In many lakes worldwide, as well as in marine environments, diatoms are an important component of phytoplankton populations. Accumulations of diatoms are known from all aquatic environments including wetlands, lakes, and the marine environment (Clarke, Reference Clarke2003). In marine environments, accumulations of diatom-rich sediments extend back to the Late Cretaceous (Harwood et al., Reference Harwood, Nikolaev and Winter2007), whereas the oldest diatom-rich lake sediments are from the Eocene (Flower et al., Reference Flower, Elias and Mork2013), with large deposits found in the Miocene (Bradbury and Krebs, Reference Bradbury and Krebs1995). Massive accumulations of fossil diatom frustules have been observed in multiple lakes situated in silica-rich environments, especially in volcanic and hydrothermally active areas, such as Yellowstone Lake, US (Theriot et al., Reference Theriot, Fritz, Whitlock and Conley2006), Lake Myvatn, Iceland (Opfergelt et al., Reference Opfergelt, Eiriksdottir, Burton, Einarsson, Siebert, Gislason and Halliday2011), or Lake Challa, Tanzania/Kenya (Barker et al., Reference Barker, Hurrell, Leng, Plessen, Wolff, Conley, Keppens, Milne, Cumming, Laird, Kendrick, Wynn and Verschuren2013). In these settings, the high dissolved silicon concentrations promote the growth of diatoms (Wallace, Reference Wallace2003). However, high diatom concentrations in sediment also have been observed in lakes with no volcanic or hydrothermal influence, for example in lakes of Northern Sweden (Frings et al., Reference Frings, Clymans, Jeppesen, Lauridsen, Struyf and Conley2014) or Lough Neagh, Ireland (Plunkett et al., Reference Plunkett, Whitehouse, Hall, Brown and Baillie2004). In the oceanic environment, high biogenic silica accumulations occur in the equatorial Pacific Ocean, where diatoms grow in zones fed by continental siliceous dust and nutrients brought by upwelling. Similarly, cold-water regions, such as the productive Antarctic convergence zone, have sufficient nutrient and dissolved silicon supply that diatom-rich sediments are formed (Flower et al., Reference Flower, Elias and Mork2013).
Conger (Reference Conger1942) hypothesized that the requirements for diatomaceous accumulation are to have (1) conditions favourable for diatom growth, and (2) a reduced accumulation of other sedimentary constituents that would dilute the concentration of the siliceous tests of diatoms. Diatom growth is dependent on many environmental factors, such as dissolved silicon availability, phosphorus and nitrogen availability, pH, salinity, and light (Battarbee et al., Reference Battarbee, Jones, Flower, Cameron, Bennion, Carvalho, Juggins, Smol, Birks, Last, Bradley and Alverson2002). Diatom test preservation is sensitive to temperature and pH; biogenic silica dissolves faster with increasing pH (>8) and temperature (Alexander et al., Reference Alexander, Heston and Iler1954). Although diatom-rich sediments are found worldwide in various environments, of various ages and various settings, the terminology for classifying high accumulations of diatom frustules in sediment is not consistent in the literature, particularly in the Quaternary literature.
Sediments can be classified based on the sedimentary environment, sedimentary structures and processes, and sediment texture and composition, including sorting, shape of grains, grain size, and the ratio of matrix to grains. Grain size, for example, is used in various classification systems (e.g., Wentworth, Reference Wentworth1922 or see summary in Pettijohn [Reference Pettijohn1949]). This is appropriate for minerogenic sediments, but biogenic sediments contain more than just mineral grains. Biogenic sediments (also called bioclastic or organic) are composed of skeletal remains, shells, or tests from organisms composed of biogenic silica or calcium carbonate, or remains of soft organic material, mainly organic carbon. The classification of calcium carbonate sediments based on texture and the ratio of matrix to the abundance of grains is well described by Dunham (Reference Dunham and Ham1962), and an effective general classification of lacustrine sediments was proposed by Schnurrenberger (Reference Schnurrenberger, Russell and Kelts2003), but it has not been widely used.
