of the state, the setting of remediation targets, the development of a remediation plan and monitoring to determine whether those targets were achieved. This remediation required a series of value judgements to be made, and these were made ad hoc, without recourse to a formal framework. Post-remediation monitoring showed that, even after the site was flooded by rising lake waters, there was no detectable impact on the chemistry of overlying lake water.
To assist in guiding future clean-up operations in Antarctica, we evaluate the process followed in the Vanda Station rehabilitation with reference to two formal frameworks developed since then, the Net Environmental Benefit Analysis (NEBA) (Efroymson, Nicolette & Suter, Reference Efroymson, Nicolette and Suter2004) developed for remediation of more temperate regions and the current guidance provided in the Antarctic clean-up Manual (ATCM, 2019). Our aim here is to review the decision-making process at Vanda Station in the light of these frameworks to identify barriers to restoration and lessons learned for future facility decommissioning in Antarctica.
Background to Vanda Station
The McMurdo Dry Valleys were first described by members of Scott’s 1901–04 National Antarctic Expedition, who stumbled on the strange, ice-free valleys when they lost their way in poor visibility returning from the polar plateau. A more comprehensive investigation followed during the 1910–13 British Antarctic Expedition when a team led by geologist Griffith Taylor travelled the length of what became known as the Taylor Valley. Later aerial reconnaissance revealed the full extent of the McMurdo Dry Valleys, which are now recognised as the largest continuous area of ice-free land in Antarctica (Levy, Reference Levy2013). The region, with its arid landscape dotted with streams, ponds and lakes, has been the site of intensive scientific research since 1956. Of particular interest to New Zealand researchers has been the Wright Valley, with the centre of operations at New Zealand’s Vanda Station on the shores of the large (∼6 km2), deep (∼75 m) Lake Vanda (Figs. 1 and 2). A “social history” of Vanda Station is provided by Howkins et al. (Reference Howkins, Chignell and Fountain2021).

Figure 1. Location of Lake Vanda in the Wright Valley, Southern Victoria Land, Antarctica. The position of the Wright Valley is indicated by the red dot in the inset map and of Vanda Station, enlarged as Figure 2, by the red box in the main map.
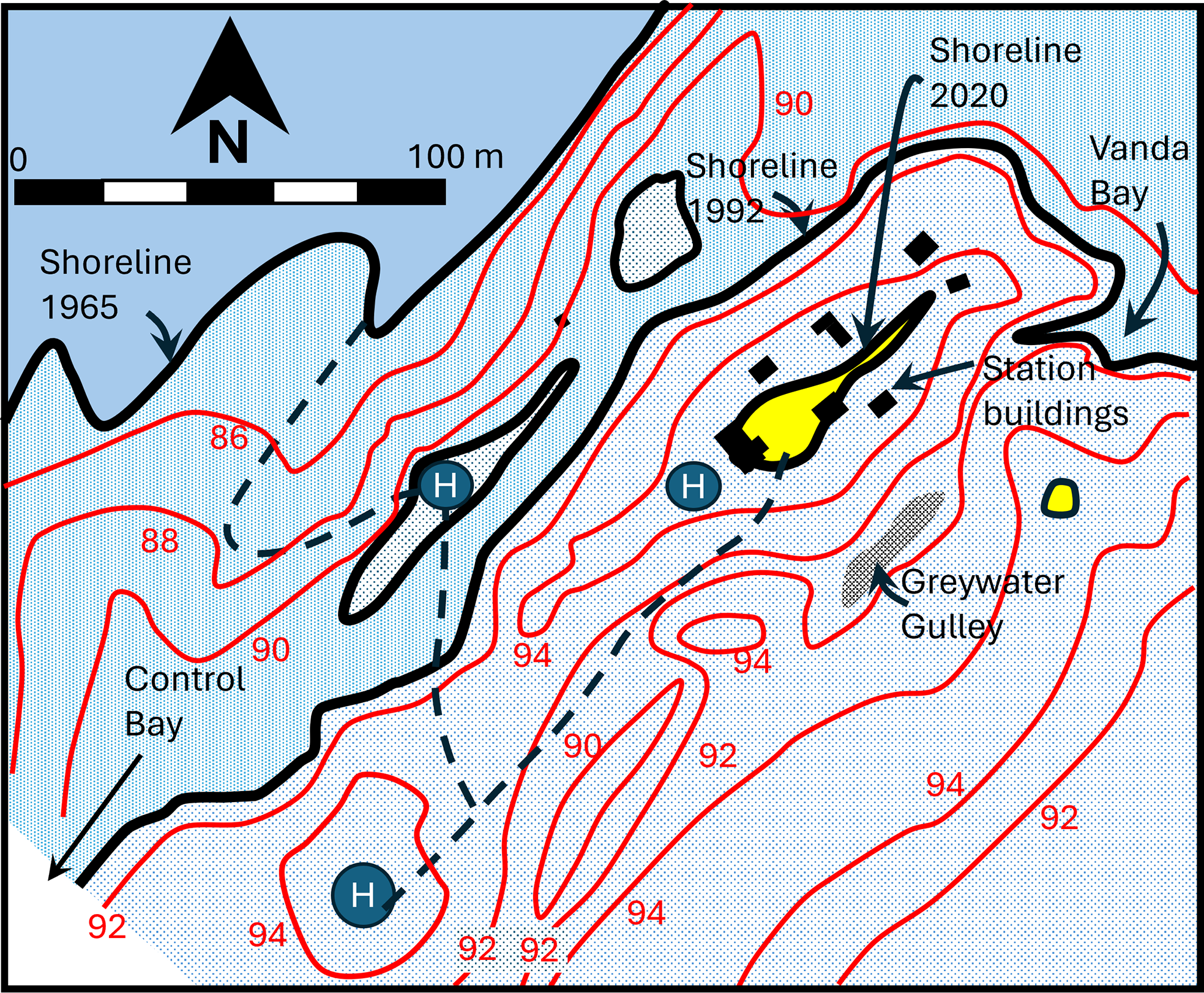
Figure 2. Sketch map of Vanda Station site on the eastern end of Lake Vanda. Contours (red) are at 2 m intervals of elevation above mean sea level, derived from an unpublished topographic survey in 1965. Shorelines in 1992 and 2020 are derived from Sheppard, Campbell and Claridge (Reference Sheppard, Campbell and Claridge1993) and Google Earth imagery, respectively. “H” indicates a helicopter landing pad, and dashed lines represent vehicle tracks. The blue shaded area is the lake surface in 1965, and the yellow-filled areas are the land remaining above lake level in 2020.
Vanda Station was constructed in the 1968–69 summer on a ridge over 200 m from, and 15 m above, the shoreline of Lake Vanda at the time (Figs. 3 and 4). It consisted of eight buildings and could accommodate up to 12 people. At its maximum extent, the buildings comprised a mess hut with kitchen facilities, a radio room, a storeroom, three sleeping huts, a workshop, a generator house, a laboratory and a “toilet” consisting of a shelter over a removable drum for solid toilet waste and a urinal leading to removable waste containers. Close to the station were a climate station, a wind turbine and two primary helicopter landing sites. A “road” had been smoothed between the station and the helicopter landing sites. A third helicopter landing area, used rarely, was situated close to the mess building. The station had a tractor with a trailer for transporting goods between it and the main helicopter landing pads. The tractor had a front-end loader attached for collecting ice from the surface of Lake Vanda for the significant ice melter outside the mess building.
