Book contents
- Frontmatter
- Contents
- Preface
- 1 Preliminaries
- 2 Dynamics of Single-Degree-of-Freedom Linear Systems
- 3 Dynamics of Multi-Degree-of-Freedom Linear Systems
- 4 Finite Element Method
- 5 Stochastic Processes
- 6 Variance Spectrum
- 7 Environmental Loads
- 8 Random Environmental Processes
- 9 Response Spectrum
- 10 Response Statistics
- 11 Statistics for Nonlinear Problems
- 12 Short-Term and Long-Term Extremes
- 13 Dynamic Load Effects for Design Checks
- 14 Equations of Motion
- 15 Numerical Solution Techniques
- 16 Monte Carlo Methods and Extreme Value Estimation
- A Integrals
- B Poisson Process
- C Statistical Moments and Cumulants
- References
- Index
- References
References
Published online by Cambridge University Press: 05 February 2013
- Frontmatter
- Contents
- Preface
- 1 Preliminaries
- 2 Dynamics of Single-Degree-of-Freedom Linear Systems
- 3 Dynamics of Multi-Degree-of-Freedom Linear Systems
- 4 Finite Element Method
- 5 Stochastic Processes
- 6 Variance Spectrum
- 7 Environmental Loads
- 8 Random Environmental Processes
- 9 Response Spectrum
- 10 Response Statistics
- 11 Statistics for Nonlinear Problems
- 12 Short-Term and Long-Term Extremes
- 13 Dynamic Load Effects for Design Checks
- 14 Equations of Motion
- 15 Numerical Solution Techniques
- 16 Monte Carlo Methods and Extreme Value Estimation
- A Integrals
- B Poisson Process
- C Statistical Moments and Cumulants
- References
- Index
- References
Summary
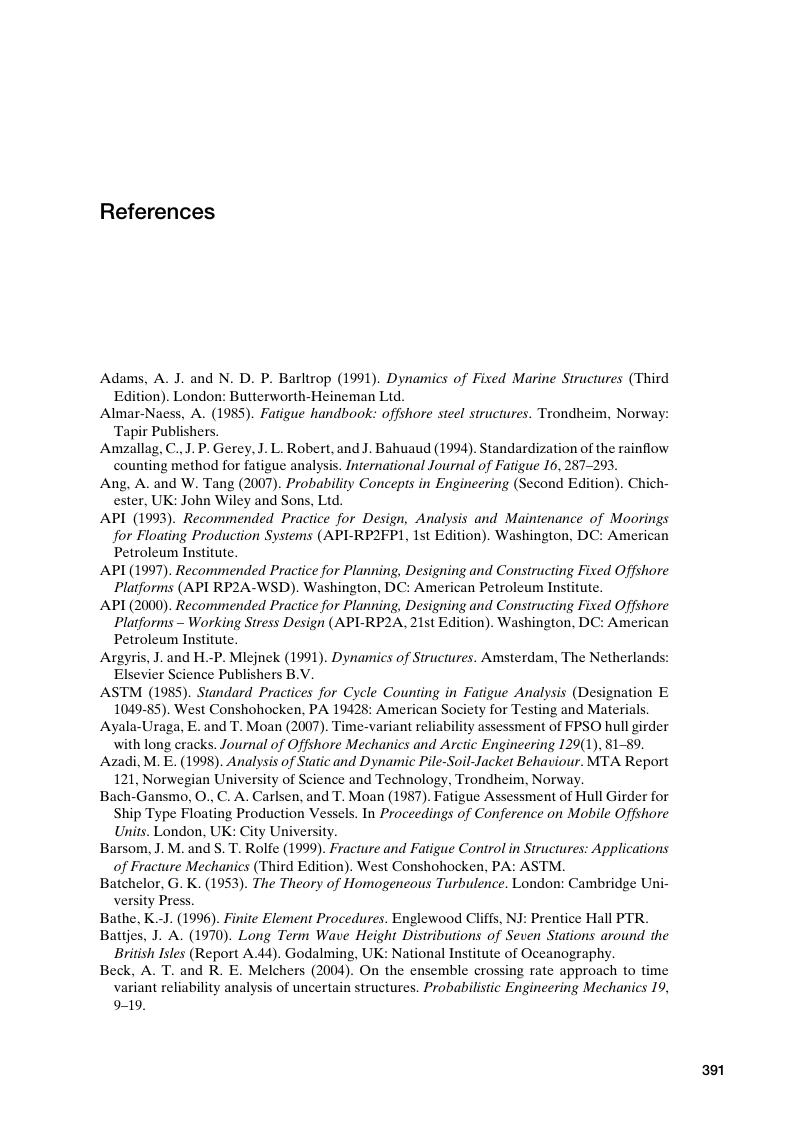
- Type
- Chapter
- Information
- Stochastic Dynamics of Marine Structures , pp. 391 - 404Publisher: Cambridge University PressPrint publication year: 2012