Book contents
- Frontmatter
- Contents
- Preface
- Acknowledgements
- Chapter 1 Global transitions in proteins
- Chapter 2 Molecular forces in biological structures
- Chapter 3 Conformations of macromolecules
- Chapter 4 Molecular associations
- Chapter 5 Allosteric interactions
- Chapter 6 Diffusion and Brownian motion
- Chapter 7 Fundamental rate processes
- Chapter 8 Association kinetics
- Chapter 9 Multi-state kinetics
- Chapter 10 Enzyme catalysis
- Chapter 11 Ions and counterions
- Chapter 12 Fluctuations
- Chapter 13 Ion permeation and membrane potential
- Chapter 14 Ion permeation and channel structure
- Chapter 15 Cable theory
- Chapter 16 Action potentials
- Appendix 1 Expansions and series
- Appendix 2 Matrix algebra
- Appendix 3 Fourier analysis
- Appendix 4 Gaussian integrals
- Appendix 5 Hyperbolic functions
- Appendix 6 Polar and spherical coordinates
- References
- Index
- References
References
Published online by Cambridge University Press: 24 May 2010
- Frontmatter
- Contents
- Preface
- Acknowledgements
- Chapter 1 Global transitions in proteins
- Chapter 2 Molecular forces in biological structures
- Chapter 3 Conformations of macromolecules
- Chapter 4 Molecular associations
- Chapter 5 Allosteric interactions
- Chapter 6 Diffusion and Brownian motion
- Chapter 7 Fundamental rate processes
- Chapter 8 Association kinetics
- Chapter 9 Multi-state kinetics
- Chapter 10 Enzyme catalysis
- Chapter 11 Ions and counterions
- Chapter 12 Fluctuations
- Chapter 13 Ion permeation and membrane potential
- Chapter 14 Ion permeation and channel structure
- Chapter 15 Cable theory
- Chapter 16 Action potentials
- Appendix 1 Expansions and series
- Appendix 2 Matrix algebra
- Appendix 3 Fourier analysis
- Appendix 4 Gaussian integrals
- Appendix 5 Hyperbolic functions
- Appendix 6 Polar and spherical coordinates
- References
- Index
- References
Summary
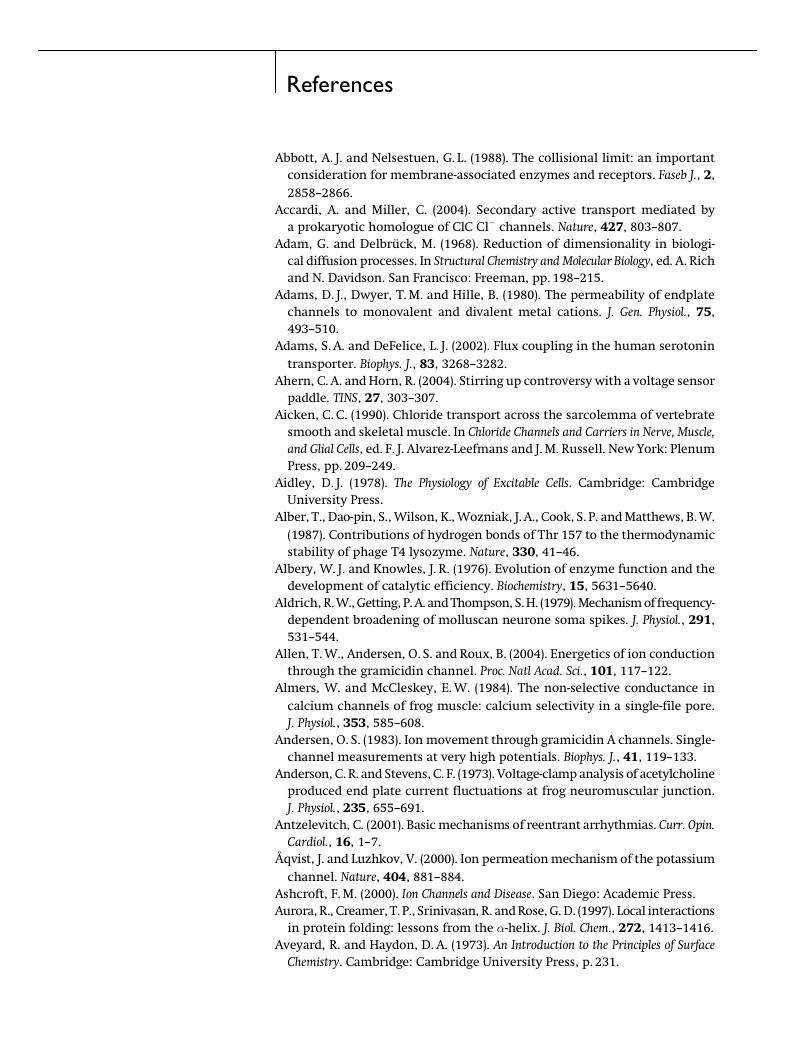
- Type
- Chapter
- Information
- Molecular and Cellular Biophysics , pp. 486 - 503Publisher: Cambridge University PressPrint publication year: 2006