Book contents
- Frontmatter
- Contents
- Preface
- Frequently Used Notation
- TWO-PHASE FLOW, BOILING AND CONDENSATION IN CONVENTIONAL AND MINIATURE SYSTEMS
- PART ONE TWO-PHASE FLOW
- PART TWO BOILING AND CONDENSATION
- APPENDIX A Thermodynamic Properties of Saturated Water and Steam
- APPENDIX B Transport Properties of Saturated Water and Steam
- APPENDIX C Thermodynamic Properties of Saturated Liquid and Vapor for Selected Refrigerants
- APPENDIX D Properties of Selected Ideal Gases at 1 Atmosphere
- APPENDIX E Binary Diffusion Coefficients of Selected Gases in Air at 1 Atmosphere
- APPENDIX F Henry's Constant of Dilute Aqueous Solutions of Selected Substances at Moderate Pressures
- APPENDIX G Diffusion Coefficients of Selected Substances in Water at Infinite Dilution at 25°C
- APPENDIX H Lennard–Jones Potential Model Constants for Selected Molecules
- APPENDIX I Collision Integrates for the Lennard–Jones Potential Model
- APPENDIX J Physical Constants
- APPENDIX K Unit Conversions
- References
- Index
- References
References
- Frontmatter
- Contents
- Preface
- Frequently Used Notation
- TWO-PHASE FLOW, BOILING AND CONDENSATION IN CONVENTIONAL AND MINIATURE SYSTEMS
- PART ONE TWO-PHASE FLOW
- PART TWO BOILING AND CONDENSATION
- APPENDIX A Thermodynamic Properties of Saturated Water and Steam
- APPENDIX B Transport Properties of Saturated Water and Steam
- APPENDIX C Thermodynamic Properties of Saturated Liquid and Vapor for Selected Refrigerants
- APPENDIX D Properties of Selected Ideal Gases at 1 Atmosphere
- APPENDIX E Binary Diffusion Coefficients of Selected Gases in Air at 1 Atmosphere
- APPENDIX F Henry's Constant of Dilute Aqueous Solutions of Selected Substances at Moderate Pressures
- APPENDIX G Diffusion Coefficients of Selected Substances in Water at Infinite Dilution at 25°C
- APPENDIX H Lennard–Jones Potential Model Constants for Selected Molecules
- APPENDIX I Collision Integrates for the Lennard–Jones Potential Model
- APPENDIX J Physical Constants
- APPENDIX K Unit Conversions
- References
- Index
- References
Summary
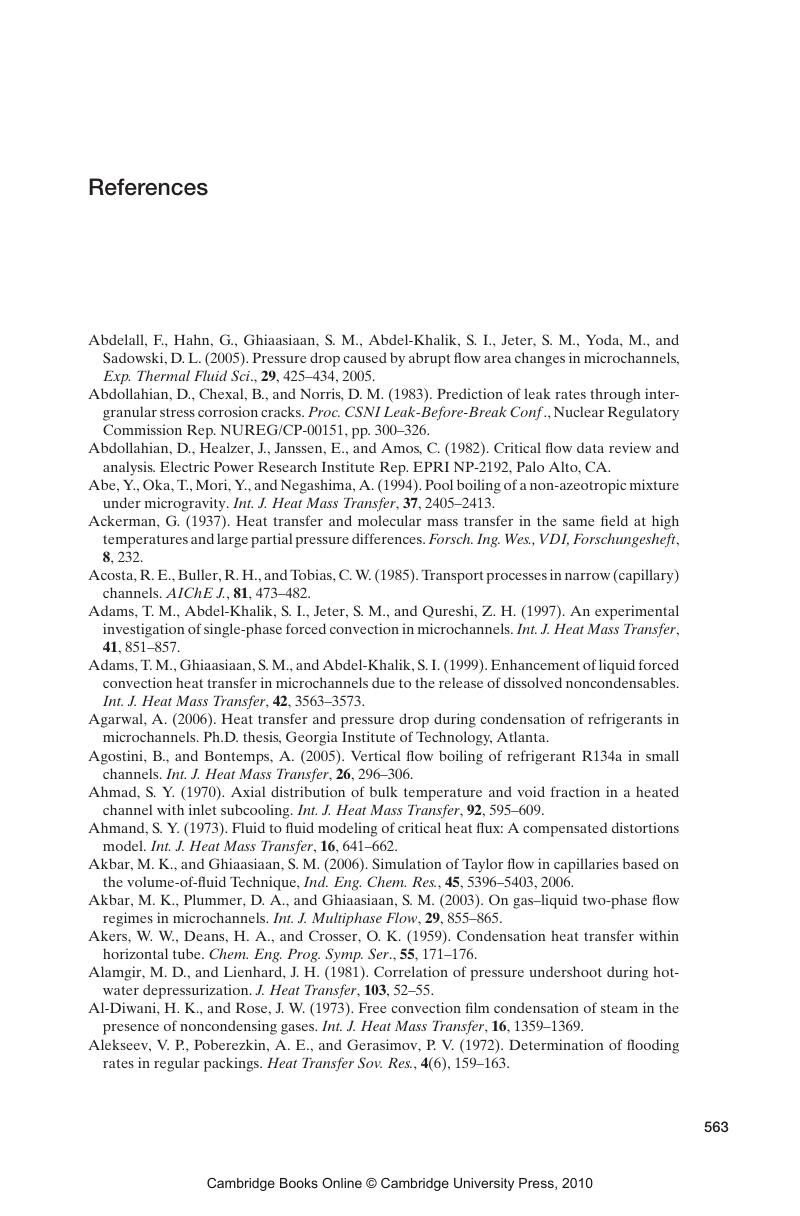
- Type
- Chapter
- Information
- Two-Phase Flow, Boiling, and CondensationIn Conventional and Miniature Systems, pp. 563 - 600Publisher: Cambridge University PressPrint publication year: 2007