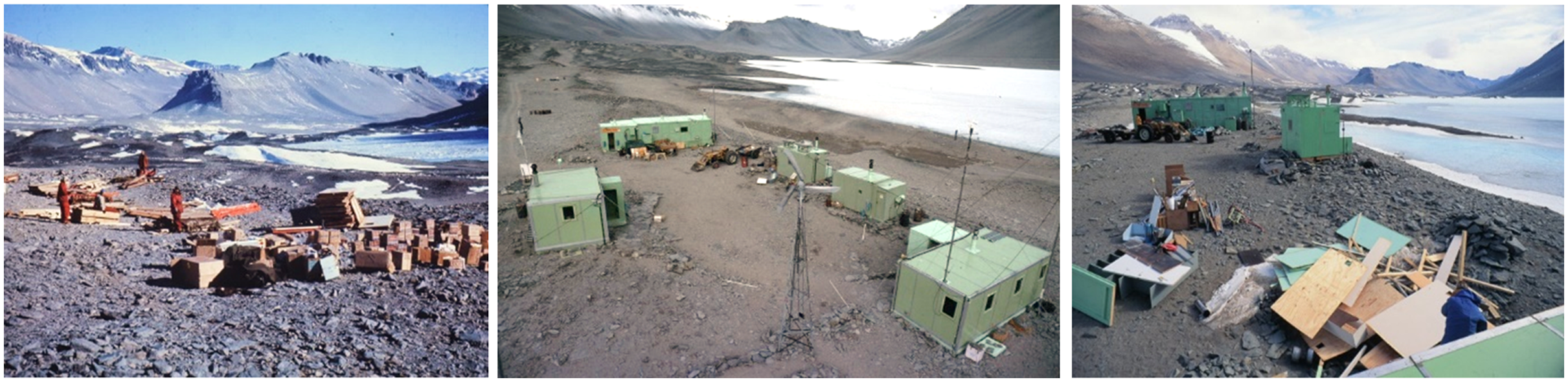
Figure 3. Left – Vanda Station under construction in 1968. Centre – Vanda Station in operation. Right – deconstruction underway in 1993. Note the gradual encroachment of the lake up to the station site (Images Antarctica New Zealand; A.D.A.M.).

Figure 4. Vanda Station in the 1970s (top), at the time of removal in 1993 (centre) and the flooded site in 2023 (yellow boxes mark the same footprint of the station in all images).
Fuel for the station was low-temperature diesel for the generators and tractor and kerosene for the kitchen. Fuel was stored in a large tank adjacent to the main mess hut, into which it was pumped by hand from drums. There was no physical containment of the fuel storage areas.
The station was occupied every summer and for three winters (1969–70 and 1974) with a permanent staff of four, supporting a variable number each year of scientists, surveyors, maintenance staff, aircraft crews and VIPs. The numbers of person-days by Vanda Station staff alone amounted to 13,980 days (9,600 days in normal years plus 4,380 days in the overwintering years). Figures compiled by Andrew (Reference Andrew2010) suggest that in addition, between the years 1968 and its decommissioning in 1992 ca. 2750 science event person-days were spent at the base giving a total of 16,730 person-days (45.8 person-years) over the life of the station, a substantial human footprint for a polar desert site. While the majority of staff at the station directly supported New Zealand science activities, the station also hosted expeditions from the Japanese Antarctic Research Expedition in the 1970s and 1980s (e.g. Hayward, McFarlane, Keys, & Campbell, Reference Hayward, McFarlane, Keys and Campbell1994, Matsumoto Torii & Hanya, Reference Matsumoto, Torii and Hanya1984, Takamatsu Kato, Matsumoto & Torii, Reference Takamatsu, Kato, Matsumoto and Torii1998) and individual scientists from a range of nations who collaborated with New Zealand researchers from the station (Howkins et al., Reference Howkins, Chignell and Fountain2021).
The final year of occupation of Vanda Station was the 1991–92 summer, following which were two years of deconstruction when a number of personnel stayed on the site. From 1992, research activities in the Wright Valley were moved to a much smaller, un-staffed facility, comprising three relocatable, prefabricated huts near the Lake Vanda inflow, the Onyx River, providing two laboratory spaces and a cooking and eating space. Researchers stay in tents adjacent to that facility.
Impacts of station operations
As outlined in Waterhouse (Reference Waterhouse1993), by 1992 the 23-year occupation of Vanda Station had resulted in considerable impacts at the site. Construction in 1968–69 had included excavations and site clearing and levelling for the buildings, construction of the track to the two main helicopter pads and the pads themselves (Fig. 2). Rocks marking the route to and around these pads and other key locations were painted with lead-based orange paint that was later found in the adjacent soils (Sheppard et al., Reference Sheppard, Campbell and Claridge1993). Cumulative impacts included trampling and vehicle movements at the station, waste disposal, soot from the exhaust of the station’s generator, erosion of paint from buildings and occasional loss of small objects from the mess and workshop that dropped into the soil (Waterhouse, Reference Waterhouse, Lyons, Howard-Williams and Hawes1997).
Waste disposal procedures evolved over the lifetime of the station, as environmental awareness became more important for Antarctic activities. Some burning of solid wastes occurred during the early years of occupation. All solid and toilet waste was removed from the Wright Valley from 1970 onwards, but in the first two years of operation, liquid wastes were disposed of on land. One site, known colloquially as “Greywater Gully” (Fig. 2), initially received most liquid wastes, including urine, used battery acid, domestic washing wastewater and used photo-chemicals (Waterhouse, Reference Waterhouse1993). From 1972, all liquid waste except for strained greywater from the kitchen and washing was collected into drums and returned to Scott Base for disposal. Greywater continued to be disposed of in Greywater Gully until 1992 (Waterhouse, Reference Waterhouse, Lyons, Howard-Williams and Hawes1997).
During the remediation, it was found that liquid disposed of in Greywater Gully appeared to have accumulated in the soil profile, presumably constrained by an ice-cemented aquitard. The bases of soil pits dug to the permafrost in the gully filled with water tens of cm thick, which sat at a level higher than the lake itself, suggesting that there was some barrier to direct drainage into the lake. Throughout, we term this accumulated fluid “groundwater,” and it was visibly contaminated with bathing and kitchen wastes. The typical presentation of Wright Valley groundwater was first described by Wilson (Reference Wilson1979) as a thin film of brine flowing along the upper surface of the ice-cemented permafrost during the warmer months, which he termed a supra-permafrost layer (SP). We suspect that the water in Greywater Gully was a mix of naturally generated SPF and accumulated wastewater, but no similar control gullies were examined, and it was not possible to evaluate the contributions of the potential sources. Fuel management during the lifetime of Vanda Station was poor relative to current operating practices, with no use of fuel bunds or other spill containment. As a result, there were frequent spills directly on to soil during transfer or through leakages from drums in storage, and cumulative hydrocarbon contamination of soils was extensive (Webster, Webster, Nelson & Waterhouse, Reference Webster, Webster, Nelson and Waterhouse2003). Hydraulic oils jettisoned from helicopters at landing, as well as residual material from smoke bombs used for helicopter approaches were associated with the helicopter pad sites.