The first usage of the word diatomite dates to the nineteenth century from deep-sea deposits that were called diatom ooze, collected during the voyage of the HMS Challenger (Murray and Renard, Reference Murray and Renard1891). Later, Conger (Reference Conger1942) described pure diatomaceous earth as material that reached a purity of 95 to 98% of diatom silica. According to Terzaghi et al. (Reference Terzaghi, Peck and Mesri1996), diatomaceous ooze should be used for loose unconsolidated sediment containing mainly diatoms. In colloquial nonscientific literature, diatomaceous earth is used as a name for both milled diatomite and for diatomaceous ooze, which creates ambiguity in the usage of diatomaceous earth as a definition. For this reason, we exclude diatomaceous earth from our proposed classification, which is intended for use in scientific literature rather than in the public domain.
Inglethorpe (Reference Inglethorpe1993) described the characteristics of diatomite as a unique combination of physical and chemical properties (high porosity, high permeability, small particle size, large surface area, low thermal conductivity, and chemical inertness) that make diatomite suitable for a wide range of industrial applications. Diatomite was defined as a “pale coloured, soft, light-weight rock composed principally of the silica microfossils” (Inglethorpe, Reference Inglethorpe1993, p. 1). A diatomite of high SiO2 purity (ranging from 80 to 99 wt% of biogenic SiO2) is now commonly used in scientific research as a reference material in isotope geochemistry for the measurement of stable silicon isotopes. One widely used standard (Reynolds et al, Reference Reynolds, Aggarwal, André, Baxter, Beucher, Brzezinski and Engström2007) originates from the Lompoc quarry in California, more precisely from the Miocene strata of the Monterey Formation, which is well known for numerous lithological stages of siliceous deposits—diatomite, diatomaceous shales, diatomaceous mudstones, porcelanite, and cherts (Bramlette, Reference Bramlette1946). The Lompoc area is well described by Bramlette (Reference Bramlette1946), including a description of the purity of diatomaceous deposits.
Various generalized classification systems for lacustrine and marine sediments have been proposed by Dean (Reference Dean, Leinen and Stow1985), Mazzullo et al. (Reference Mazzullo, Meyer, Kidd, Mazzullo and Graham1988), Owen (Reference Owen2002), and Schnurrenberger (Reference Schnurrenberger, Russell and Kelts2003). Dean (Reference Dean, Leinen and Stow1985) and Mazzullo et al. (Reference Mazzullo, Meyer, Kidd, Mazzullo and Graham1988) proposed that biogenic sediment be defined as sediment that contains at least 50% of biogenic material. In this approach, biogenic content is estimated visually or by point-counting on smear slides, which has been an efficient method for calcareous nanoplankton sediment classification. For siliceous nanoplankton (diatoms, radiolarians, and chrysophyte cysts), which are comparatively big and porous, the point-counting method can both overestimate (Dean et al., Reference Dean, Leinen and Stow1985) and underestimate (Conley, Reference Conley1988) the percentage of biogenic silica. An alternative approach is to use the wt% biogenic silica relative to the dry sediment to classify unconsolidated biogenic silica-rich sediment using the methods of DeMaster (Reference DeMaster1981), Morlock and Froelich (Reference Mortlock and Froelich1989), or Conley and Schelske (Reference Conley, Schelske, Smol, Birks, Last, Bradley and Alverson2001). However, the weak base extractions dissolve only biogenic silica that is classified as opal-A, and sediments that are more diagenetically altered containing highly ordered opal-CT require a much stronger base.
