Book contents
- Frontmatter
- Contents
- Preface
- Contributors
- 1 CNS oligarchs; the rise of the oligodendrocyte in a neuron-centric culture
- 2 Comparative biology of Schwann cells and oligodendrocytes
- 3 Control of oligodendrocyte development and myelination in the vertebrate CNS
- 4 Molecular organization of the oligodendrocyte and myelin
- 5 The genetics of oligodendrocytes
- 6 Immunobiology of the oligodendrocyte
- 7 Oligodendrocytes and disease: repair, remyelination and stem cells
- 8 Glial progenitor cells and the dynamics of the oligodendrocyte and its myelin in the aged and injured CNS
- 9 Oligodendroglial pathology in multiple sclerosis
- 10 Glutamate receptors, transporters and periventricular leukomalacia
- References
- Index
- Plate section
- References
References
Published online by Cambridge University Press: 05 August 2012
- Frontmatter
- Contents
- Preface
- Contributors
- 1 CNS oligarchs; the rise of the oligodendrocyte in a neuron-centric culture
- 2 Comparative biology of Schwann cells and oligodendrocytes
- 3 Control of oligodendrocyte development and myelination in the vertebrate CNS
- 4 Molecular organization of the oligodendrocyte and myelin
- 5 The genetics of oligodendrocytes
- 6 Immunobiology of the oligodendrocyte
- 7 Oligodendrocytes and disease: repair, remyelination and stem cells
- 8 Glial progenitor cells and the dynamics of the oligodendrocyte and its myelin in the aged and injured CNS
- 9 Oligodendroglial pathology in multiple sclerosis
- 10 Glutamate receptors, transporters and periventricular leukomalacia
- References
- Index
- Plate section
- References
Summary
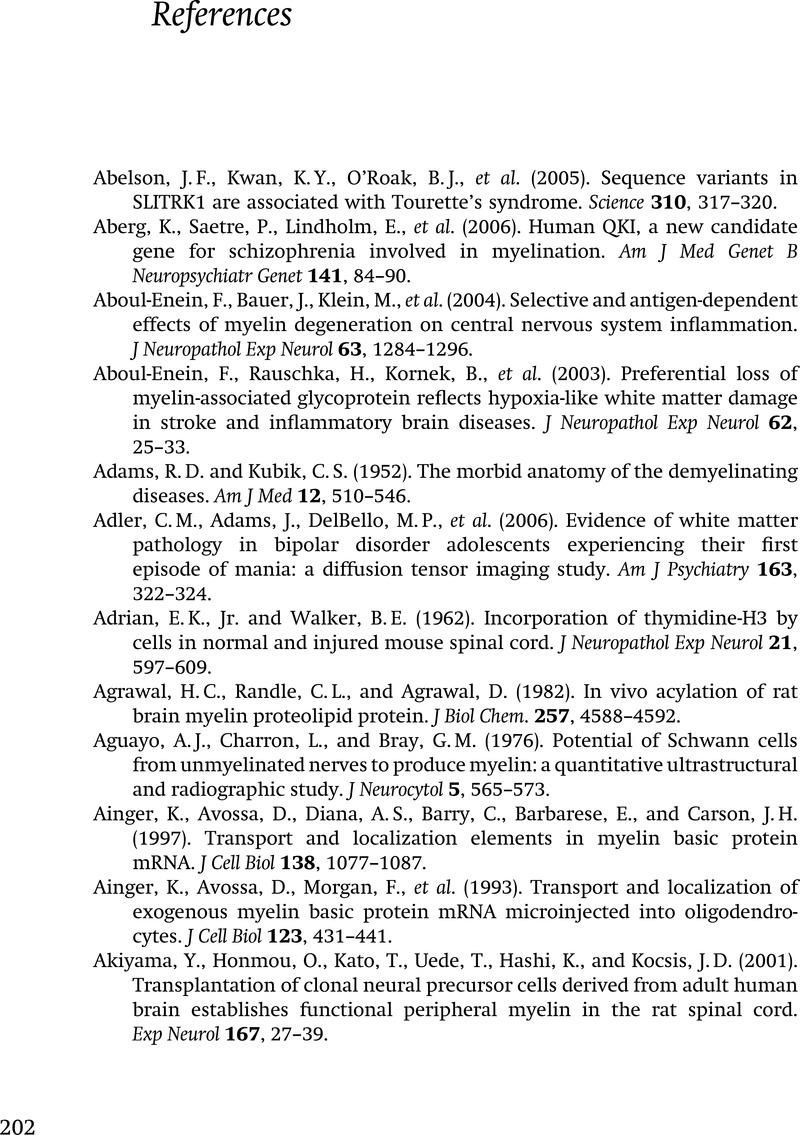
- Type
- Chapter
- Information
- The Biology of Oligodendrocytes , pp. 202 - 277Publisher: Cambridge University PressPrint publication year: 2010