The Initial Environmental Evaluation (IEE) for the decommissioning of the station (Hayward et al., Reference Hayward, McFarlane, Keys and Campbell1994) provided details on the specific locations around the station of known potential sites of significant contamination.
Rising lake levels
Like most of the large lakes of the McMurdo Dry Valleys, Lake Vanda occupies a closed basin (it has an inflow but no outflow). Long-term changes to the balance between water entering the lake from the inflows and leaving via ablation and evaporation results in changes to lake levels over time (Chinn & Mason, Reference Chinn and Mason2015), and Lake Vanda has proved particularly vulnerable to level change (Castendyk, Obryk, Leidman, Gooseff, & Hawes, Reference Castendyk, Obryk, Leidman, Gooseff and Hawes2016). In the 22 years between the year of establishment and 1991, the lake had risen by 12.5 m (Figs. 4 and 5), threatening to flood the lower-lying parts of Vanda Station. The sequence of events is summarised in Hawes et al. (Reference Hawes, Howard-Williams, Gilbert, Hughes, Convey and Quesada2023) and illustrated in Figure 5.
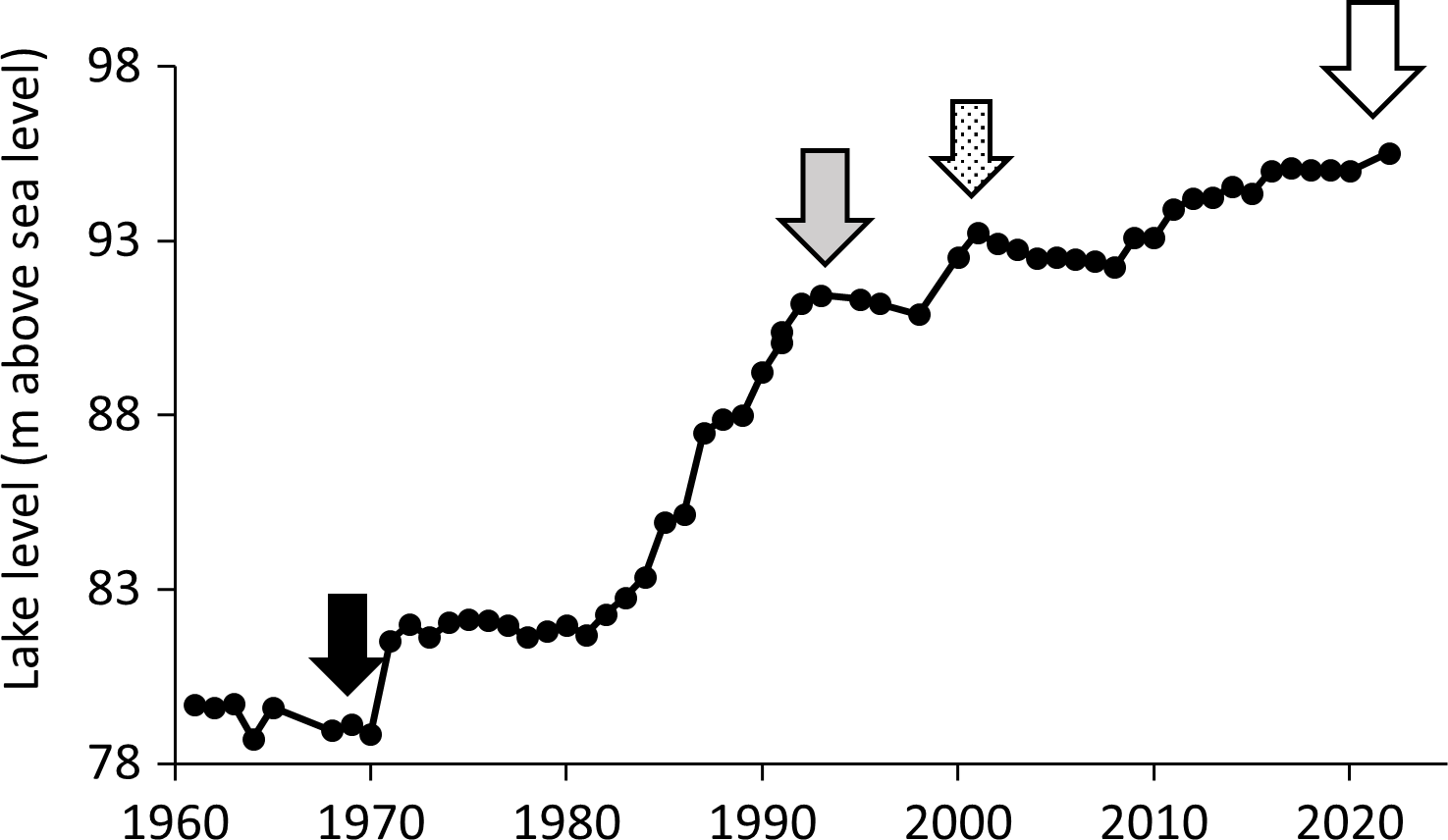
Figure 5. Water level of Lake Vanda from 1961 to 2022. The black arrow indicates the construction of Vanda Station, the grey arrow its decommissioning and the dotted arrow indicates the time at which “Greywater Gully” became connected with the main lake. The white arrow shows the point at which the entire station site was underwater (see Figs. 2 and 4).
Two features in Figure 5 of relevance to the decommissioning process are:
-
1. The rapid rise of lake level over the 10-year period prior to 1991, which created a sense of urgency due to the apparent imminent inundation of the site; and
-
2. The periods of lake level stabilisation or decline between 1993 (station decommissioning) and 1999 and then from 2001 to 2009, which impacted the planned post-inundation monitoring.
Decommissioning the station
With the prospect of imminent inundation, the New Zealand Antarctic Programme (NZAP) decided in 1991 to decommission and remove the station, and the scale of the task was assessed. Importantly, in 1991, the Environmental Protocol to the Treaty (hereafter the Protocol) was agreed upon and signed by New Zealand, though it would not enter into force internationally until 1998. New Zealand implemented the Protocol domestically in 1994 as the Antarctica (Environmental Protection) Act, but prior to that, it was determined in 1991 that the decommissioning of the station should be compliant with the Protocol. Key issues that this raised were that:
-
1. Article 2 of Annex I to the Protocol required an IEE for the decommissioning, containing, “sufficient detail to assess whether a proposed activity may have more than a minor or transitory impact and shall include:
-
a. a description of the proposed activity, including its purpose, location, duration and intensity; and
-
b. consideration of alternatives to the proposed activity and any impacts that the activity may have, including consideration of cumulative impacts in the light of existing and known planned activities.”
-
-
2. Article 1(5) of Annex III to the Protocol (Waste Disposal and Waste Management) specifically directs that “Past and present waste disposal sites on land and abandoned work sites of Antarctic activities shall be cleaned up by the generator of such wastes and the user of such sites.” The guidance specifically notes that the obligation does not require “the removal of any structure or waste material in circumstances where the removal by any practical option would result in greater adverse environmental impact than leaving the structure or waste material in its existing location.”