A detailed classification based on diatom SiO2 content by Owen (Reference Owen2002) does not consider the stage of lithification caused by diagenetic processes. Diagenetic processes decrease the sediment porosity and alter the crystalline structure of SiO2. For example, non-crystalline opal-A (such as the SiO2 found in live siliceous organisms and their biogenic remains) is transformed into the disordered silica polymorph opal-CT (Rice et al., Reference Rice, Freund, Huang, Clouse and Isaacs1995) with some of the stacking disorder removed (Murata and Randall, Reference Murata and Randall1975). Temperature and burial depth play an important role in the extent of diagenesis. Therefore, the interpretation of diatomite raises an important second question of whether an unconsolidated sediment can be called diatomite. We suggest here that diatomite, a term widely used for diatom-rich sediments, be used only for consolidated sediments.
We are using a variety of previous studies as a guide, including Murray and Renard (Reference Murray and Renard1891), Conger (Reference Conger1942), Bramlette (Reference Bramlette1946), Murata and Larson (Reference Murata and Larson1975), Isaacs (Reference Isaacs, Garrison, Douglas, Pisciotto, Isaacs and Ingle1981c), Pedersen (Reference Pedersen1981), Kadey (Reference Kadey1983), Dean et al. (Reference Dean, Leinen and Stow1985), Mazzullo et al. (Reference Mazzullo, Meyer, Kidd, Mazzullo and Graham1988), Minoura et al. (Reference Minoura, Susaki and Horiuchi1996), Inglethorpe (Reference Inglethorpe1993), Lemons (Reference Lemons1996), Akin et al. (Reference Akin, Schembre, Bhat and Kovscek2000), Owen (Reference Owen2002), Moyle (Reference Moyle, Dolley, Bliss, Moyle and Long2003), and Schnurrenberger (Reference Schnurrenberger, Russell and Kelts2003). We adopt the recommendation from Mazzulo et al. (Reference Mazzullo, Meyer, Kidd, Mazzullo and Graham1988), in which any sediment material that is present in the sediment with more than 10% of the composition is considered as a modifier and, therefore, it should be stated in the name. The following definitions of diatomaceous ooze and diatomite are proposed in Figure 1 and we suggest nomenclature for defining related materials rich in diatom silica.
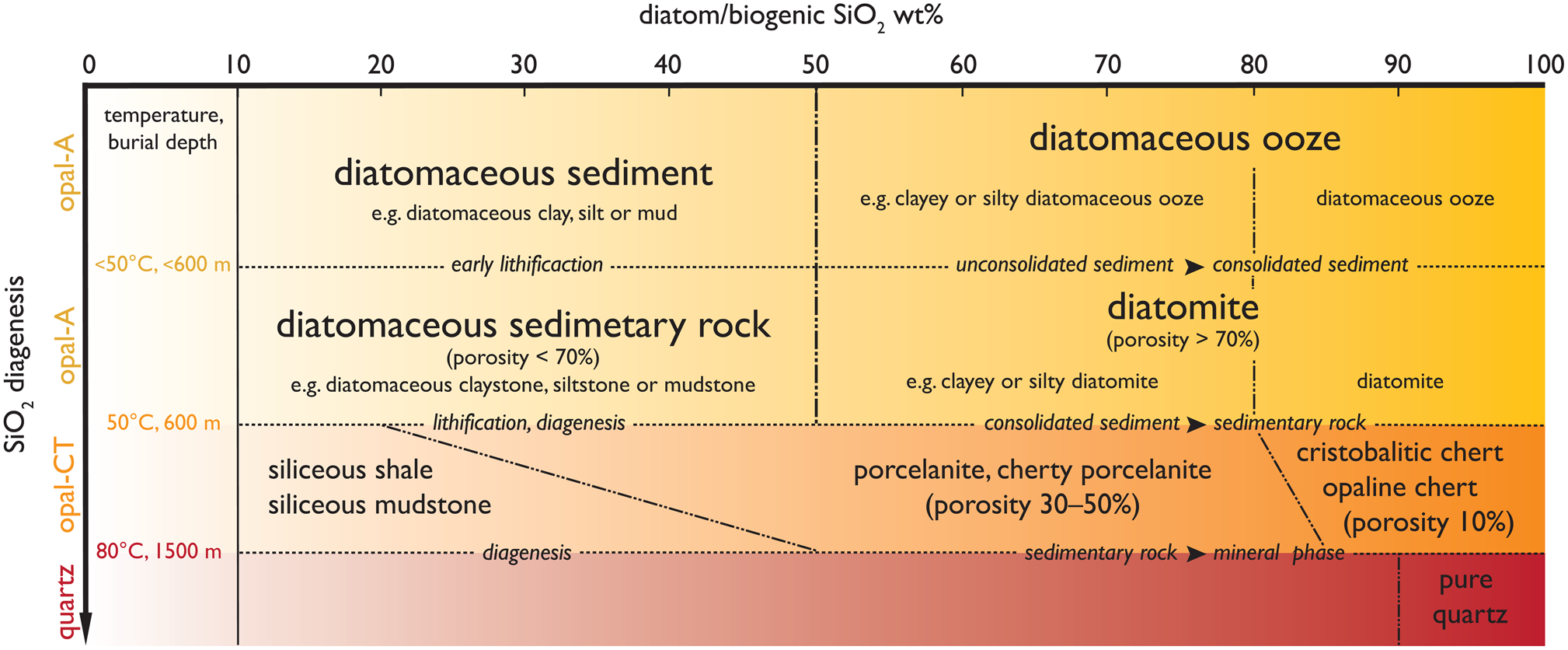
