Part III - Complex Mixing Consequences
Published online by Cambridge University Press: 05 June 2016
Summary
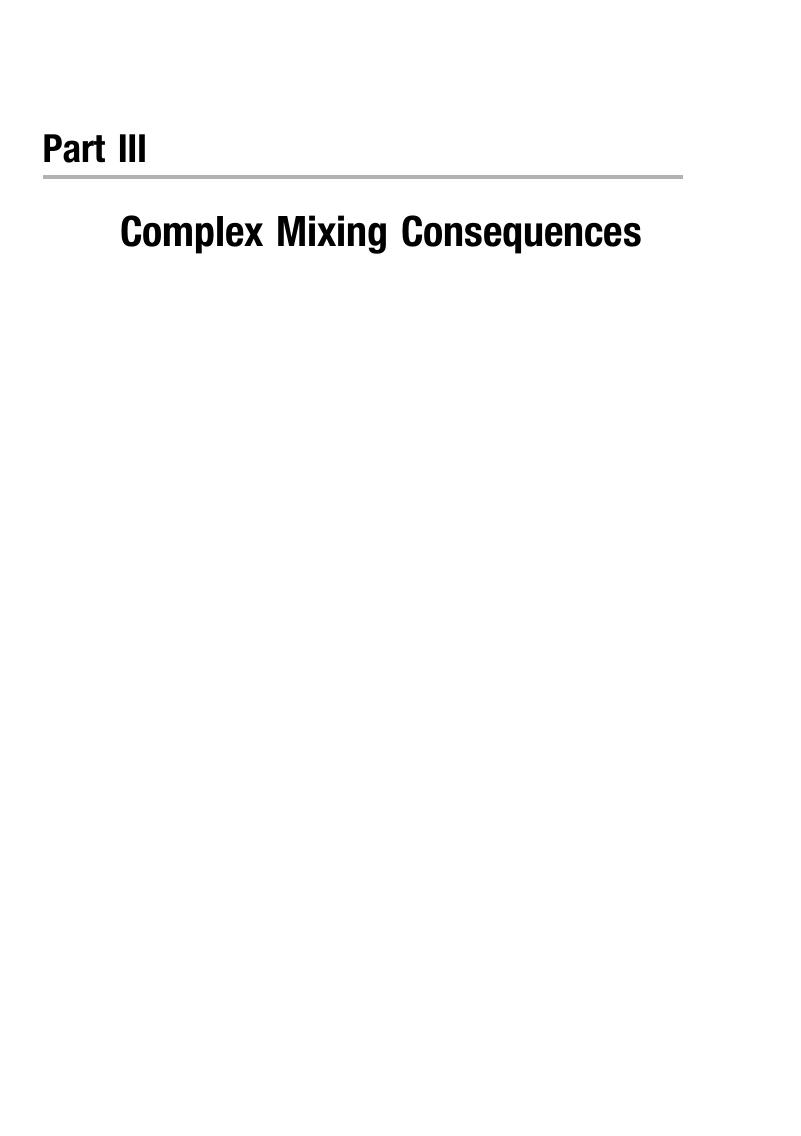
- Type
- Chapter
- Information
- Coarse Grained Simulation and Turbulent Mixing , pp. 191 - 442Publisher: Cambridge University PressPrint publication year: 2016