The need for remediation activities to be calibrated to optimise gains achieved by mitigation in comparison to any adverse effects of mitigation activities is recognised in these articles. There is a need to balance the impacts of remediation actions with the environmental benefits that accrue, and the Protocol appears to recognise this. It advocates not removing contaminants when removal creates a greater adverse impact on environmental values than leaving them in place (1 above) and requiring overt consideration of the potential impacts of the remediation itself (2 above). Making such evaluations is difficult, and a variety of decision-support tools for optimising remediation interventions have been developed (e.g. Anderson, Norrman, Back, Söderqvist, & Rosén, Reference Anderson, Norrman, Back, Söderqvist and Rosén2018). Selecting an appropriate course of action requires a methodology to collate impacts on diverse values and an understanding of interactions between environmental contaminants and ecosystem response. The latter is simplified in many jurisdictions through published threshold contaminant concentrations above which impact is assumed. However, the agreed threshold values for Antarctica do not yet exist.
The Vanda Station remediation involved a series of phases, from scoping the extent of contamination, identifying remediation objectives, designing a strategy, implementing the strategy and monitoring the outcome.
Scoping
To scope the remediation, a survey of soil contamination at the station site was undertaken in 1992–93 (Sheppard et al., Reference Sheppard, Campbell and Claridge1993). The survey was largely confined to the immediate area of the station, together with a few more distant reference sites. It reported areas of soil visibly contaminated with hydrocarbons and domestic wastes. Analyses of soils revealed elevated concentrations of metals, particularly cadmium, copper, lead and zinc relative to control sites. As expected, the soils of Greywater Gully were the most contaminated (Table 1). Contamination with nutrients, particularly phosphate (likely from detergents), was also evident in Greywater Gully soil samples; the report also listed “a wide range of solid materials (e.g. food scraps, fats, material fragments associated with wrapping and packaging, etc.).”
Table 1. Example results of readily leachable trace metal concentrations in soil samples collected in Greywater Gully and Vanda Station helicopter pads in 1992–93. Results are taken from Sheppard et al. (Reference Sheppard, Campbell and Claridge1993), and all measures are calculated as mg/kg. Each value is the mean of three depths within a soil profile. Control samples (V34 and V35) are from sites well clear of the station
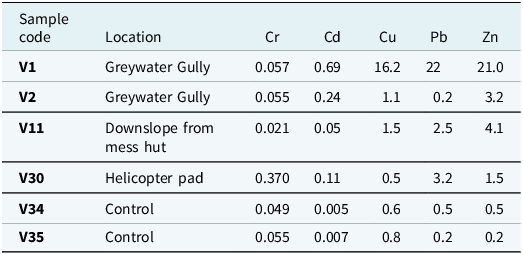
Planning
The planning process initiated by NZAP to cover the decommissioning and removal of Vanda Station was submitted as an IEE, as required under the Protocol, in 1993 for review by two New Zealand agencies independent of the IEE preparation, the Ross Dependency Research Committee and the Antarctic Environmental Assessment Panel (Howard-Williams, Reference Howard-Williams1993a, Reference Howard-Williams1993b; Waterhouse, Reference Waterhouse, Lyons, Howard-Williams and Hawes1997). The objectives of the decommissioning were summarised as:
-
“To ensure that in the event of the waters of Lake Vanda inundating the station, there will be an absolute minimum of human-induced environmental change to the lake system;
-
To ensure that if change is unavoidable, the nature of change is understood and documented; and
-
To submit proposals for protecting Lake Vanda to national and international review.”
The wording of these objectives recognises that a complete “return to pristine” condition was not possible. Rather the objective was to ensure an “absolute minimum” of human-induced change to the lake system, and that the nature of that change was documented. This was a pragmatic decision, given the extent and degree of contamination that was evident in soils in areas of activity. It is consistent with the Protocol, insofar as it implies that rehabilitation should proceed to a point where the benefits clearly outweigh the damage.
To provide context to “how clean is clean enough,” the trace metal and nutrient contamination levels reported by Sheppard et al. (Reference Sheppard, Campbell and Claridge1993) were compared with the trace metal and nutrient loads entering the lake each year via the Onyx River (Howard-Williams, Reference Howard-Williams1993a). The conclusions were that the site could add only a small proportion to the annual loads entering the lake from the natural inflows but that this would be localised. In addition, benthic algal mats (sensu Hawes & Schwarz, Reference Hawes and Schwarz2001) were expected to develop in the rising lake that would serve as traps for both nutrients and trace metals, particularly those bound to sediments, resulting in a degree of natural remediation. Once dispersed within the lake waters, the small increases that may have occurred over time would have made immeasurably small additions to concentrations naturally occurring in the lake and were considered unlikely to have persistent ecosystem consequences. The major concern of the IEE was the potential for contamination of lake water by the organic compounds that did not occur naturally in the lake (condensed polyphosphates, hydrocarbons, soot, animal fats, etc.), some of which were largely associated with the water that had accumulated in the Greywater Gully wastewater disposal site.
The final mitigation plan therefore included excavation and removal from the site of the soils and pooled groundwater most contaminated with materials that would not normally enter the lake but may impact ecological values, along with all surface structures and painted rocks, followed by grooming of the site to mimic the surrounding terrain and return aesthetic and wilderness values to the site. While the primary focus was on preventing adverse impacts on the lake ecosystem, aesthetic and wilderness values are given the same protection under the Protocol, and these measures were intended to restore these as far as possible to pristine.
In the final review of the IEE, Howard-Williams (Reference Howard-Williams1993b) provided detailed recommendations on the rehabilitation plan and concluded that, if the recommendations were followed, the decommissioning and removal of Vanda Station would have no more than a minor transitory impact and a Comprehensive Environmental Evaluation under Annex 1 of the Protocol was therefore not required. There was no argument that removing contaminants would have a positive impact.
A key feature of the IEE was the requirement for monitoring the site post-flooding to assess the rate of release and quantity of any contaminants from the station site entering the lake (Hayward et al., Reference Hayward, McFarlane, Keys and Campbell1994; Waterhouse, Reference Waterhouse, Lyons, Howard-Williams and Hawes1997).
Implementation
During the decommissioning process, all man-made structures above and below ground were removed, along with all debris that could be located, and the physical appearance of the site was restored as far as possible to a natural state (Hayward et al., Reference Hayward, McFarlane, Keys and Campbell1994; Waterhouse, Reference Waterhouse, Lyons, Howard-Williams and Hawes1997). Below-ground structures were limited to building anchors and a cool storage unit, all of which were fully removed during the summer thaw period.
Particular attention was paid to the “epicentre” of contamination, Greywater Gully (Fig. 6). In 1993–94, 400 kg of contaminated groundwater that had ponded above the permafrost at Greywater Gully was pumped into drums (Fig. 6) and flown back to Scott Base for treatment at the wastewater plant, together with a total of 7000 kg of contaminated soil from the Gully itself that was shipped to Scott Base for further treatment.

Figure 6. Greywater Gully, where liquid washing waste (personal and from kitchen) was deposited from 1969 to 1990. Left, the site prior to remediation in 1992; right, pump removing contaminated groundwater for transport back to Scott Base.