Figure 1. Proposed classification of sediment containing diatoms. The weight percentage (wt%) of diatom/biogenic SiO2 contained in sediments is on the x-axis and their transformations as a function of burial depth appears on the y-axis.
The principal name describing unconsolidated sediment containing diatom frustules with more than 50% of sediment weight is diatomaceous ooze. Oozes have a content of 50 to 80 wt% of diatom SiO2 together with another major sediment component, such as clay or silt. This major sediment modifier should be stated in the name, e.g., clayey or silty diatomaceous ooze. The stand-alone principal name diatomaceous ooze is used for ooze composed of more than 80 wt% of diatoms without any major sediment modifier. Unconsolidated sediment with diatom frustules less than 50% of sediment weight should be called diatomaceous sediment using supporting sediment terminology, such as diatomaceous clay, diatomaceous silt, or diatomaceous mud, to describe the matrix. Sediments with less than 10 wt% of diatom SiO2 are named based on the clastic sediment classification without reference to diatoms.
Diatomite is defined as a siliceous/opaline consolidated sediment with a diatom SiO2 content higher than 50% of sediment weight and porosity higher than 70%. As diatomites are often composed of 50–80% by weight of diatom SiO2, the major sediment modifier material must be named with the adjectives describing the sediment matrix components, such as clay or silt, for example clayey or silty diatomite. Further, we suggest that the stand-alone principal name diatomite is used strictly for consolidated sediment with more than 80% of sediment weight comprised of diatom SiO2. Chrysophyte cysts, radiolarians, and sponge spicules can be a minor part of the sediment (Bramlette, Reference Bramlette1946). Consolidated sediments containing less than 50% sediment weight of diatom SiO2 are then named based on the prevalent sediment component, e.g., diatomaceous claystone, siltstone, or mudstone. The porosity of these consolidated sediments should be lower than 70%. Diatomite and diatomaceous sediments are formed at low temperatures and pressures (<50°C and burial depth <600m). Significant recrystallization occurs at higher temperature and burial depths.
