References
Published online by Cambridge University Press: 05 June 2012
Summary
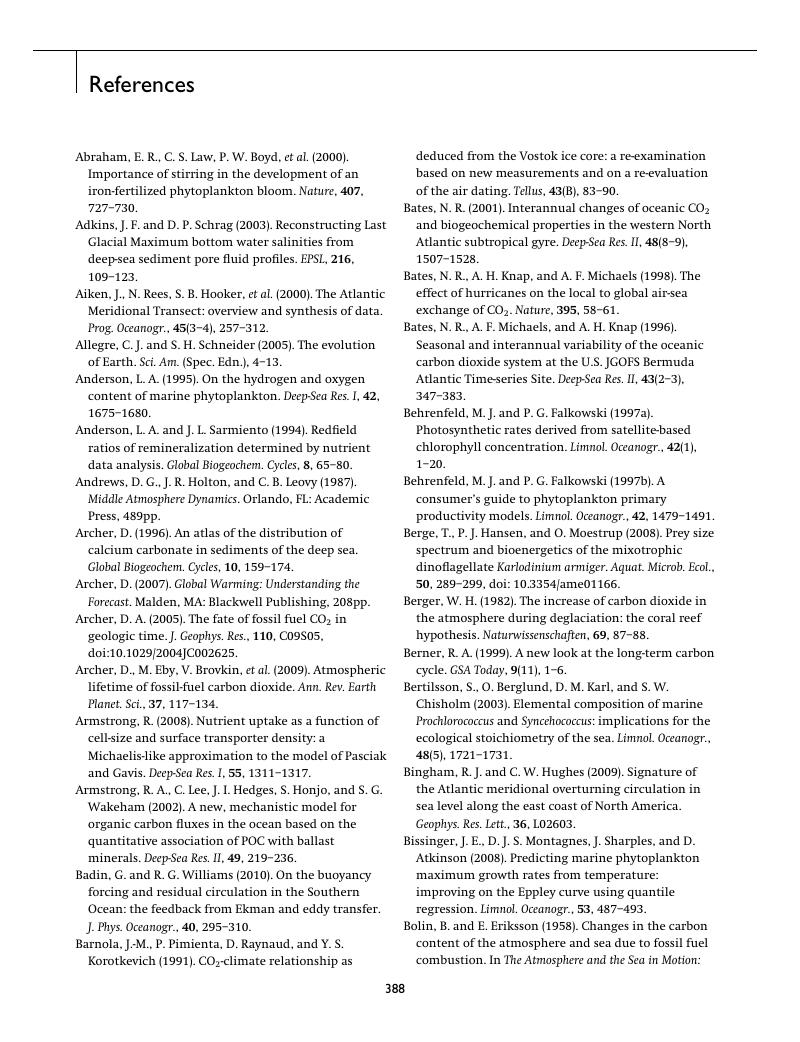
- Type
- Chapter
- Information
- Ocean Dynamics and the Carbon CyclePrinciples and Mechanisms, pp. 388 - 399Publisher: Cambridge University PressPrint publication year: 2011