Painted rocks (most paints were lead-based) were removed where possible, along with soils adjacent to buildings that were visibly contaminated by hydrocarbons, and an early rubbish-burning site was dug out. A grand total of 15,000 kg of contaminated material was removed from the site by helicopter.
Post-remediation investigations
Developing a programme of post-remediation investigations in 1992 was effectively defined by a stated target of “an absolute minimum of human-induced environmental change to the lake system” and what was then known about the nature of contaminants, the sensitivity of the lake ecosystem to those, and weighted by the anticipation that the soon-to-be flooded soils could release limited amounts of the more soluble contaminants (e.g. zinc, naphthalene and phosphate) into the water column. There they could potentially have localised effects on the growth and species composition of microbial mats (Hawes, Smith & Sutherland, Reference Hawes, Smith and Sutherland1999; Webster et al., Reference Webster, Webster, Nelson and Waterhouse2003) and wider effects on the lake’s phytoplankton. Lake Vanda is an ultra-oligotrophic lake (Vincent & Vincent, Reference Vincent and Vincent1982; Canfield & Green, Reference Canfield and Green1985) and could potentially respond adversely to toxic contaminants and to increased nutrient concentrations.
Post-remediation monitoring activities at the site began in the summer of 1994–95 (Hawes & Howard-Williams, Reference Hawes and Howard-Williams1996; Supplementary Material Table 1) and included nutrients (N and P, though P was of prime concern due to the low natural load resulting in intense P limitation of phytoplankton growth – Vincent & Vincent, Reference Vincent and Vincent1982), trace metals in water and accumulated by benthic microbial communities, along with the composition and biomass of the microbial communities at the lake edge, as the lake rose towards and then encroached on the station site.
Three sites for monitoring were selected: Greywater Gully itself, which supported a meltwater pond in 1994–95 and was anticipated to connect to the lake very soon; an embayment in the lake (Vanda Bay, Fig. 2) directly in the potential flow path for ground and surface water from Greywater Gully; and an unimpacted control site (Control Bay, Fig. 2) well away from Vanda Station but with similar aspect and bathymetry to Vanda Bay (Sutherland, Reference Sutherland2002).
In the first two years following decommissioning, monitoring confirmed that contaminants still present in soils in Greywater Gully had the potential for contamination of Lake Vanda water. Elevated concentrations of N, P, Cd, Cr, Cu and Ni were found in the ponded water of Greywater Gully (Supplementary Material Table 2a). However, for the following six years, there was no evidence of metal enrichment reaching adjacent Lake Vanda waters (Supplementary Material Table 2b). In part, this was because, over that period, the lake did not rise (Fig. 5), and the anticipated flooding of Greywater Gully did not occur. Indeed, ponded water in Greywater Gully was not seen after the 1996–97 sampling. The seven years of annual monitoring did, however, demonstrate occasional significant variability in the nutrient concentrations in Vanda Bay due to variability in Onyx River flows (Sutherland, Reference Sutherland2002). It also noted that microbial mats with similar biomass and algal species composition were observed at both Control and Vanda bays (mostly cyanobacteria – mostly Leptolyngbya fragilis and L. frigida) and diatoms – Hantschia amphioxys, Luticola muticopsis, Stauroneis anceps, Muelleria peraustralis and M. meridionalis), though this was prior to the inundation of Greywater Gully.
An intensive evaluation of the state of the station site was undertaken in the 1996–97 summer, which revisited many of the locations sampled in the pre-remediation survey of 1992–93 and analysed for trace metals and hydrocarbons (Aislabie, Balks, Astori, Stevenson, & Symmonds, Reference Aislabie, Balks, Astori, Stevenson and Symons1999; Hawes et al., Reference Hawes, Smith and Sutherland1999; Webster et al., Reference Webster, Webster, Nelson and Waterhouse2003). These studies confirmed that hydrocarbon and trace metal contamination remained at the station site. Similar degrees of enrichment of contaminated soils (relative to controls) were measured for metals when compared to the 1992–93 study (Sheppard et al., Reference Sheppard, Campbell and Claridge1993), though the 1996–97 investigation examined a wider suite of metals, used more aggressive soil leaching and included subsurface environments (Webster et al., Reference Webster, Webster, Nelson and Waterhouse2003). Pb, Ag, Zn and Cd were still substantially enriched in soils from around the station site. It was also noted in 1996–97 that the hydrocarbons had moved only slowly through soils, though some low molecular weight fractions appeared to have been lost by volatilisation.
Despite the removal of 400 L of pooled groundwater from Greywater Gulley during remediation, a brine was found at the base of soil pits dug there in 1997. Phosphate and all trace metals investigated were elevated in this fluid, which also foamed, likely indicative of residual phosphatic detergents (Webster et al., Reference Webster, Webster, Nelson and Waterhouse2003). This brine was only a few cm thick, less than that encountered during the rehabilitation, and likely was again a mix of the downslope flow of supra-permafrost fluid flushing contaminants from soil and residual wastewater.
To assist with the evaluation of the impacts of soil contaminants on ecosystem processes, soil samples from the station and control sites collected in 1996–97 were returned to New Zealand, where laboratory bioassays showed that the soils from Greywater Gully supported an enhanced rate of growth and final yield of algae compared to control soils, attributed to nutrient enrichment (Hawes et al., Reference Hawes, Smith and Sutherland1999). However, this study also showed that cyanobacterial mats covering the soil surface mitigated contamination of overlying water by largely preventing nutrient contaminants within sediments from entering the water column, though only when illuminated. In darkness, particularly in the absence of oxygen, these mats did not prevent nutrient release.
A direct hydraulic connection from Lake Vanda into Greywater Gully finally occurred during the period of rapid lake level rise in the early 2000s (Fig. 3). The funded monitoring period was, however, complete, and no further post-flooding sampling was undertaken until 2014–15, by which time Greywater Gully had been inundated for approximately 12 years (Taylor, Reference Taylor2015). Lake water samples were collected from water overlying Greywater Gully, as closely as possible co-located with previous soil and SPF sampling. By 2014–15, few contaminated soil sites that were identified during the 1992–93 survey were still accessible, but those that were, were resampled, and coring of the flooded, contaminated sediments was attempted (Taylor, Reference Taylor2015). That resampling found that unflooded soils that were previously contaminated with metals, nutrients and hydrocarbons were still contaminated. No contaminants were detectable in the lake water overlying the station site, even water directly overlying the soil-water interface in Greywater Gully locations, though it is likely that the 12 years of hydraulic connection to the lake basin affected considerable advection and dilution of any released contaminants. The 2014 resampling revealed some small residual debris in the small, unflooded area, such as paint flecks and the occasional small metal objects such as nails, screws and small metal or wood pieces.
Discussion
How effective was the rehabilitation?
There were two separate environmental considerations to the Vanda Station decommissioning: (1) the physical remnants of human occupation and (2) the residual chemical legacy in the soils and groundwaters. Both were substantially but not completely remediated. Of the physical remnants, all easily identified materials were transported out of the Dry Valleys environment by helicopter, although, despite close ground searches, complete removal of very small items such as screws or nails, batteries and small metal or plastic shards (<1 cm) was not accomplished, evidenced by the continued finding of such items in the surface soil in 2014.