The boundary at 50% by weight of diatom/biogenic SiO2, e.g., between diatomaceous sediment and diatomaceous ooze (unconsolidated sediment) or between diatomaceous sedimentary rock and diatomite (consolidated sediment), as shown in Figure 1, is consistent with the widely used Ocean Drilling Program marine sediment classification system (Mazzullo et al., Reference Mazzullo, Meyer, Kidd, Mazzullo and Graham1988), as well as the commonly used sedimentological nomenclature practices described by e.g., Schnurrenberger et al. (Reference Schnurrenberger, Russell and Kelts2003). The boundary at 80% by weight of diatom/biogenic SiO2, e.g., between clayey or silty diatomaceous ooze and diatomaceous ooze (unconsolidated sediment) or between clayey or silty diatomite and diatomite (consolidated sediment), is based on the industrial and commercially used diatomite, which has a diatom content higher than 80% by weight.
At burial depths between 600 to 1500 m and temperatures ranging from 50 to 80°C, as found for example in the Monterey Formation, diatomite is transformed into opaline chert with a low porosity of around 10% (Isaacs, Reference Isaacs and Isaacs1981b, Reference Isaacs, Garrison, Douglas, Pisciotto, Isaacs and Ingle1981c). Chert is then defined as sedimentary rock with more than 80% of sediment weight originating from biogenic SiO2 (predominantly diatom SiO2). Diagenetic processes transform the biogenic SiO2 (chrysophyte cysts, diatoms, radiolarians, and sponge spicules), and the original source of SiO2 is difficult to determine, although fossils can be found in cherts (Pessagno and Newport, Reference Pessagno and Newport1972). For this reason, we use biogenic SiO2 in our definition of chert instead of the wt% of diatom SiO2. Diatomites and diatomaceous sediments containing, for example, clay or silt in combination with between 20 and 85 wt% biogenic SiO2, exposed to deeper burial depths and higher temperatures are altered to porcelanite or cherty porcelanite, with a final porosity between 30 and 50%, depending on the clay/silt content (Bramlette, Reference Bramlette1946; Isaacs, Reference Isaacs and Isaacs1981a; MacKinnon, Reference MacKinnon1989). Sediments that contain less than 20 to 50 wt% biogenic SiO2 under these conditions form shales, siliceous shales or siliceous mudstones, depending on the mineral content (Isaacs, Reference Isaacs and Isaacs1981a).
With increasing temperatures and burial depth, the final stage of alteration is reached at depths between 1500 to 2000 m and temperatures above 80°C; these conditions result in recrystallization of opal-CT into quartz (Murata and Larson, Reference Murata and Larson1975; Isaacs, Reference Isaacs1980). The boundaries based on biogenic SiO2 content between cherts, and especially between porcelanites and shales, are not sharp (Fig. 1). This is because the definitions of these siliceous sedimentary rocks take into account not only porosity and content of biogenic SiO2 but also hardness, fracturing patterns, and other petrological criteria (Isaacs, Reference Isaacs and Isaacs1981a), as diagenesis changes the properties of the sedimentary rock. We summarize our suggested classification of diatomaceous sediments and sedimentary rocks based on diatom/biogenic SiO2 wt% in Figure 1.
In summary, there are inconsistencies in the present use of the term diatomite and diatomaceous sediment throughout the literature. Based on our definition, unconsolidated lake or marine sediments that have not undergone any diagenesis (burial depth and temperature, as defined above), such as those of Holocene age, should not be referred to as diatomite, but instead should be called diatomaceous ooze. We encourage scientists working with diatomaceous sediments and sedimentary rocks to apply the definitions used here to ensure consistent use of terminology and hence comparability among studies. For sediment types that do not fit within the parameters that we have defined (Fig. 1), we suggest that all sediment components (mineralogy, texture, and porosity) be described in detail. We believe that proper naming will bring clarity to future studies focused on diatomaceous sediments and diatomite.
ACKNOWLEDGMENTS
This work was supported by The Royal Physiographic Society in Lund, by the Center for Geosphere Dynamics (UNCE/SCI/006) and by the Charles University project (GA UK 40217) to PZ, the Swedish Research Council to DJC, and NSF EAR-1514814 to SCF.