Book contents
- Frontmatter
- Contents
- Preface
- 1 Introduction
- 2 Clouds and Their Properties
- 3 Thermodynamic Relations
- 4 Properties of Water and Aqueous Solutions
- 5 Diffusion and Coagulation Growth of Drops and Crystals
- 6 Wet Aerosol Processes
- 7 Activation of Cloud Condensation Nuclei into Cloud Drops
- 8 Homogeneous Nucleation
- 9 Heterogeneous Nucleation of Drops and Ice Crystals
- 10 Parameterizations of Heterogeneous Ice Nucleation
- 11. Deliquescence and Efflorescence in Atmospheric Aerosols
- 12 Terminal Velocities of Drops and Crystals
- 13 Broad Size Spectra in Clouds and the Theory of Stochastic Condensation
- 14 Analytical Solutions to the Stochastic Kinetic Equation for Precipitating Clouds
- References
- Notations
- Index
- References
References
Published online by Cambridge University Press: 05 September 2014
- Frontmatter
- Contents
- Preface
- 1 Introduction
- 2 Clouds and Their Properties
- 3 Thermodynamic Relations
- 4 Properties of Water and Aqueous Solutions
- 5 Diffusion and Coagulation Growth of Drops and Crystals
- 6 Wet Aerosol Processes
- 7 Activation of Cloud Condensation Nuclei into Cloud Drops
- 8 Homogeneous Nucleation
- 9 Heterogeneous Nucleation of Drops and Ice Crystals
- 10 Parameterizations of Heterogeneous Ice Nucleation
- 11. Deliquescence and Efflorescence in Atmospheric Aerosols
- 12 Terminal Velocities of Drops and Crystals
- 13 Broad Size Spectra in Clouds and the Theory of Stochastic Condensation
- 14 Analytical Solutions to the Stochastic Kinetic Equation for Precipitating Clouds
- References
- Notations
- Index
- References
Summary
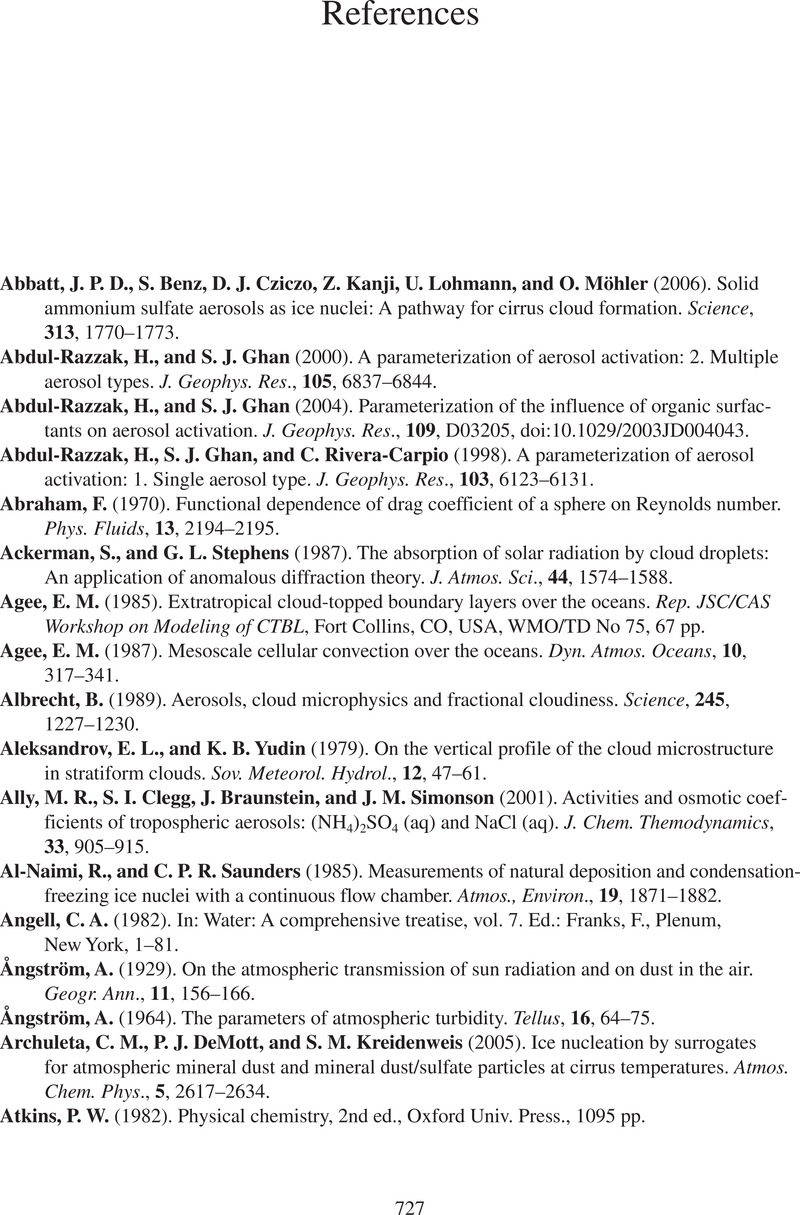
- Type
- Chapter
- Information
- Thermodynamics, Kinetics, and Microphysics of Clouds , pp. 727 - 766Publisher: Cambridge University PressPrint publication year: 2014