While broad-scale aesthetic and wilderness values were largely restored by the physical removal of buildings and soil grooming (Fig. 3), the post-decommissioning monitoring studies demonstrated the persistence of trace metal, nutrient and organic contaminants (PAHs, PCBs) in the soils and SPF (Taylor, Reference Taylor2015; Taylor, Webster-Brown, & Hawes, Reference Taylor, Webster-Brown and Hawes2016; Webster et al., Reference Webster, Webster, Nelson and Waterhouse2003). There has, however, been no evidence of elevated nutrients or trace elements in the lake water that has subsequently flooded Greywater Gully or the helicopter pads. Furthermore, healthy cyanobacterial mat development was observed at the sites of contamination, and the taxa present (species of the filamentous cyanobacterium Leptolyngbya and benthic diatoms) were largely those expected in this habitat in the McMurdo Dry Valleys (c.f. Ramoneda et al., Reference Ramoneda, Hawes, Pascual-García, Mackey, Sumner and Jungblut2021). The development of microbial mats may even have acted as a natural remediation by retarding the release of contaminants (Hawes et al., Reference Hawes, Smith and Sutherland1999), and in Lake Vanda, microbial mats appeared to sequester and attenuate contaminants, primarily through their ability to trap and encapsulate the fine contaminated sediment (Webster-Brown & Webster, Reference Webster-Brown and Webster2007). To this extent, the objectives of the remediation have been achieved in that there was no detectable human-induced environmental change to the lake system.
Complete elimination of unconstrained contaminants in Antarctic soils would not be possible without recourse to large-scale excavation (Waterhouse, Reference Waterhouse, Lyons, Howard-Williams and Hawes1997). While the availability of machinery at many Antarctic stations makes this feasible (ATCMXXVII, 2004, IP54), the collateral damage can be such that meeting the criterion of net benefit requires careful consideration. For a case such as Vanda Station, where high wilderness values could be re-established, the potential impact of transporting equipment capable of extensive excavations and shipping out or on-site treatment of removed materials could have resulted in large-scale degradation of the old station environs while the landscape slowly restabilised, with questionable tangible benefits.
Could the rehabilitation have been improved?
The experience at Vanda Station allows consideration of whether a more structured pathway for remediation of sites destined for removal and deconstruction would have been more or equally effective. We have re-evaluated the decision-making process at Vanda Station to determine if this could have been improved using a formal framework. We considered two such frameworks: the NEBA approach of Efroymson et al. (Reference Efroymson, Nicolette and Suter2004) and the later Antarctic Clean-up Manual (ATCM, 2019). The “Guiding Principles” of the Antarctic Clean-up Manual closely match the key phases of the NEBA process and follow a sequence: 1. site assessment; 2. environmental risk assessment; 3. environmental quality targets for clean-up; 4. consideration of clean-up options; 5. clean-up actions; 6. monitoring and evaluation.
In hindsight, we have aligned the decision-making processes at Vanda Station, including those of the Station Decommissioning IEE (Hayward et al., Reference Hayward, McFarlane, Keys and Campbell1994), with the recommendations of the Antarctic Clean-up Manual and with the NEBA process. These were:
-
1. Site assessment: The site assessment at Vanda included a soil contaminant survey (Sheppard et al., Reference Sheppard, Campbell and Claridge1993) and information on the chemistry of Lake Vanda and the inflowing glacial melt streams.
-
2. Environmental risk assessment: The risk assessment encompassed in the IEE (Hayward et al., Reference Hayward, McFarlane, Keys and Campbell1994) focussed on the rising levels of the lake that created urgency to decommission the station, recognition of the aesthetic and wilderness values of adjacent Lake Vanda and surroundings and the special ecosystem properties of Lake Vanda including its chemistry and microbial communities.
-
3. Environmental quality targets: The setting of environmental quality targets focussed on preventing contamination of Lake Vanda and the inclusion in the monitoring programme of “Control Sites” away from the station against which to measure contamination at the station site.
-
4. Consideration of clean-up options: This was included in the IEE (Hayward et al., Reference Hayward, McFarlane, Keys and Campbell1994) only in a binary sense, as the unacceptability of a “do nothing” option given the need to avoid nutrient and toxicant effects on Lake Vanda’s microbial communities with minimal disturbance to aesthetic and wilderness values.
-
5. Clean-up actions: In the case of the clean-up, no formal analysis was made other than a decision to remove and transport from the site all structures and the most contaminated soils and polluted groundwater at selected spots. The preliminary survey provided only a partial analysis of the extent of contamination and was biased by a focus on known sites of contamination. It was assumed that once the site was flooded, microbial mat formation over the contaminated sites including the Greywater Gully site would have been a natural barrier to contaminant leakage.
-
6. Monitoring and evaluation: At Vanda, this stage focussed on the lake quality and microbial communities along its shoreline as it encroached on the station site. An annual monitoring programme was designed with this in mind. A continued evaluation of contamination effects up to 2015 showed none were detectable as of 2015 when the station site flooded over (Fig. 4).
Major gaps in the Vanda Station clean-up process were:
-
a. Despite the initial site survey for contaminants covering 73 locations (Sheppard et al., Reference Sheppard, Campbell and Claridge1993), it was guided by local knowledge of areas known to have been contaminated or that were clearly contaminated from observations (particularly hydrocarbons). It was not systematic. As such, it did not provide a sufficient dataset from which to determine the extent of contamination and to quantify the scale of rehabilitation required. Post-rehabilitation monitoring showed that substantial contamination remained, though again post-rehabilitation monitoring was not systematic. Essentially, it demonstrated that the clean-up was only partial.
-
b. Limited attempts were made to evaluate different courses of action. Essentially, a binary question was considered, whether to take remediation action or not. No methodology was subsequently defined for assessing the net benefit of planned remediation, and, in combination with (a) above, this resulted in no systematic consideration of the scale of remediation that was needed to meet defined objectives and limited justification for the levels of residual contamination.
-
c. No threshold concentrations were defined against which to view the rehabilitation and post-rehabilitation monitoring.
-
d. Structured monitoring of the site ceased prior to known areas of residual contamination being flooded.
In hindsight, these gaps stem from a common root, a lack of published research and accepted guidelines to inform the clean-up framework and setting of threshold concentration targets that would have allowed rehabilitation options to be evaluated. An important contributor to this was the limited toxicological testing that had been undertaken on Antarctic organisms for the contaminants under consideration at the time that the remediation was planned. Likewise, there was little information on the extent to which Antarctic ecosystems naturally rehabilitate contaminants, how contaminants move through soils and over permafrost, nor the timescales over which this occurs. Similar limitations applied to knowledge of the timescales of recovery from physical disturbance that may be particularly acute in the arid soils of polar deserts, where the wind plays a major role in developing soil surfaces, and biotic or water interactions with soils are slow (Hewitt, Balks & Lowe, Reference Hewitt, Balks and Lowe2021). This absence of disturbance recovery data limited the ability to assess the impacts of remediation options that result in soil damage.
