Book contents
- No Miracles Needed
- Reviews
- No Miracles Needed
- Copyright page
- Dedication
- Contents
- Figures
- Foreword
- Preface
- 1 What Problems Are We Trying to Solve?
- 2 WWS Solutions for Electricity Generation
- 3 WWS Solutions for Electricity Storage
- 4 WWS Solutions for Transportation
- 5 WWS Solutions for Buildings
- 6 WWS Solutions for Industry
- 7 Solutions for Nonenergy Emissions
- 8 What Doesn’t Work
- 9 Electricity Grids
- 10 Photovoltaics and Solar Radiation
- 11 Onshore and Offshore Wind Energy
- 12 Steps in Developing 100 Percent WWS Roadmaps
- 13 Keeping the Grid Stable with 100 Percent WWS
- 14 Timeline and Policies Needed to Transition
- 15 My Journey
- References
- Index
- References
References
Published online by Cambridge University Press: 02 February 2023
- No Miracles Needed
- Reviews
- No Miracles Needed
- Copyright page
- Dedication
- Contents
- Figures
- Foreword
- Preface
- 1 What Problems Are We Trying to Solve?
- 2 WWS Solutions for Electricity Generation
- 3 WWS Solutions for Electricity Storage
- 4 WWS Solutions for Transportation
- 5 WWS Solutions for Buildings
- 6 WWS Solutions for Industry
- 7 Solutions for Nonenergy Emissions
- 8 What Doesn’t Work
- 9 Electricity Grids
- 10 Photovoltaics and Solar Radiation
- 11 Onshore and Offshore Wind Energy
- 12 Steps in Developing 100 Percent WWS Roadmaps
- 13 Keeping the Grid Stable with 100 Percent WWS
- 14 Timeline and Policies Needed to Transition
- 15 My Journey
- References
- Index
- References
Summary
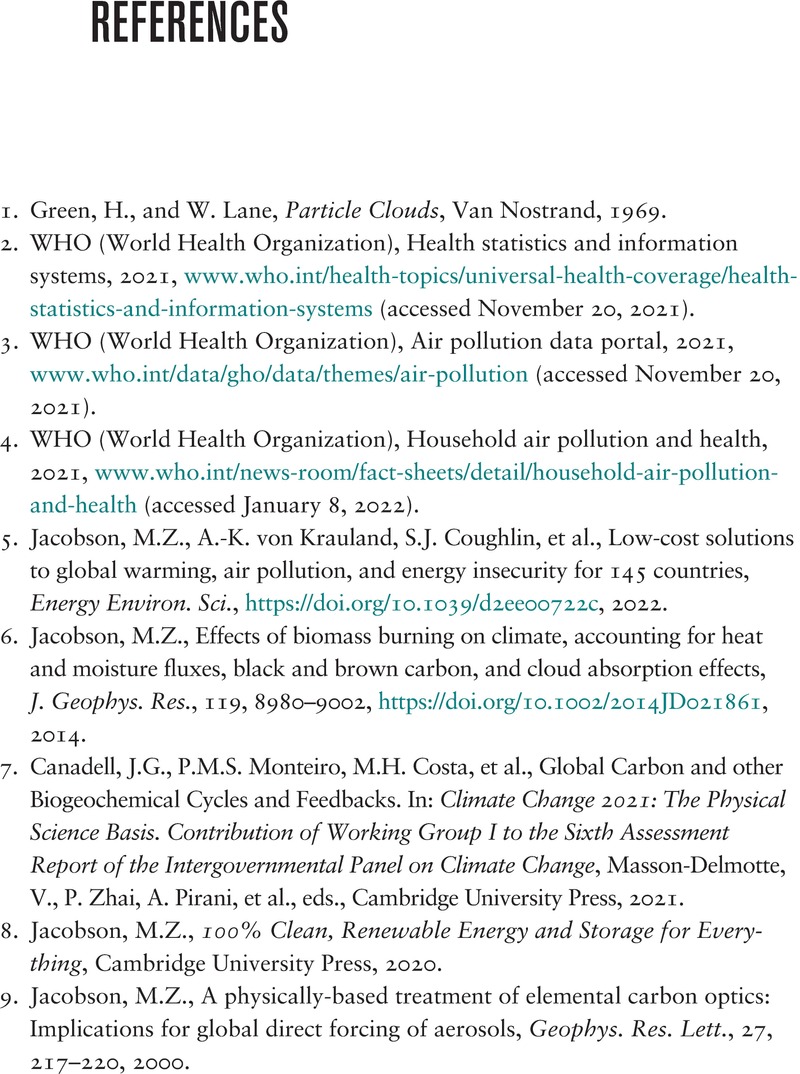
- Type
- Chapter
- Information
- No Miracles NeededHow Today's Technology Can Save Our Climate and Clean Our Air, pp. 384 - 412Publisher: Cambridge University PressPrint publication year: 2023