3 - Equilibrium large-scale conformational properties of DNA
Published online by Cambridge University Press: 05 March 2015
Summary
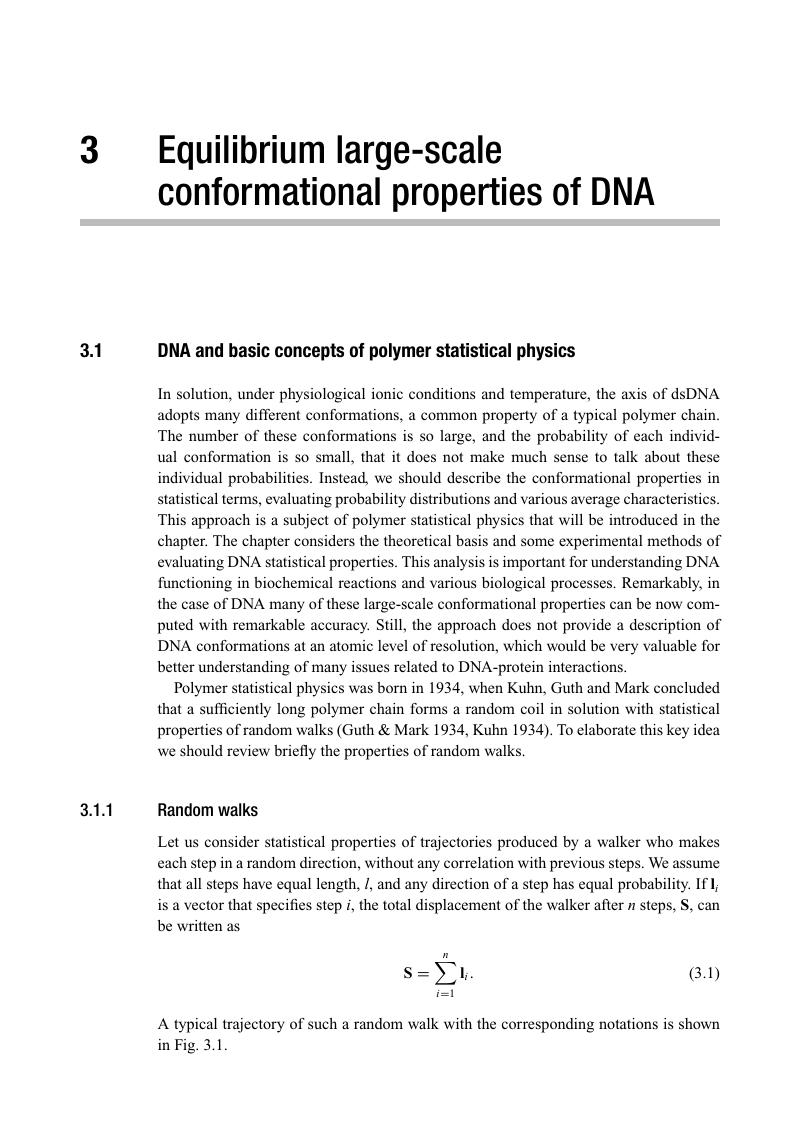
- Type
- Chapter
- Information
- Biophysics of DNA , pp. 72 - 136Publisher: Cambridge University PressPrint publication year: 2015
References
- 1
- Cited by