There remains a paucity of information on all of the issues today. We note, however, that the existing evidence does not support a generalisation that Antarctic organisms are unusually vulnerable to hydrocarbons and trace metals (e.g. Darham et al., Reference Darham, Zakaria, Zulkharnain, Sabri, Khalil, Merican and Ahmad2023; Fernández et al., Reference Fernández, Martorell, Blaser, Ruberto, de Figueroa and MacCormack2017; Gran-Scheuch et al., Reference Gran-Scheuch, Ramos-Zuñiga, Fuentes, Bravo and Pérez-Donoso2020; Tomova, Stoilova-Disheva, Lazarkevich, & Vasileva-Tonkova, Reference Tomova, Stoilova-Disheva, Lazarkevich and Vasileva-Tonkova2015; Zahri et al., Reference Zahri, Gomez-Fuentes, Sabri, Zulkharnain, Khalil, Lim and Ahmad2021). It may prove that thresholds and guidelines established in other jurisdictions will prove suitable for Antarctica, but further research is required to determine this.
Setting a single set of thresholds for contaminants in Antarctica is, however, unlikely to be effective, due to profound differences in the biota, climate and physical settings around the continent. Thresholds suited to the hyper-arid polar deserts, inhabited by sparse, highly stress-tolerant microbial communities, are unlikely to apply in the continent’s relatively mild, wet maritime regions. How then to approach threshold setting? For evaluating contaminants at Vanda Station, two methods were used that were based on local comparisons: assessing the risk of raising trace metal loads to the lake itself by comparing the potential for contamination with the natural loads and comparing contaminant concentrations in soils with concentrations in local control soils (Hayward et al., Reference Hayward, McFarlane, Keys and Campbell1994; Howard-Williams, Reference Howard-Williams1993b). It may be that local thresholds can be set by setting limits based on a statistically significant increase over local control sites or loading rates, though without an understanding of sensitivity, this still precludes ecological effects-based assessments.
Since the implementation of the Protocol, comprehensive pre-construction surveys (Comprehensive Environmental Evaluations) are required to be completed for all new installations. To aid the inevitable clean-up that will be required when these sites are removed or replaced, we suggest that these surveys should consider the remediation of the site once the occupation is terminated. They should also include baseline studies of potential contaminants within soils, waterways and adjacent coastal regions to allow contamination to be recognised. Analytical procedures are likely to improve, and new contaminants emerge, over the lifetime of installations, and we further recommend that samples of soils, sediments and waters should be archived to allow future analysis. Where it is too late to undertake pre-construction surveys, we recommend that “otherwise similar” locations nearby be used to derive baseline conditions of soils, sediments and waters. This will allow thresholds to be developed that will indicate when soils are likely to be contaminated, but further research is required to determine whether they pose a risk to local organisms.
Assessing the ability of native biota to tolerate and attenuate contaminants at concentrations above these baselines is the second element required to set thresholds. The relative species paucity of many locations in Antarctica may make site-specific toxicological experiments on locally dominant taxa tractable. Ultimately, these could contribute to a comprehensive, spatially explicit database on the pollution-tolerance of representative Antarctic biota.
A challenge for the Vanda Station rehabilitation was the time factor – no action was taken until inundation was seemingly imminent. We recommend that research to guide rehabilitation should begin well before it is operationally required. We further recommend that the research should not only assess the vulnerability of local biota to likely contaminants but also the positive aspects of how natural remediation or sequestration might occur. From Vanda Station, for example, we learned that natural microbial mats could attenuate and sequester contaminants under suitable conditions, though this was determined after the rehabilitation was terminated (Hawes et al., Reference Hawes, Smith and Sutherland1999).
Further considerations for developing optimal rehabilitation schemes will require acknowledgement of timelines for recovery following different treatment options. An example is provided in the NEBA process (Efroymson et al., Reference Efroymson, Nicolette and Suter2004) summarised here in Figure 7. Depending on the likelihood of natural attenuation processes, the rates of dispersion, the timeline of the remediated state and other factors, decisions may be made at several points on continuing remediation following a contamination event. The scale of soil removal required, for example, needs to be considered in relation to the recovery timescales of local soils. Minor surface soil removal may be a net benefit, whereas after the removal of contaminated soils down to the permafrost, decadal timelines will be needed to re-establish a soil structure reminiscent of the original (Campbell, Claridge, & Balks, Reference Campbell, Claridge and Balks1998; O’Neill, Balks, López-Martínez & McWhirter, Reference O’Neill, Balks, López-Martínez and McWhirter2012). Ultimately, a spectrum of assessment outcomes might be expected (Fig. 7), requiring immediate and disruptive remediation actions at one extreme and no action at the other. For example, relatively inactive or benign contaminants, strongly bound to subsurface soils, which are not expected to come into contact with meltwater or which are isolated in pockets of dense, hypersaline groundwaters, may not warrant remediation. Similarly, rapid natural attenuation processes may limit the need for significant physical intervention such as excavations of contaminant sites.

Figure 7. Hypothetical trajectories of environmental status following establishment, removal and recovery at a facility such as Vanda Station in Antarctica.
-
A. Various aspects of environmental status degrade relative to reference sites over time during site occupation.
-
B. Status may decline during removal and rehabilitation depending on the choice of action.
-
C. Intense remediation with rapid and effective natural recovery.
-
D. Physical remediation with slow natural recovery.
-
E. Do nothing option, with natural attenuation of contaminant effect.
-
F. Do nothing option, with natural recovery prevented by residual contaminants.
Not all aspects of the “Environmental State” will respond similarly to the planned intervention. Natural variability is not depicted. Modified from Figure 6 of Efroymson et al. (Reference Efroymson, Nicolette and Suter2004).
Climate change as a factor
Local variability in climate resulting in lake level rise was a significant component of the Vanda Station planning process as predictions were that the contaminated site would soon be subsumed into an ultra-oligotrophic lake. Had the flooding not been anticipated, the impact of the contaminants on soil ecosystems rather than lake ecosystems would have been required, and a rehabilitation involving less contaminant removal, less soil disturbance and greater reliance on natural attenuation could have been considered.
It is anticipated that increasing wetness is likely across much of Antarctica in response to climate change (Lee et al., Reference Lee, Raymond, Bracegirdle, Chadès, Fuller, Shaw and Terauds2017), and we argue that consideration of the likely impacts of this on remediation needs must be included in the assessment process. Future increases in surface meltwater, or subsurface permafrost meltwaters, potentially provide a mechanism for lateral distribution of contaminants as well as increasing their bioavailability. The scale of soil removal required needs to be considered in relation to the recovery timescales in different types of Antarctic soils. Minor surface soil removal may be a net benefit, whereas after the removal of contaminated soils down to the permafrost, decadal timelines will be needed to re-establish a soil structure reminiscent of the original. Future climate-driven increases in surface meltwater, or subsurface permafrost meltwaters, may provide a mechanism for wide lateral distribution of contaminants as well as increasing their bioavailability.
The wider picture
The number of facilities that have been moved or deconstructed throughout Antarctica is poorly documented. Based on extrapolation from contaminated sites at Australia’s Casey Station, Snape et al. (Reference Snape, Riddle, Stark, Cole, King, Duquesne and Gore2001) estimated that the volume of abandoned, unconfined waste materials in Antarctica may be greater than 1 million m3 and that the volume of hydrocarbon-contaminated sediment may be similar. Contaminated sites are thus likely throughout the continent, and a strategy for developing rehabilitation that meets the Protocol criterion of avoiding removal of contaminants using options that would result in greater adverse environmental impact than leaving in place is required.
Although Vanda Station is one of few stations that have been decommissioned under an approved IEE in Antarctica, there have been a number of facilities such as field huts or long-term encampments and waste disposal sites that have been dismantled and removed for a number of reasons. For example, Peter, Buesser, Mustafa and Pfeiffer (Reference Peter, Buesser, Mustafa and Pfeiffer2008) reported on several huts dismantled, with some subsequent clean-up efforts on the Fildes Peninsula, King George Island. In addition, 24 bases around the continent are located at 10 m or less above sea level, a number at 5 m or less, and sea-level rise may require their removal (www.coolantarctica.com/Community/antarctic_bases.php). While sea-level rise may not be an issue around some parts of the continent due to continuing isostatic uplift (SCAR-COMNAP, 2023), there are places where it may well require facility removal. ATCM (2012) summarises 43 such actions and notes that “Long-term planning of clean-up operations is important, including reliable site characterisations, design and implementation of clean-up activities, and monitoring during and after clean-up operations.” With changing priorities for research, modernising facilities and with the projected impacts of climate change, we suspect that dismantling and removal of facilities on ice-free land will continue.
Within the McMurdo Dry Valleys, facilities other than Vanda Station have been moved or removed with varying degrees of rehabilitation and monitoring. New Zealand alone has disestablished at least three small inland field facilities in addition to Vanda Station, including huts in Miers, Wright and Taylor Valleys. Other buildings established close to now-rising lakes have also had to be moved upslope including include US Antarctic Programme facilities in the lakes Bonney, Hoare and Fryxell, with old sites fully or partially flooded (e.g. the original Lake Bonney station). It is of interest that Antarctic Specially Managed Areas (ASMAs) may provide an additional solution and a tool into which the processes in the Antarctic Clean-up Manual and/or the NEBA approach can be integrated. The McMurdo Dry Valleys ASMA Management Plan (ATCM, 2015) includes a spatial management component involving activities zoning. For instance, “Facilities Zones” with designated activities within defined boundaries include provision for fuel storage and containment and waste minimisation and management. Mandating of accurate site mapping of where workshops, fuel containers and toilet facilities are located for subsequent environmental impact assessment is required. Section 7(iii) of the ASMA Management Plan covers the installation, modification or removal of structures and directs that permanent or semi-permanent structures should not be installed outside defined Facilities Zones unless they are small (e.g. VHF radio repeater stations). In this way, the ASMA plan will mitigate against contaminant release, document vulnerable locations and limit physical disturbance in the case of any decommissioning.
The Antarctic Clean-up Manual and the NEBA approach show similar pathways that will be of use, but based on the Vanda Station experience, modifications could be made to improve the application of this framework in an Antarctic environment. More guidance to support a decision on whether to extract contaminated soils, isolate them within the landscape or leave them in place without remediation is warranted. Hemmings and Kriwoken (Reference Hemmings and Kriwoken2010) challenged the existing environmental assessment process in Antarctica along the lines that the evaluations were invariably undertaken by the proponents, that the legal jurisdiction covering operations in Antarctica can be vague and argued that a more robust international approach to environmental assessment would potentially achieve better outcomes, and this can be extended to facility clean-up decision-making as discussed here. We note also that at Vanda Station, the lack of systematic records of contamination meant that when it came to assessing the area contaminated, this was largely by anecdote and visual assessment. We recommend that National Antarctic Programmes continue to maintain proactive spill reporting and rapid remediation activities to prevent the type of cumulative impact seen at Vanda Station.
Conclusions: lessons from the Vanda Station removal
In this contribution, we set out to use the historic Vanda Station decommissioning, for which pre- and post-remediation data were obtained, to illustrate challenges to the remediation of formerly occupied sites in Antarctica. We found that recent guidelines on the clean-up of contaminated sites in Antarctica provide valuable guidance but that challenges remain, largely due to a lack of understanding about the consequences of contamination on Antarctic ecosystems. We conclude that:
-
1. Frameworks are needed to guide setting and reviewing targets for decontamination and to provide mechanisms to evaluate clean-up progress. This will require research to inform guideline derivation, contaminant mobility and attenuation pathways and likely recovery trajectories for the site.
-
2. Complete removal of contaminants released into the environment will never be possible, and a decision is needed on whether the benefits of attempting to remove contaminated soils, and the damage that this entails, outweigh the benefits of leaving them in situ with minimal disturbance of the soil profile.
-
3. Comprehensive Environmental Evaluations for all new installations should consider the challenges of remediation of contaminants and include baseline studies of potential contaminants in the environment, the preservation of samples for later analysis and consideration of the vulnerability of local biota to contaminants.
-
4. When planning remediation, the scale of activities needs to be considered in relation to the recovery timescales in different Antarctic regions and in relation to future climate conditions and meltwater dynamics.
-
5. Remediation planning should begin sufficiently before the remediation is necessary to allow comprehensive evaluation of the process.
-
6. Research on the toxicology of Antarctic organisms, especially hydrocarbons and other contaminants commonly used in Antarctic field stations, with a view to developing regionally robust threshold criteria, should be prioritised.
-
7. While soil and water quality guidelines for natural Antarctic systems will be useful, there is also a need to rely on control sites and high-quality pre-construction surveys, for assessing rehabilitation targets. We should consider archiving samples for future analyses.
-
8. Post-rehabilitation monitoring to determine whether the targets have been met, and reporting on this, is a critical final stage. These should continue until a new equilibrium is reached. Adaptive management may be required.
-
9. There needs to be explicit consideration of climate change in designing remediation measures.
-
10. Sites established and managed in accordance with the Protocol may still be contaminated but most likely to a lesser extent than in older sites. New contaminants (DNA, black carbon, etc) will continue to emerge.
Supplementary material
The supplementary material for this article can be found at https://doi.org/10.1017/S0032247424000354.
Acknowledgements
The authors wish to acknowledge the New Zealand National Institute of Water and Atmospheric Research (CH-W). The Antarctic Science Platform and our colleagues around the world for many discussions that have stimulated our thinking on the issues covered in this manuscript.
Financial support
We thank the New Zealand Antarctic Science Platform for support through MBIE Contract ANTA1801.
Competing interests
The authors declare no financial benefit from the publication of this article. Author Howard-Williams was involved in the design of the assessment of the Vanda Station remediation, and authors Howard-Williams, Hawes and Webster-Brown were involved in the post-rehabilitation monitoring of the site. Author Poirot is employed as an Environmental Manager by Antarctica New Zealand, the organisation that oversaw the rehabilitation of the site.
Ethical standards
The authors assert that all procedures contributing to this work comply with the ethical standards of the relevant national and institutional committees on human experimentation and with the Helsinki Declaration of 1975, as revised in 2008.
The authors assert that all procedures contributing to this work comply with the ethical standards of the relevant national and institutional guides on the care and use of laboratory animals.